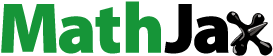
ABSTRACT
In this work, NaOH-activated Aerva javanica leaf (NAJL) powder was successfully developed as a cost-effective adsorbent for removal of crystal violet (CV) from an aqueous environment. The prepared adsorbent was characterized using FTIR, SEM, and their surface area was determined by BET technique. Different parameters affecting the adsorption of CV dye such as pH solution, adsorbent dose, equilibrium time, initial CV concentration, and temperature were investigated. The results revealed that the best-optimized values were found as pH–9, dose–20 mg, time–30 min, temperature– 25 °C. The adsorption experimental data exhibited excellently fitted well to the nonlinear Langmuir isotherm and Pseudo-first-order kinetic models. The maximum monolayer adsorption capacity of CV dye was 315.2 mg/g. The experimental parameters of thermodynamic analysis exhibited that the CV dye adsorption onto NAJL powder was exothermic and spontaneous. Electrostatic attraction, π-π interactions, and Hydrogen bonding were the mechanism behind the adsorption of CV dye.
1. Introduction
Nowadays, organic dyes are one of the main sources of water pollution due to their wide application in industry such as for dyeing papers, textile fibers, plastics, leathers and pharmaceuticals [Citation1]. According to a recent report, about 10 thousand various types are produced with a rate of more than 700 thousand tons/year, and most of the dyes available in the market [Citation2]. Dyes such as malachite green (MG), methylene blue (MB) and crystal violet (CV) have a negative effect on water quality, and it is carcinogenic dyes that can potentially affect human as well as animal life [Citation3–5]. Hence, the removal of toxic dyes poses challenges to the researchers in finding excellent methods for the elimination of dyes before their release into the aqueous environment. Numerous techniques of treating dye effluents including electrochemical [Citation6] ion exchange [Citation7] membranes [Citation8], ozonation [Citation9], degeneration [Citation10,Citation11] and adsorption [Citation12–14]. Among them, adsorption is a simple method widely used in water treatment due to its low cost, effectiveness and easy regeneration [Citation15,Citation16]. Elimination of dyes from wastewater can be accomplished by various adsorbents that were used in adsorption techniques like TLAC/Chitosan [Citation17], bentonite-alginate composite [Citation18], cellulose-based adsorbent (CGS) [Citation19], coffee husks [Citation20], polypyrrole-based KOH-activated carbon [Citation21], acid-modified eucalyptus leaves [Citation22]. Commercially activated carbon is applied for dye adsorption from wastewater, but these adsorbents are highly expensive, limiting its commercial application [Citation23–25]. The suitable adsorbent can be described by low cost, high removal capacity and high renewability [Citation26].
Recently, the adsorbents based on agricultural material or biosorption (biomass) have been a special interest due to its offer several benefits; they are cost-effective, non-toxic, biodegradable, and it has in its structure many functional groups that can form bonds with dyes. Some of the bio adsorbents that have been applied to eliminate pollutants include Moringa oleifera [Citation27], Senna alata [Citation28], Gmelina leaves [Citation29], Imperata cylindrica [Citation30], Walnut shell [Citation31], Artocarpus camansi Blanco core [Citation32], Casuarina equisetifolia needle [Citation33], Pine leaves [Citation34], Pinus nigra [Citation35], etc. Therefore, the utilization of abundantly available biomass from plants is a feedstock for the production of excellent adsorbents having a wide range of energy and environmental applications.
Aerva javanica is a herb and widely dispersed in countries of the world. It is found in some Asian countries like Saudi-Arabia (Western-Southern parts) and the native of Aerva javanica is Africa [Citation36]. Aerva javanica is a herb medicinal plant and has various medicinal benefits. Almost all parts of Aerva javanica exhibit antioxidant, antiviral and antidiabetic activities [Citation37]. Anand et al. conducted a study to examine the components of Aerva javanica and the results revealed that the presence of flavonoids, alkaloids, phenols, glycosides, tannins, saponins and a minute amount of terpenoids [Citation38].
The objective of this study was for activation of the Aerva javanica leaves and inflorescence using H2O2 and NaOH reagent. Then, the untreated Aerva javanica leaves & inflorescence and NaOH-activated Aerva javanica leaves and inflorescence adsorbents were used for the elimination of CV dye ions from the aqueous solution. The NAJL powder were characterized using SEM, FTIR and BET techniques. The effect of pH, contact time, initial CV concentration, adsorbent dose and temperature on the adsorption process was achieved. The nonlinear three isotherms and kinetic adsorption were studied to quantify the adsorption equilibrium and mechanism adsorption. Further, the spontaneousness and nature of the adsorption process were described via thermodynamic study.
2. Experiment
2.1. Chemicals and instrumentation
The chemicals used in this study were analytical reagents (AR). Rhodamine B (RB), crystal violet (CV) and methylene blue (MB) dyes were procured from Sigma Aldrich. HNO3, HCl and NaOH were obtained from BDH, England. Deionized water was used in this work. The adsorbent material was characterized by different techniques such as scanning electron microscopy (Model JEM-2100 F, JEOL, Japan), Fourier transform infrared spectroscopy (Nicolet iS50 FTIR, Thermo Scientific), BET surface area and pore diameter analyzer Micromeritics (Gemini VII, 2390, USA). The CV, MB and RB dye concentrations in the solution were determined using UV–Vis spectrophotometer (Shimadzu UV-1800, 900 T).
