ABSTRACT
In this study, we investigated the potential of swallow feces as a bioindicator to evaluate soil pollution from anthropogenic sources. This biomonitoring was performed with trace elements, polycyclic aromatic hydrocarbons (PAHs), and their chlorinated derivatives (ClPAHs) as pollutants. The concentration of total trace elements targeted was reasonably consistent in both soil and fecal samples. The composition of individual trace elements also showed a similar profile across the sampling sites. The concentration of total PAHs varied in both soil and fecal samples among the sites, whereas their composition showed a similar profile. There were no significant correlations between total PAH or total ClPAH concentrations in either soil or fecal samples. This suggests that the sources and metabolic pathways in swallows may differ between PAHs and ClPAHs. Predictions of individual contaminant levels in soil samples were made from corresponding datasets of the feces using partial least squares analysis, and showed significant correlations based on the magnitude order. It permitted to roughly complement the levels of contaminants in the soils by the estimated equation.
1. Introduction
Biomonitoring using wildlife has become increasingly common as a useful approach to explore environmental pollution, with birds frequently chosen as an appropriate organism [Citation1–4]. Birds are useful bioindicators of environmental problems because they are conspicuous organisms that are relatively easy to observe [Citation1]. Furthermore, birds occupy a high position in the food chain, so they are susceptible to environmental contaminants, especially persistent compounds that undergo biomagnification and bioconcentration. Among birds used as bioindicators, raptors and seabirds are frequently used because they occupy the top position in food chains in terrestrial and aquatic ecosystems, respectively [Citation1]. Research has shown that seabirds are often affected by pollution in the marine environment [Citation1,Citation5–7]. The potential of various body parts of birds as bioindicators have been investigated and their usefulness has been confirmed. Feathers and eggs in particular are biomaterials that are known to be useful indicators and have been widely investigated in various sites to assess biological and environmental pollution [Citation3,Citation4,Citation8,Citation9].
Concerning the relationships between birds and environmental pollution, there have been a number of reports that show the contribution of heavy metals in invertebrates to soil pollution [Citation10–13]. Among them, swallows are recognized to be useful indicators of environmental pollution as the biotransfer of pollutants can occur via their diet of insects [Citation1]. For example, the fecal concentrations of non-essential elements of barn swallows were attributable to those in the insect prey [Citation11]. Hedde and Van Oort [Citation14], reported that certain trace elements in invertebrates are influenced by the soil pollution. Furthermore, fecal sampling of birds characterizes their overall exposure to polycyclic aromatic compounds (PACs) but not the sources of exposure to PACs for the birds [Citation15]. These findings suggest that soil pollution could be transferred through the food web to aerial insectivores, including swallows. According to Bryant and Turner [Citation16], the range of barn swallows are generally within approximately 1 km from their nest, so we hypothesized that biomonitoring using the feces of barn swallows would be a possible means to evaluate local soil pollution.
Soil pollution by chemicals is driven by specific processes depending on the site. For example, automobiles are a typical anthropogenic source of pollution, causing not only air pollution from exhaust components but also soil pollution from road dust and atmospheric fallout. That is, soil pollution has an intimate relationship with anthropogenic activities in the surrounding environment. As described above, the biomonitoring of certain elements in the food web using swallows has been partially investigated and resulted in quantitative evaluations, whereas investigations of polycyclic aromatic hydrocarbons (PAHs) have been far more limited. Fernie et al. [Citation15], reported that PAH concentrations in the muscles of tree swallows significantly correlated with those in the feces, suggesting that fecal PAHs could represent PAH exposure of birds. On the other hand, there is no information relating to the biomonitoring of chlorinated PAHs (ClPAHs) in birds. ClPAHs are a relatively new group of anthropogenic pollutants, but they are ubiquitously distributed in the environment [Citation17,Citation18]. The objectives of the present study were to evaluate the possibility of using the feces of barn swallows (Hirundo rustica) as a bioindicator for assessing local soil pollution caused by anthropogenic activities. The evaluation was carried out using not only trace elements and PAHs but also ClPAHs as a novel contaminant indicator.