2.2. Chemical treatment
For the present study, Aerva javanica leaves (AJL) and Aerva javanica inflorescence (AJF) were collected from Albaha City in Saudi Arabia. AJL and inflorescence materials were washed several times with deionized water to eliminate any dirt and other contaminants followed by dried at 100°C on the oven for 24 h. Then, the dried AJL and AJF were crushed separately in a grinder to get a fine powder. After that, 50 g of leaf powder was taken in a beaker containing 200 mL of 10% H2O2 under stirring for 24 h at room temperature [Citation39] [Citation40]. The powder was washed with deionized water followed by separation using centrifuge. The AJL powder was immersed in a 200 mL 1 M NaOH solution. The mixture solution was stirred at room temperature for 24 h and washed off with deionized water and dried at room temperature for 72 h to get NaOH-activated Aerva javanica leaf (NAJL) powder adsorbent. The same procedure was applied for activation of the Aerva javanica inflorescence (AJF) to produce NaOH-activated Aerva javanica inflorescence (NAJF) powder adsorbent. The preparation of NAJL powder adsorbent is shown in .
2.3. Adsorption studies
To test the adsorption efficiencies of CV, MB and RB dyes onto four adsorbents: untreated AJL and untreated AJF, NAJL and NAJF powder adsorbent, the batch adsorption was applied. CV dye adsorption experiment was executed under different temperature (25, 35 and 45°C), pH solution (3–11), adsorbent dose (1–100 mg), contact time (5–120 min), initial CV dye concentration (50–250 mg/L). In general, a definite amount of adsorbents was added to the conical flask containing 50 mL of CV dye solution with a certain initial concentration at the preferred pH value and temperature. The initial pH solution was adjusted by 0.1 M NaOH and 0.1 M HCl solution. The mixture was shaken by a shaker at 200 rpm. The residual CV, MB and RB dye ions concentrations were determined by UV/Visible spectrometer at λmax (590 nm), (663 nm) and (556 nm), respectively. The adsorption efficiency (%) of CV, MB and RB dye ions onto adsorbents and adsorption capacities (mg/g) of adsorbents are calculated according to EquationEquation (1)(1)
(1) and EquationEquation (2)
(2)
(2) , respectively.
where Co (mg/L) is the initial CV dye concentration; Ce (mg/L) is the CV dye concentration at equilibrium; m (g) is the amount of adsorbent and V (L) is the solution volume of the CV, MB and RB dyes.
3. Results and discussion
3.1. Characterization of adsorbents
shows the FTIR spectrum for AJL and NAJL, and CV dye loaded on NAJL. The complex nature of AJL powder results in the existence of some absorption peaks. In the spectrum of untreated AJL powder ()), the broad peak at 3325 cm−1 assigned to the stretching vibration of O-H groups suggesting the presence of carboxylic and hydroxyl groups on the surface of AJL powder [Citation41]. The peaks at 2922 and 2855 cm−1 were attributed to asymmetric and symmetric vibration of aliphatic C–H, respectively. The peak at 1731 cm−1 was assigned to carbonyl stretching in AJL structure [Citation42,Citation43]. The strong peak at 1620 cm−1 was described as C = O stretching from the carboxylic group and also for aromatic bending of the alkene group (C = C) [Citation44,Citation45]. The peaks at 1310–1370 cm−1 and 1237 cm−1 were assigned to the symmetric stretching vibration of deprotonated carboxyl group (-COO−) and (C-O-C) respectively. All these functional groups present in the structure of AJL powder are attributed to some compounds, such as lignin, phenol, alkaloids, flavonoids, glycosides and tannins [Citation38,Citation46]. After chemical activation of AJL powder with H2O2 and NaOH ()), the intensities of –OH, -COO−/C = C and C-O-C peaks were increased. As we are known that the peak area commonly reflects mole percentage of the corresponding functional group and FTIR can be used for such qualitative analysis with acceptable accuracy when a known unaffectable peak was used as an internal standard. Therefore, the peak areas of the carboxylate bands from both AJL and NAJL powder were calculated. In this regard, it is assumed that the peak corresponding to CH, CH2 and CH3 (sp3 C-hybridization) in the range 3000–2800 cm−1 were unaffected during the application of H2O2 and thus can be used as an internal standard. By comparing the peak areas of –COO− with respect to CHx in both, we found that COO/CHx is 11.7 and 9.5 in both NAJL and AJL, respectively indicating –COO− mole fraction increase after treatment. The disappearance of the COOH peak at 1731 cm−1 is possibly due to transformation into carboxylate which causes a slight shift into lower frequency and thus overlapped with other carbonyl, carboxyl derivatives, etc., of the peak centered at 1620 cm−1 [Citation47].
Figure 2. FT-IR spectra of untreated AJL and NAJL powder (a), NAJL powder and CV dye adsorption onto NAJL powder (b)

A new peak at 1422 cm−1 attributed to stretching of –OH bonds in carboxyl groups may be due to increasing the carboxylic groups in the NAJL surface [Citation48]. These results confirmed the activation of AJL powder with H2O2 and NaOH. After the CV dye adsorption ()), some bands disappeared, decreased in intensity and shifted to lower frequency. The bands at 3325 cm−1 and 1620 cm−1 were reduced intensity and moved to 3312 cm−1 and 1598 cm−1, respectively which confirmed the binding of these bands with CV dye ions over the NAJL surface. Therefore, the results indicated that a large number of -OH, -COOH, C = O groups were available on the surface NAJL powder [Citation49].
The morphology of AJL, NAJL powder, and CV dye loaded on NAJL powder are shown in ). The AJL powder exhibits an irregular rough surface with fewer pores with some impurities ()). After being activated with NaOH reagent, the surface of AJL powder was smooth, pores, visible cavities with different shapes and sizes due to the removal of impurities from the surface of the AJL powder adsorbents by activation agent (NaOH) ()). Thus, the NAJL powder adsorbent with these characteristics generally has a good surface, which provides them a good removal efficiency. After CV dye adsorption onto the NAJL powder, the surface of adsorbents appeared to be fewer pores and covered with CV dye ions ()), indicating successful loading of CV dye on the NAJL powder surface.
displays the N2 adsorption-desorption isotherms and pore diameter distribution of the AJL and NAJL powder. The pore-volume, pore diameter and surface area of the AJL and NAJL powder are shown in . The results revealed that the BET surface area of NAJL powder was 10.65 m2/g which is greater than untreated AJL powder 6.20 m2/g ( due to the pore structure being formed after activating with NaOH agent which led to the increased surface area of NAJL powder. The pore diameter of AJL and NAJL powder was 10.2 nm and 18.6 nm (, respectively indicating the mesoporous structure of adsorbents.
Table 1. Pore structure parameter for AJL and NAJL powder adsorbent
3.2. Adsorption studies
3.2.1. Selectivity studies of adsorbent
The selective adsorption of cationic dyes MB, RB and CV dyes from aqueous environments was tested on four different adsorbents: untreated AJL, AJF, NAJL and NAJF powder (). The results showed that the NAJL powder exhibited selective and higher removal efficiencies towards CV dye ions than that is untreated AJL, AJF and NAJF powder. The highest CV dye removal efficiency of 97.49% was achieved by NAIL powder at the conditions of the parameters (dose – 50 mg, T – 25°C, Co– 50 mg/g). The removal efficiencies of MB and RB were 71.26% and 3.0% by NAIL powder at the same conditions, respectively. The decrease in adsorption efficiency of MB and RB dyes onto NAJL adsorbent may be due to the MB dye has a positive charge on its S atom due to its resonance form. This positive charge of S atom binds to the negative carboxyl and hydroxyl groups on the NAJL surface. Therefore, MB molecules occupy larger areas on the NAJL surface, hindering the binding of additional MB molecules, leading to a decrease in adsorption efficiency [Citation50]. RB has a carboxylic group in its structure which has an electrostatic effect with carboxyl groups on NAJL surface, and also it has a larger steric hindrance that affects its ability to be absorbed on the NAJL surface, leading to a decrease in adsorption efficiency. The high removal efficiency of CV dye on NAIL powder may be due to the use of H2O2 and NaOH as an activation agent. The NaOH reacts with silica in AJL powder adsorbent to produce a soluble sodium silicate in water and is eliminated by the washing process. Thus, the pore structure is formed which led to the increased surface area of the adsorbent [Citation51,Citation52]. The NAJL powder adsorbent was selected for CV dye removal from an aqueous solution.
Table 2. Selectivity studies of cationic dyes onto the adsorbents
3.2.2. Effect of pH
As we know, the adsorption process is strongly dependent on pH value because the pH is the effect on the surface charge of adsorbent and ionic state of adsorbent. Therefore, the effect of pH solution on CV dye adsorption on NAJL powder was tested in the range (3 − 11) as shown in ). It was observed that the CV dye removal efficiency and adsorption capacity were increased from (39.2% to 79.8%) and (49 to 99.71 mg/g) with rising pH values from 3 to 9 due to increasing the negative charge of NAJL powder adsorbent with rising pH value. With a further increase in pH from 8 to 9, no change in CV dye removal efficiency was noticed. A similar tendency of pH effect was noticed for the CV adsorption on NaOH-modified rice husk [Citation53]. In an acidic medium, the adsorption capacity of CV dye was low due to the protonation of adsorbent’s active sites [Citation54]. With rising pH of the solution, the adsorption process was increased significantly to 99.71 mg/g at pH 9 due to deprotonation of active sites of NAJL powder adsorbent, and the electrostatic attraction CV dye is attracted to these negatively charged groups on the surface of NAJL powder adsorbent [Citation55]. Thus, pH 9 was selected for further experiments.
3.2.3. Effect of adsorbent dose
The influence of NAJL powder adsorbent dose on CV dye elimination was studied under various adsorbent doses from 1 to 100 mg to obtain the best appropriate amount of adsorbents at condition parameters (Co– 50 mg/L; time – 30 min; T – 25°C), as shown in ). The result displayed that as the amount of NAJL powder adsorbent increased from 1 to 20 mg the removal efficiency of CV dye improved from 54.1% to 98.9% due to an increase in the active site number adsorbent. On the other hand, the adsorption capacity of NAJL powder adsorbent decreased from 195.35 to 3.57 mg/g with an increase of the amount of adsorbent from 1 to 100 mg. At lower adsorbent amount, the CV dye is more easily accessible to NAJL surface; thus, the removal of CV dye per unit weight of adsorbent is higher. With the rise in the mass of NAJL adsorbent, some of the adsorption sites present on the surface of NAJL remaining unoccupied during the adsorption process due to the fact that, at higher adsorbent mass, there is a very fast superficial adsorption onto the NAJL surface that produces a lower CV dye concentration in the solution than when the adsorbent dose is lower [Citation56]. Therefore, with rising adsorbent amounts from 1 to 100 mg, the quantity of CV dye adsorbed per unit mass of adsorbent is decreased [Citation57]. Also, the reduction in adsorption capacity may be due to a decline in the total surface area and a rise in diffusion path length resulting from aggregation or overlapping of adsorption active sites of adsorbent, leading to a reduction in adsorption. These results are in agreement with the findings of literature reported in [Citation58,Citation59]. Thus, the optimum adsorbent dose for removal of CV dye onto NAJL powder adsorbent was 20 mg.