2. Materials and methods
2.1. Sampling sites and method
The sampling of barn swallow feces and surface soils was performed from May to June 2017, at urban, suburban, park, and local sites in central Japan (). Fecal samples were collected in boxes that had an interior coated with aluminum foil. The boxes were placed below the nests of barn swallows to collect feces and left for a few days to 1 week. The urban site was 9 km east of the center of Nagoya city, a mega city in Japan with a population of approximately 2.3 million people. At the urban site, two samples of swallow feces were collected from the eaves of commercial buildings. In addition, samples of surface soil from the urban site were randomly collected from four points (soil from a park and a roadside plant) within a 1 km radius from the swallow feces sampling point. The sampling point at the suburban site was located in Inuyama city, approximately 20 km north of the center of Nagoya city, with a population of around 70,000 people. At the suburban site, two samples of swallow feces were collected from the edge of the eaves of a private house on different days. Five samples of surface soils were randomly collected within a 1 km radius from the swallow feces sampling point. Although the park site was located near to the urban site (within 5 km), some relatively large natural parks (0.6–1.5 km2) were present in the area. At the site, four samples of swallow feces were collected from the eaves of three commercial buildings. Thirteen samples of surface soils were collected from the natural parks and a planted area by the roadside within a 1 km radius from the swallow feces sampling point. The local area was located in the northern part of the Japanese midlands, which is approximately 130 km away from the urban site (). The population of the area is around 1,500 people. The area is typical of the farming villages found in Japan and is surrounded by forests. In this area, six samples of swallow feces were collected from the edge of the eaves of private houses. Nine soil samples were randomly collected from field and roadside soil surrounding the houses where the swallow fecal samples were collected (within 1 km). The collected samples were freeze-dried and stored in centrifuge tubes at −28°C until extraction.
2.2. Chemicals
The target compounds were 24 ClPAHs. Detailed procedures for synthesizing 16 of these have been described previously [Citation19,Citation20]. In addition, 18 PAHs, including 16 that have been classed as priority pollutants by the US Environmental Protection Agency, were analyzed. Standards of the PAHs were purchased from Sigma–Aldrich (Saint Louis, MO, USA). Three deuterated PAHs (phenanthrene-d10, fluoranthene-d10, and perylene-d12) purchased from Cambridge Isotope Laboratories, Inc. (Andover, MA, USA), was used as internal standards. The solvents used in the extraction and cleanup were of pesticide analysis grade, purchased from Wako Pure Chemical (Osaka, Japan) or Kanto Chemical (Tokyo, Japan). The 24 ClPAHs analyzed were 9-chlorophenanthrene (9-ClPhe), 1,9-dichlorophenanthrene (1,9-Cl2Phe), 3,9-dichlorophenanthrene (3,9-Cl2Phe), 9,10-dichlorophenanthrene (9,10-Cl2Phe), 3,9,10-trichlorophenanthrene (3,9,10-Cl3Phe), 2-chloroanthracene (2-ClAnt), 9-chloroanthracene (9-ClAnt), 9,10-dichloroanthracene (9,10-Cl2Ant), 3-chlorofluoranthene (3-ClFluor), 8-chlorofluoranthene (8-ClFluor), 1,3-dichlorofluoranthene (1,3-Cl2Fluor), 3,4-dichlorofluoranthene (3,4-Cl2Fluor), 3,8-dichlorofluoranthene (3,8-Cl2Fluor), 1-chloropyrene (1-ClPy), dichloropyrene (Cl2Py), trichloropyrene (Cl3Py), tetrachloropyrene (Cl4Py), 6-chlorochrysene (6-ClChry), 6,12-dichlorochrysene (6,12-Cl2Chry), 7-chlorobenz[a]anthracene (7-ClBaA), 7,12-dichlorobenz[a]anthracene (7,12-Cl2BaA), 6-chlorobenzo[a]pyrene (6-ClBaP), dichlorobenzo[a]pyrene (Cl2BaP), and trichlorobenzo[a]pyrene (Cl3BaP). The 18 PAHs analyzed were naphthalene (Nap), acenaphthylene (Acy), acenaphthene (Ace), fluorine (Flu), phenanthrene (Phe), anthracene (Ant), fluoranthene (Fluor), pyrene (Py), benzo[a]anthracene (BaA), chrysene (Chry), benzo[b]fluoranthene (BbF), benzo[k]fluoranthene (BkF), benzo[e]pyrene (BeP), benzo[a]pyrene (BaP), Perylene (Pery), benzo[ghi]perylene (BghiP), dibenzo[a,h]anthracene (DBahA), and indeno[1,2,3-cd]pyrene (IP). The following eleven species of elements were targeted (abbreviations): vanadium (V), chromium (Cr), manganese (Mn), cobalt (Co), nickel (Ni), copper (Cu), zinc (Zn), arsenic (As), cadmium (Cd), mercury (Hg), and lead (Pb).
2.3. Sample extraction and cleanup
Soxhlet extraction with hexane–acetone (1:1) was used to determine the concentration of PAHs and ClPAHs in soil and swallow feces samples. One to 2 g of dried soil or feces spiked with Fluor-d10 (16 ng) and Pery-d12 (24 ng) as surrogates were placed in a cellulose thimble and extracted with 200 ml hexane–acetone solution for 16 h. The extracts were concentrated to 2 ml using a rotary evaporator, followed by concentrations to 0.2 mL using a gentle stream of N2.
The extract was cleaned using a silica gel column (Supelclean LC-Si SPE, Sigma-Aldrich, St. Louis, MO, USA). The packed column with 1 g silica gel was pre-cleaned and conditioned with 20 mL n-hexane. After the sample was loaded, the target PAHs and ClPAHs were eluted with 12 mL of n- hexane. The eluted solution was pre-concentrated to 0.5 mL and cleaned further by activated alumina column (1 g). After the sample was loaded, the first fraction was eluted with 15 mL hexane and was discarded since there were no analytes contained in this fraction. The second fraction contained the targets and was eluted with 10 mL 30% dichloromethane–hexane. The latter fraction was concentrated to 0.2 mL by N2 purge and transferred into amber glass vials. At this stage, Phe-d10 (18 ng) was added as a syringe spike, and the solution was analyzed by gas chromatography–mass spectrometry (GC–MS).
2.4. ClPAH and PAH analysis
Chemical analyses of PAHs and ClPAHs were performed using a JMS-Q1000GC quadrupole MS (JEOL, Tokyo) equipped with a 7890A (Agilent Technologies, Santa Clara, CA) GC with an InertCap 5 MS/NP capillary column (30 m × 0.25 mm i.d. × 0.25 μm film thickness, GL Science Inc., Tokyo). The MS was operated in selected ion monitoring mode. The ion current was maintained at 200 μA. Helium was used as a carrier gas at a flow rate of 1.0 mL/min. For the ClPAH analysis, the oven temperature was kept at 100°C for 2 min, then increased to 200°C at a rate of 25°C/min until the oven reached 200°C with no hold, and then increased to 300°C at a rate of 5°C/min, then kept at 300°C for 15 min. The temperatures of the injector and GC–MS transfer line were maintained at 300°C and 280°C, respectively. The MS system was run in the electron impact ionization mode, and the electron energy was 70 eV. For the PAH analysis, the oven temperature was kept at 70°C for 3 min, then increased to 240°C at a rate of 20°C/min until the oven reached 240°C with no hold, then increased to 310°C at a rate of 5°C/min, and then kept at 310°C for 30 min. The temperatures of the injector and GC–MS transfer line were maintained at 280°C and 280°C, respectively. Detailed information about the GC–MS analytical conditions is described elsewhere [Citation21].