3.2.4. Effect of contact time at various concentrations
The influence of contact time on the elimination of CV dye at various initial concentrations 75 and 100 mg/L onto NAJL powder adsorbent at condition parameters (T–25°C; dose–20 mg; pH–9) are presented in ). The results revealed that, at all initial CV dye concentrations, the adsorption capacity of NAJL powder adsorbent for CV dye was rapidly increased from 99.39 to 132.09 mg/g at 75 mg/L and from 120.54 to 177.83 mg/g at 100 mg/L with increasing time from 5 to 30 min. Hence, 30 min was chosen as the best equilibrium time for further work. A similar tendency of contact time effect was noticed for the CV adsorption on grapefruit peel (GFP) [Citation60].
3.2.5. Effect of initial concentration at various temperatures
) displays the influence of initial CV dye concentration on the adsorption process at different concentrations (50 to 250 mg/L) and different temperatures (25, 35 and 45°C) under condition (dose – 20 mg; pH – 9; time – 30 min). The results exhibited that the adsorption capacity of NAJL powder adsorbent increased from 101.57 to 268.70 mg/g when the CV dye concentrations increased from 50 to 250 mg/L at 25°C. The high rise in adsorption capacity at higher initial concentrations, due to the increase of the driving force for mass transfer from the solution to the NAJL powder adsorbent surface [Citation61, Citation62].
3.2.6. Effect of temperatures
The influence temperature on CV dye adsorption onto NAJL powder adsorbent was performed at various temperatures (25, 35 and 45°C) and at different concentrations (50 to 250 mg/L) under condition (dose – 20 mg; pH–9; time–30 min). The obtained results are presented in . It was noticed that the adsorption capacity slightly reduced with rising temperature, indicating that the adsorption of CV dye was an exothermic process. This is due to the destruction of binding forces on the surface of NAJL powder adsorbent at higher temperatures [Citation40]. This result is in agreement with the study done by Shoukat et al. [Citation63].
3.2.7. Adsorption isotherm
CV dye adsorption onto NAJL powder adsorbent with different initial concentrations (50- 250 mg/L) and different temperature was studied with keeping other the other parameters constants (pH–9; dose– 20 mg; time–30 min; 200 rpm). The adsorption experimental data are investigated by Langmuir (EquationEquation (3(3)
(3) )) [Citation64], Freundlich (EquationEquation (4))
(4)
(4) [Citation65] and Dubinin Radushkevich nonlinear isotherm models (EquationEquations (5–7)
(5)
(5) ) [Citation66]. Isotherm experimental data for isotherm modeling is presented in .
Table 3. Nonlinear isotherm models for CV adsorption on NAJL powder adsorbent
where qe (mg/g) is the amount of CV dye adsorbed at equilibrium, qm (mg/g) is maximum adsorption capacity; Ce (mg/L) is the CV dye concentration at equilibrium; KL (L/mg) is Langmuir constant represents the adsorption affinity. KF and KD are Freundlich constant and constant related to the adsorption energy, respectively; n is the parameter that characterizes the system heterogeneity; E (kJ/mol) refers to mean free energy; ɛ is the Polanyi potential. Nonlinear curves of isotherms models at various temperatures (25, 35 and 45°C) are shown in . The results of the models employed are summarized in . Based on the high correlation coefficients (R2) of nonlinear isotherm models, the Langmuir model (R2 > 0.9843) is the best fit of experimental data than that’s Dubinin Radushkevich and Freundlich model. These results suggested that the adsorption of CV on NAJL powder adsorbent was a monolayer adsorption process. The maximum adsorption of CV on NAJL powder adsorbent was 315.2 mg/g at 25°C. The RL values at different temperatures (25–45°C) were in the range (0.044–0.026), indicating favorable adsorption. The comparison of parameter condition and adsorption capacities of NAJL powder adsorbent for CV dye removal with different adsorbents such as NaOH-modified rice husk (NMRH) [Citation53], grapefruit peel [Citation60], Eichhornia crassipes live roots [Citation67], jackfruit leaf powder (JLP) [Citation68], banyan leaf powder [Citation69], treated ginger waste (TGW) [Citation70] are shown in . Based on the results of the Dubinin–Radushkevich isotherm model, the free energy value (E) was less than 8 kJ mol−1 which suggests that the CV adsorption on NAJL powder adsorbent is physisorption.