2.5. Element analysis
Eleven trace elements in the soil and swallow fecal samples were analyzed by means of energy dispersive X-ray fluorescence (EDXRF) spectrometry. The sample preparation was carried out as follows. Approximately 0.1 g of dried soil or feces was weighed out then homogenized using pestle and mortar. The powder was set in a dedicated holder and the analysis was performed. The EDXRF apparatus (EDXL300, Rigaku, Tokyo, Japan) consisted of a Pd-anode X-ray tube (50 W), four secondary targets (Mo of 50 kV and 200 s, Cu of 50 kV and 100 s, Al of 50 kV and 100 s, and RX9 (graphite crystal) of 25 kV and 100 s), and a silicon drift detector. The analyses were controlled by a PC data acquisition system. Quantification of each element in the samples was performed using the fundamental parameter (FP) method. The advantage of using the FP standardless analysis is that there is no need to prepare standard materials for each target element [Citation22].
2.6. Quality assurance
For the quality assurance of the current analytical procedure, a recovery test of target compounds using a sequence of analytical procedures was done, and recoveries were confirmed to be reasonable in our previous work [Citation21]. Briefly, the recoveries of PAH standards spiked into the blank samples ranged from 48 ± 6% for Nap to 103 ± 1% for Pery. In addition, the recoveries of surrogates spiked into the fecal and soil samples were 79 ± 9% for Fluor-d10 and 71 ± 20% for Pery-d12 and 77 ± 12% for Fluor-d10 and 90 ± 22% for Pery-d12, respectively (Table S1). The target ClPAHs were not detected in the procedural blank samples. The analytical precision of element analysis by EDXRF was confirmed using NIES CRM No. 2 Pond Sediment as an authenticated standard sample for element analysis (Shimadzu Co Ltd., Kyoto, Japan). The accuracies of the measured values to certified values ranged from 94.8% for Cu to 108% for As.
2.7. Data analysis
For data analysis of the soil and fecal samples, correlation analysis and partial least squares (PLS) analysis were carried out using the statistical analysis software Excel 2019 and ORIGIN 2016 (OriginLab Corp., MA, USA). In this study, PLS was performed to estimate the predicted values from the corresponding measured values. PLS is a multivariable regression technique capable of determining the relationship between a data matrix Y (dependent variables) and a data matrix X (independent variable). Relationships between X and Y variables are found by simultaneous projections of both the X and Y spaces to a plane or hyperplane. Here, individual concentrations of compounds in each fecal sample and the mean concentrations in soil samples from all sampling sites were used as independent and dependent variables, respectively.
3. Results and discussion
3.1. Occurrence of trace elements in soils and swallow feces
The mean concentrations of eleven species of trace elements in surface soils and swallow feces at each sampling site are summarized in . For all soil samples, Mn was the most abundant species, with a range of 555 to 764 μg/g, followed by Zn with a range of 126 to 205 μg/g, and V with a range of 75 to 110 μg/g. Although Mn in soil may sometimes be viewed as an indicator of anthropogenic activities, such as petrol combustion, in most cases it is considered to be an element of natural origin [Citation23]. In the present study, Mn in the soil samples was similar to levels seen in soils defined as being of natural origin [Citation23]. Therefore, these levels of Mn meant it was unsuitable as an indicator of anthropogenic impacts. For Zn, an indicator of typical anthropogenic activities, the current levels in the soil at other local sites were considerably higher compared with the mean concentration in 27 cities (50 μg/g on average) and were above the limit (140 μg/g) of sustainable soil quality proposed by the Dutch Ministry [Citation24,Citation25]. For other anthropogenic elements, such as Cr, Ni, and Cd, their concentrations in this study were almost the same as or less than the corresponding world means in soils as follows: 40 μg/g for Cr, 20 μg/g for Ni, and 0.3 μg/g for Cd [Citation24]. Consequently, soil pollution caused by anthropogenic activities could have had relatively little impact on the sampling sites selected in this study.
Table 1. Mean concentrations ± standard deviation (μg/g) of individual trace elements in surface soils and the feces of barn swallows throughout the sampling sitesa.
Of the trace elements in the fecal samples, the concentrations were overall at lower levels than those seen in soils, except for Cu and Zn (). The concentrations of Cu and Zn in the fecal samples were at significantly higher levels compared with other elements. Such specific contents in feces have been widely observed, not only in birds but also in pigs and cattle [Citation26–28]. According to Ancora et al. [Citation29], the highly excretion to feces would be associated with specific metabolism such as metallothionein complexes form in addition to influence of the habitat environment. Although the contaminant levels varied among the sites, the composition of both soils and feces was similar among them ().
3.2. Occurrence of PAHs in soils and swallow feces
Of the PAHs in the surface soils, the total concentration was 1,190 ng/g on average in all samples, and the mean levels at each site were as follows: urban (1,940 ng/g) > suburban (1,410 ng/g) > local (1,330 ng/g) > park (779 ng/g) (). These contamination levels were relatively high compared with levels previously reported for Asian soils [Citation30]. Compared to the composition of aromatic rings among the sampling sites, PAHs with relatively high molecular weights (6-rings) were dominant in samples from the urban site, followed by the suburban, park, and local sites, whereas the rates of PAHs with relatively low molecular weights (≤3-rings) increased in the inverse order ().
Figure 3. The concentration of (a, b) total PAHs and (c, d) total ClPAHs in (a, c) surface soils and (b, d) barn swallow feces at each site
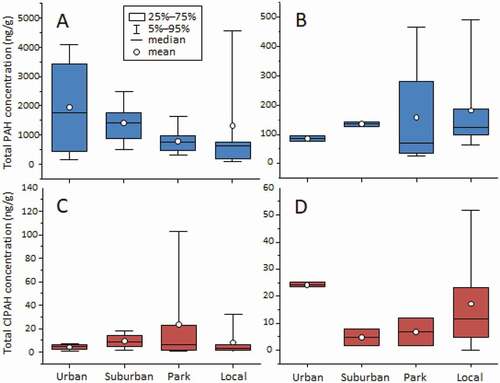
Figure 4. The composition of aromatic rings for PAHs in (a) surface soils and (b) barn swallow feces at each site
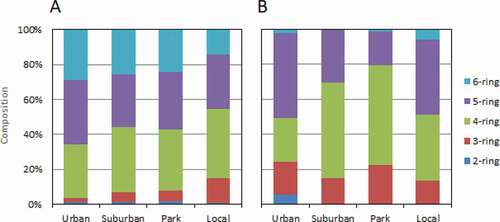
For the swallow fecal samples, the mean concentrations of total PAHs were approximately ten-fold lower than those in the soils, and showed an inverse trend to the soils, as follows: local (182 ng/g) > park (159 ng/g) > suburban (136 ng/g) > urban (86.8 ng/g) (). When comparing the PAH composition of the feces, the profiles were somewhat similar to those seen in soils, although the contribution of 6-ring PAHs was lower than that in the soils (). Fernie et al. [Citation15], suggested that PAH profiles in the muscles of tree swallows are influenced by contaminants in environmental matrices such as air and water, but not diet. These findings are expected that the fecal PAHs are not a little influenced by the soil contaminants since their content profiles are similar to each other, whereas detailed metabolic mechanisms such as assimilation and elimination of PAHs in the swallows remain unclear.
The mean concentrations of individual PAHs in the soils and fecal samples in each site are listed in Table S1. In the soil samples, the concentrations of IP and BghiP were dominant species among sites. In general, vehicular exhaust is considered as a major source of such high molecular weight PAHs in urban environments [Citation31]. In particular, the high percentage of BghiP in the soils is attributable to their continuous emission and subsequent deposition [Citation32]. Therefore, the major sources of PAHs in the soil samples in the present study could be attributed to vehicular exhaust. On the other hand, PAHs in the fecal samples, the dominant species varied among the sites. These discordancy of PAH levels between the soil and fecal samples could support the suggestion that fecal PAHs are not a little influenced by the soil contaminants as described in above.