Table 4. Comparison of the maximum adsorption capacities (qm) for CV adsorption with different plant adsorbents
3.2.8. Kinetics adsorption
Applicability of different nonlinear kinetic models such as Elovich (EquationEquation (8))(8)
(8) models [Citation71], pseudo-first-order (EquationEquation (9))
(9)
(9) [Citation72] and pseudo-second-order (EquationEquation (10))
(10)
(10) models was used to analyze the kinetic experimental data.
where, k1 and k2 are the equilibrium rate constant of pseudo-first-order adsorption and pseudo-first-order, respectively. ԛt is the adsorption capacity at t time (mg/g); α and β are the desorption constant and the initial adsorption rate, respectively.
Nonlinear curves of kinetic models at condition parameters (dose – 20 mg; pH–9; time – 30 min, Co – 75 mg/L) are presented in . The results of the models employed are listed in . It was observed that the pseudo-first-order model shows better R2 (R2 = 0.997) values compared to that’s pseudo-second-order (R2 = 0.976) and Elovich (R2 = 0.974), suggesting the involvement of the physical adsorption process. The experimental adsorption capacity (qe,exp = 132 mg/g) was very close to qe,cal 132.5 mg/g in pseudo-first-order model.
Table 5. Nonlinear kinetics model parameters for CV adsorption on NAJL powder adsorbent
3.2.9. Adsorption thermodynamics
To understand the nature of the adsorption process for CV dye adsorption onto NAJL powder adsorbent, the experiments were achieved at various temperatures (25, 35 and 45°C) and concentration (50,100 and 150 mg/L). Thermodynamic factors including change in free energy (ΔGo) (EquationEquation (11)(11)
(11) ), enthalpy (ΔHo) and entropy (ΔSo) (EquationEquation (12))
(12)
(12) were calculated using the following equations:
where T (K) is the temperature in Kelvin, R is the gas constant (8.314 J mol−1 K−1). Kc is the equilibrium constant. The values of ΔHo and ΔSo were calculated from the slope and intercept of the plot of ln Kc versus (1/T) as presented in . presents the thermodynamic factors for CV dye adsorption onto NAJL powder adsorbent. The results revealed that the negative values of ΔH° and ΔS° verifies the exothermic nature of CV dye adsorption onto NAJL powder adsorbent and reduced in randomness. The negative values of ΔG° indicating the spontaneous of the adsorption process [Citation73,Citation74]. The value of ΔH° was 29.8 kJ/mol that means the CV dye adsorption onto NAJL powder adsorbent was physisorption [Citation75].
Table 6. Thermodynamic parameters for the adsorption of CV on NAJL powder adsorbent
3.3. Adsorption mechanism
The FTIR analysis results in shown that the existence of negatively charged binding sites such as carboxylic (COOH), and hydroxyl (–OH) and (–C = O) groups onto the NAJL powder adsorbent surface that can be strongly adsorbed CV dye from aqueous solutions by different mechanisms included π-π interactions between the aromatic ring molecule in the NAJL powder adsorbent surface and the aromatic ring in the CV dye molecule, electrostatic interactions between –COO− and –OH groups in the surface of NAJL powder and +N(CH3)2 in the CV dye molecule, hydrogen bonding between dimethylamino (−N(CH3)2) group of CV dye and -OH group of the NAJL powder adsorbent surface.
3.4. Desorption study
Two eluents (0.1 M HNO3 and 0.1 M HCl) were used for desorption of CV dye from NAJL powder adsorbent surface. The CV dye-loaded NAJL powder was washed with deionized water and then added into 50 mL of 0.1 M HNO3 as eluent. The mixture was shaken at 200 rpm for 30 min. After that, the mixture was separated, and the residual CV dye concentration was determined by UV/Visible spectrometer. The percentage desorption was calculated by the following EquationEquation (13)(13)
(13) :
The results show that the best eluent for recovery of CV dye from NAJL powder adsorbent surface was 0.1 M HNO3 (39%) compared to 0.1 M HCl (14%) ().
4. Conclusion
The present study shows that NAJL powder is an effective adsorbent for the elimination of CV dye from aqueous solution. The adsorbent performance of NAJL powder was strongly affected by different parameters, such as pH initial CV dye ion concentration, temperature, contact time and adsorbent dose. Langmuir isotherm model is well fitted for the equilibrium data adsorption of CV dye compared with Freundlich and Dubinin–Radushkevich models, indicating monolayer adsorption with maximum adsorption capacity of 315.2 mg/g of the NAJL powder at 25°C and pH 9. The CV dye adsorption onto NAJL powder was fitted well to pseudo-first-order model indicating physisorption. The calculated thermodynamic parameters exhibited that the adsorption process was exothermic and spontaneous. Generally, the results obtained suggest that NAJL powder can be applied as an efficient adsorbent and environmentally friendly for the elimination of CV dye from aqueous solutions.
Acknowledgments
We thank the King Saud University, Deanship of Scientific Research for funding this work through the research group No. RG-1441-305. We gratefully acknowledge the financial support by Albaha University (Project No. 1441/3) and are grateful to the Scientific Research.
Disclosure statement
The author declares no competing financial interest.
References
- Alqadami AA, Naushad M, Alothman ZA, et al. Adsorptive performance of MOF nanocomposite for methylene blue and malachite green dyes: kinetics, isotherm and mechanism. J Environ Manage. 2018;223:29–36.
- Gautam D, Hooda S. Magnetic graphene oxide/Chitin nanocomposites for efficient adsorption of methylene blue and crystal violet from aqueous solutions. J Chem Eng Data. 2020;65(8):4052–4062.
- Alharthi FA, Al-Zaqri N, El marghany A, et al. Synthesis of nanocauliflower ZnO photocatalyst by potato waste and its photocatalytic efficiency against dye. J Mater Sci Mater Electron. 2020;31(14):11538–11547.
- Zhang L, Yang K. Facilely magnetized CuZnFe2O4 activated carbon composite as an efficient recyclable adsorbent of cationic dyes. J Chem Eng Data. 2020;65(11):5532–5544.
- Alqadami AA, Naushad M, Abdalla MA, et al. Adsorptive removal of toxic dye using Fe3O4–TSC nanocomposite: equilibrium, kinetic, and thermodynamic studies. J Chem Eng Data. 2016;61(11):3806–3813.
- Mohan N, Balasubramanian N, Basha CA. Electrochemical oxidation of textile wastewater and its reuse. J Hazard Mater. 2007;147(1–2):644–651.
- Mane VS, Babu PVV. Studies on the adsorption of brilliant green dye from aqueous solution onto low-cost NaOH treated saw dust. Desalination. 2011;273(2–3):321–329.
- Zinadini S, Zinatizadeh AA, Rahimi M, et al. Novel high flux antifouling nanofiltration membranes for dye removal containing carboxymethyl chitosan coated Fe3O4 nanoparticles. Desalination. 2014;349:145–154.
- Wang C, Yediler A, Lienert D, et al. Ozonation of an azo dye C.I. Remazol black 5 and toxicological assessment of its oxidation products. Chemosphere. 2003;52(7):1225–1232.
- Vinay SP, Alharthi FA, Udayabhanu AA, et al. Hydrothermal synthesis of Ag/rGO@CTFE nanocomposite as a promising photocatalyst for degradation action. J Mol Struct. 2021;1228:129722.
- Krishnakumar B, Alsalme A, Alharthi FA, et al. Synthesis, characterization of gelatin assisted ZnO and its effective utilization of toxic azo dye degradation under direct sunlight. Opt Mater (Amst). 2021;113:110854.
- Naushad M, Alqadami AA, AlOthman ZA, et al. Adsorption kinetics, isotherm and reusability studies for the removal of cationic dye from aqueous medium using arginine modified activated carbon. J Mol Liq. 2019;293:111442.
- Aldawsari AM, Alsohaimi IH, Al-Kahtani AA, et al. Adsorptive performance of aminoterephthalic acid modified oxidized activated carbon for malachite green dye: mechanism, kinetic and thermodynamic studies. Sep Sci Technol. 2021;56(5):835–846.
- Khan MA, Wabaidur SM, Siddiqui MR, et al. Silico-manganese fumes waste encapsulated cryogenic alginate beads for aqueous environment de-colorization. J Clean Prod. 2020;244:118867.
- Alorabi AQ, Shamshi Hassan M, Azizi M. Fe3O4-CuO-activated carbon composite as an efficient adsorbent for bromophenol blue dye removal from aqueous solutions. Arab J Chem. 2020;13(11):8080–8091.
- Alorabi AQ, Alharthi FA, Azizi M, et al. Removal of lead(II) from synthetic wastewater by Lavandula pubescens decne biosorbent: insight into composition–Adsorption relationship. Appl Sci. 2020;10(21):7450.
- Jayasantha Kumari H, Krishnamoorthy P, Arumugam TK, et al. An efficient removal of crystal violet dye from waste water by adsorption onto TLAC/Chitosan composite: a novel low cost adsorbent. Int J Biol Macromol. 2017;96:324–333.
- Fabryanty R, Valencia C, Soetaredjo FE, et al. Removal of crystal violet dye by adsorption using bentonite – alginate composite. J Environ Chem Eng. 2017;5(6):5677–5687.
- Zhou Y, Zhang M, Wang X, et al. Removal of crystal violet by a novel cellulose-Based adsorbent: comparison with native cellulose. Ind Eng Chem Res. 2014;53(13):5498–5506.
- Cheruiyot GK, Wanyonyi WC, Kiplimo JJ, et al. Adsorption of toxic crystal violet dye using coffee husks: equilibrium, kinetics and thermodynamics study. Sci African. 2019;5:e00116.
- Alghamdi AA, Al-Odayni A-B, Abduh NAY, et al. Adsorptive performance of Polypyrrole-Based KOH-Activated carbon for the cationic dye crystal violet: kinetic and equilibrium studies. Krajišnik D, editor. Adsorpt Sci & Technol. 2021;2021:5527594.
- Ghosh K, Bar N, Biswas AB, et al. Elimination of crystal violet from synthetic medium by adsorption using unmodified and acid-modified eucalyptus leaves with MPR and GA application. Sustain Chem Pharm. 2021;19:100370.
- Ahmad MA, Ahmed NB, Adegoke KA, et al. Adsorptive potentials of lemongrass leaf for methylene blue dye removal. Chem Data Collect. 2021;31:100578.
- Hameed BH. Spent tea leaves: a new non-conventional and low-cost adsorbent for removal of basic dye from aqueous solutions. J Hazard Mater. 2009;161(2–3):753–759.
- Parvin S, Rahman MW, Saha I, et al. Coconut tree bark as a potential low-cost adsorbent for the removal of methylene blue from wastewater. Desalin Water Treat. 2019;146:385–392.
- Chen L, Wu P, Chen M, et al. Preparation and characterization of the eco-friendly chitosan/vermiculite biocomposite with excellent removal capacity for cadmium and lead. Appl Clay Sci. 2018;159:74–82.