3.3. Occurrence of ClPAHs in soils and swallow feces
There is no information concerning the contamination of ClPAHs in residential surface soils. In this study, all 24 targeted ClPAHs were detected in the surface soils, with total concentrations ranging from 0.48 to 103 ng/g across the sites (). The mean concentration of total ClPAHs was 14.4 ng/g, which was approximately 2 orders of magnitude lower than that of total PAHs. Such differences between ClPAH and PAH concentrations have been widely observed in other environmental media, such as air and water. Therefore, the contamination of the surface soils with ClPAHs and PAHs may have been mainly due to deposition from the air. On the other hand, 21 species of the 24 targets were detected in the fecal samples. The mean concentration of total ClPAHs in fecal samples was 14.6 ng/g, which was somewhat consistent with that of ClPAHs in the soil samples.
The mean concentrations of individual ClPAH in the soils and fecal samples in each site are listed in Table S1. Compared to individual ClPAH concentrations in the surface soil samples, 6-ClChry was the most dominant, with 3.61 ng/g on average, followed by Cl3Py at 1.95 ng/g, and 3,8-Cl2Fluor at 1.46 ng/g. On the other hand, in the feces, 6,12-Cl2Chry was dominant, at 5.29 ng/g on average, followed by Cl2BaP at 4.06 ng/g and 7,12-Cl2BaA at 3.38 ng/g (Table S1). These dominant species of ClPAHs were quite different in both samples.
Comparing the concentrations of total ClPAHs in the soils among the sites, the highest concentration was observed in samples from the park, at 23.5 ng/g, followed by suburban (9.6 ng/g), local (8.3 ng/g), and urban (4.4 ng/g) samples (). However, their order in the fecal samples was quite different, as follows: urban (24.3 ng/g) > local (17.2 ng/g) > park (6.9 ng/g) > suburban (4.7 ng/g) (). For the compositional analysis of ClPAHs in the soils, their profiles were somewhat similar, with the exception of the local samples (Figure S1A). On the other hand, the composition of ClPAHs in the fecal samples was quite different from the profiles in soils (Figure S1B). Interestingly, the contribution of relatively high molecular weight ClPAHs was elevated in the fecal samples compared with their contribution in the soil samples. This fact could make the hypothesis that such ClPAHs are poorly absorbed by swallows and easily eliminated in their feces. Such differences in ClPAH profile between the soils and feces could be the result of differences not only in accumulation processes but also in degradation processes. To date, the information on sources of ClPAHs in the air are limited in some reports that showed waste combustion, vehicular emissions and coal burning as the possible sources [Citation33]. However, there is no information about the behavior of ClPAHs in surface soils or biological tissues, so further studies are needed to reveal whether they persist in the environment.
3.4. Relationships between anthropogenic pollutants in surface soils and swallow feces
First, to evaluate the relationship between contaminants in the feces of swallows and surface soils, we compared their mean concentrations of individual trace elements. The relationship shows significant correlations, as shown in Figure S2, which shows data from the local site. In this relationship, the concentration of Zn in the fecal samples was shown to be higher than that in surface soils, whereas other elements in the fecal samples tended to be present at considerably lower concentrations than in surface soils. Indeed, the concentration of Zn in fecal samples was highest at every site, but this was not the case in soils (). Such high accumulations of Zn in feces have been observed in other animals, such as chickens and pigs, suggesting that high levels of Zn excretion could be due to biological features of animals rather than intake from environmental pollution [Citation27,Citation28]. Similar relationships among trace elements between the soil and fecal samples were observed at the other sites (Figure S3). These findings suggest that trace elements in feces are strongly reflected in the soils in the habitat area, so that the magnitude order of trace elements between soils and feces could be invariance over the sites. Therefore, trace elements in the feces of swallows have the potential to provide estimates of the contamination of surrounding soils.