- Bello OS, Lasisi BM, Adigun OJ, et al. Scavenging Rhodamine B dye using moringa oleifera seed pod. Chem Speciat Bioavailab. 2017;29(1):120–134.
- Ajaelu CJ, Dawodu MO, Faboro EO, et al. Copper biosorption by untreated and citric acid modified senna alata leaf biomass in a batch system : kinetics, equilibrium and thermodynamics studies. Physical Chemistry. 2017;7:31–41. .
- Bello OS, Alabi EO, Adegoke KA, et al. Rhodamine B dye sequestration using Gmelina arborea leaf powder. Heliyon. 2020;6(1):e02872.
- Bello OS, Banjo S. Equilibrium, kinetic, and quantum chemical studies on the adsorption of Congo red using Imperata cylindrica leaf powder activated carbon. Toxicol Environ Chem. 2012;94(6):1114–1124.
- Ojo TA, Ojedokun AT, Bello OS. Functionalization of powdered walnut shell with orthophosphoric acid for Congo red dye removal. Part Sci Technol. 2019;37(1):74–85.
- Lim LBL, Priyantha N, Chieng HI, et al. Artocarpus camansiBlanco (Breadnut) core as low-cost adsorbent for the removal of methylene blue: equilibrium, thermodynamics, and kinetics studies. Desalin Water Treat. 2016;57(12):5673–5685.
- Dahri MK, Kooh MRR, Lim LBL. Application of Casuarina equisetifolia needle for the removal of methylene blue and malachite green dyes from aqueous solution. Alexandria Eng J. 2015;54(4):1253–1263.
- Yagub MT, Sen TK, Ang HM. Equilibrium, kinetics, and thermodynamics of methylene blue adsorption by pine tree leaves. Water, Air, {&} Soil Pollut. 2012;223(8):5267–5282.
- Argun ME, Dursun S, Gur K, et al. Nickel adsorption on the modified pine tree materials. Environ Technol. 2005;26(5):479–488.
- Samejo MQ, Memon S, Bhanger MI, et al. Comparison of chemical composition of Aerva javanica seed essential oils obtained by different extraction methods. Pak J Pharm Sci. 2013;26:757–760.
- Boobalan S, Kamalanathan D. Spermidine influences enhanced micropropagation and antibacterial activity in Aerva javanica (Burm. F.) Shult. Ind Crops Prod. 2019;137:187–196.
- Anand SP, Doss A, Nandagopalan V. QUALITATIVE AND QUANTITATIVE ANALYSIS OF PHYTOCHEMICALS IN AERVA JAVANICA (BURM. F .) SHULT. Acta Biomedica Scientia. 2014;1:93–97.
- Xue Y, Gao B, Yao Y, et al. Hydrogen peroxide modification enhances the ability of biochar (hydrochar) produced from hydrothermal carbonization of peanut hull to remove aqueous heavy metals: batch and column tests. Chem Eng J. 2012;200–202:673–680.
- Huff MD, Lee JW. Biochar-surface oxygenation with hydrogen peroxide. J Environ Manage. 2016;165:17–21.
- Jia Z, Li Z, Ni T, et al. Adsorption of low-cost absorption materials based on biomass (Cortaderia selloana flower spikes) for dye removal: kinetics, isotherms and thermodynamic studies. J Mol Liq. 2017;229:285–292.
- Zhou Y, Jin Q, Hu X, et al. Heavy metal ions and organic dyes removal from water by cellulose modified with maleic anhydride. J Mater Sci. 2012;47(12):5019–5029.
- Jain SN, Gogate PR. Acid Blue 113 removal from aqueous solution using novel biosorbent based on NaOH treated and surfactant modified fallen leaves of Prunus Dulcis. J Environ Chem Eng. 2017;5(4):3384–3394.
- Han X, Wang W, Ma X. Adsorption characteristics of methylene blue onto low cost biomass material lotus leaf. Chem Eng J. 2011;171(1):1–8.
- Jain SN, Gogate PR. NaOH-treated dead leaves of Ficus racemosa as an efficient biosorbent for acid blue 25 removal. Int J Environ Sci Technol. 2017;14(3):531–542.
- Nawaz H, Shad MA, Andleeb H, et al. Phytochemical and antioxidant composition of Desert Cotton (Aerva javanica) crude extracts in solvents with varying polarity. Int J Pharm Life Sci. 2015;6:4238–4246. http://eds.b.ebscohost.com.ezproxy.taylors.edu.my/eds/pdfviewer/pdfviewer?vid=19&sid=c9750d1b-6020-42db-8db0-fce28bfa12e2@sessionmgr112&hid=113
- Nandiyanto ABD, Oktiani R, Ragadhita R. How to read and interpret FTIR spectroscope of organic material. Indones J Sci Technol. 2019;4(1):97–118.
- Yao Y, Wang C, Wang X, et al. Activation of fulvic acid-like in paper mill effluents using H2O2/TiO2 catalytic oxidation: characterization and salt stress bioassays. J Hazard Mater. 2019;378:120702.
- Jain SN, Gogate PR. Adsorptive removal of acid violet 17 dye from wastewater using biosorbent obtained from NaOH and H2SO4 activation of fallen leaves of Ficus racemosa. J Mol Liq. 2017;243:132–143.
- Geçgel Ü, Üner O, Gökara G, et al. Adsorption of cationic dyes on activated carbon obtained from waste Elaeagnus stone. Adsorpt Sci Technol. 2016;34(9–10):512–525.
- Guo Y, Yang S, Yu K, et al. The preparation and mechanism studies of rice husk based porous carbon. Mater Chem Phys. 2002;74(3):320–323.
- Le Van K, Luong Thi TT. Activated carbon derived from rice husk by NaOH activation and its application in supercapacitor. Prog Nat Sci Mater Int. 2014;24(3):191–198.
- Chakraborty S, Chowdhury S, Das Saha P. Adsorption of Crystal Violet from aqueous solution onto NaOH-modified rice husk. Carbohydr Polym. 2011;86(4):1533–1541.
- Kenawy E-R, Ghfar AA, Wabaidur SM, et al. Cetyltrimethylammonium bromide intercalated and branched polyhydroxystyrene functionalized montmorillonite clay to sequester cationic dyes. J Environ Manage. 2018;219:285–293.
- Qiao H, Zhou Y, Yu F, et al. Effective removal of cationic dyes using carboxylate-functionalized cellulose nanocrystals. Chemosphere. 2015;141:297–303.
- Azman A, Ngadi N, Zaini DKA, et al. Effect of adsorption parameter on the removal of aspirin using tyre waste adsorbent. Chem Eng Trans. 2019;72:157–162.
- Sahu O, Singh N. Significance of bioadsorption process on textile industry wastewater. The impact and prospects of green chemistry for textile technology. Elsevier Ltd. 2018. DOI:https://doi.org/10.1016/B978-0-08-102491-1.00013-7
- Sabna V, Thampi SG, Chandrakaran S. Adsorption of crystal violet onto functionalised multi-walled carbon nanotubes: equilibrium and kinetic studies. Ecotoxicol Environ Saf. 2016;134:390–397.
- Tanhaei B, Ayati A, Iakovleva E, et al. Efficient carbon interlayed magnetic chitosan adsorbent for anionic dye removal: synthesis, characterization and adsorption study. Int J Biol Macromol. 2020;164:3621–3631.
- Saeed A, Sharif M, Iqbal M. Application potential of grapefruit peel as dye sorbent: kinetics, equilibrium and mechanism of crystal violet adsorption. J Hazard Mater. 2010;179(1–3):564–572.
- Tharaneedhar V, Kumar PS, Saravanan A, et al. Prediction and interpretation of adsorption parameters for the sequestration of methylene blue dye from aqueous solution using microwave assisted corncob activated carbon. Sustain Mater Technol. 2017;11:1–11.
- Ho Y-S, Chiu W-T, Wang -C-C. Regression analysis for the sorption isotherms of basic dyes on sugarcane dust. Bioresour Technol. 2005;96(11):1285–1291.
- Shoukat S, Bhatti HN, Iqbal M, et al. Mango stone biocomposite preparation and application for crystal violet adsorption: a mechanistic study. Microporous Mesoporous Mater. 2017;239:180–189.
- Wallis A, Dollard MF. Local and global factors in work stress - The Australian dairy farming examplar. Scand J Work Environ Heal Suppl. 2008;6:66–74.
- Über die Adsorption in Lösungen. Zeitschrift für Physikalische Chemie. 1907. p. 385. doi:https://doi.org/10.1515/zpch-1907-5723
- Dubinin MM, LVR. Equation of the characteristic curve of activated charcoal. Chem Zentr. 1947;1:857.
- Patil SR, Sutar SS, Jadhav JP. Sorption of crystal violet from aqueous solution using live roots of Eichhornia crassipes: kinetic, isotherm, phyto and cyto-genotoxicity studies. Environ Technol Innov. 2020;18:100648.
- Das SP, Chakraborty S, Chowdhury S. Batch and continuous (fixed-bed column) biosorption of crystal violet by Artocarpus heterophyllus (jackfruit) leaf powder. Colloids Surf B Biointerfaces. 2012;92:262–270.
- Khanam N Adsorptive removal of crystal violet by banyan leaf powder : batch and column adsorptive removal of crystal violet by banyan leaf powder : batch and Column Study. 2017.
- Kumar R, Ahmad R. Biosorption of hazardous crystal violet dye from aqueous solution onto treated ginger waste (TGW). Desalination. 2011;265(1–3):112–118.
- Roginsky S, YBZ. The catalytic oxidation of carbon monoxide on manganese dioxide. Acta Phys Chem USSR. 1934;1:554.
- Lagergren S. About the theory of so-called adsorption of soluble substances. Handlingar. 1898;24:1–39.
- Ashrafi A, Rahbar-Kelishami A, Shayesteh H. Highly efficient simultaneous ultrasonic assisted adsorption of Pb (II) by Fe3O4@MnO2 core-shell magnetic nanoparticles: synthesis and characterization, kinetic, equilibrium, and thermodynamic studies. J Mol Struct. 2017;1147:40–47.
- Feng G, Ma J, Zhang X, et al. Magnetic natural composite Fe3O4-chitosan@bentonite for removal of heavy metals from acid mine drainage. J Colloid Interface Sci. 2019;538:132–141.
- Alghamdi AA, Al-Odayni A-B, Saeed WS, et al. Adsorption of Azo dye methyl orange from aqueous solutions using alkali-activated Polypyrrole-Based graphene oxide. Molecules. 2019;24(20):3685.