Due to the relationships among individual PAHs, significant correlations were observed at the local site but not at the other sites, which was quite different from the trace elements (Figure S4A). In addition, the relationships of uncorrelated sites such as urban sites showed higher concentrations of PAHs in the soils rather than in the fecal samples (Figure S4A). Therefore, PAHs in the current sampling sites, with the exception of the local site, could be influenced by various sources, resulting in the variations in PAH contamination seen in the surface soils. Similar relationships were observed for the ClPAHs. No significant correlations were found between individual ClPAH concentrations at any of the sites (Figure S4B). Since the sources of ClPAHs were not equally present over all the sites, their impacts on swallows could fluctuate.
3.5. Prediction of soil contamination
One purpose of biomonitoring is to evaluate the behavior of biological and environmental pollutants. Therefore, the investigation of the relationships among target contaminants in biological and environmental samples is important for evaluating their usefulness for biomonitoring assessments. Here, we use PLS to investigate whether the concentration of contaminants in surface soils can be estimated from their concentration in feces. The PLS plot for trace elements showed that the order of predicted concentrations was completely consistent with the measured concentrations (). Therefore, levels of trace elements in feces have the potential to be a useful indicator to predict their levels in surrounding surface soils. This would be due to an almost constant order of magnitude of trace elements in the surface soils irrespective of sampling sites. Concerning PAHs and ClPAHs, the predictions using PLS indicated similar significant correlations, suggesting that the level of these contaminants in soils can also be predicted from their concentrations in feces (). However, the correlations of these plots were weaker than those of the trace elements. The reason for the weaker correlations between PAHs and ClPAHs may be due to the influence of volatilization and biological and physical degradation processes. Taken together, our findings suggest that biomonitoring using the feces of house swallows has the potential for predicting the level of contaminants, including not only trace elements but also PAHs and ClPAHs, in environmental soils. This non-destructive monitoring method is easy and inexpensive, so it would provide an adequate first screening method for contaminants in soils.
4. Conclusion
The concentrations of anthropogenic contaminants, such as trace elements, PAHs, and ClPAHs were investigated in surface soils and the feces of swallows at four sites with different features. Although their concentration in soils varied across the sampling sites, the composition profiles of trace elements and PAHs were similar. This finding indicates that these contaminants are uniformly distributed throughout the environment and could cause ecological damage anywhere. The survey of anthropogenic contaminants in the feces of swallows also showed specific profiles. For certain contaminant species in swallow feces, the composition was similar to those seen in soils, suggesting a relationship between them. These common profiles in soil and fecal samples allow to complement the concentrations of soil contaminants using PLS. Consequently, biomonitoring using the feces of house swallows has potential for evaluating environmental pollution.
Acknowledgments
We are very grateful to the inhabitants of the area for their helpful support of the sampling. This work was partially supported by the Japan Society for the Promotion of Science, through a Grant-in-Aid for Scientific Research (17H01865).
Disclosure statement
No potential conflict of interest was reported by the author(s).
Additional information
Funding
References
- Becker PH. Chapter 19 biomonitoring with birds. In: Ba M, Am B, Hg Z, editors. Trace metals and other contaminants in the environment. Elsevier; 2003. p. 677–736.
- Dauwe T, Jaspers V, Covaci A, et al. Feathers as a nondestructive biomonitor for persistent organic pollutants. Environ Toxicol Chem. 2005;24(2):442–449.
- Jaspers VLB, Voorspoels S, Covaci A, et al. Can predatory bird feathers be used as a non-destructive biomonitoring tool of organic pollutants? Biol Lett. 2006;2(2):283–285.
- Malik RN, Zeb N. Assessment of environmental contamination using feathers of bubulcus ibis l., as a biomonitor of heavy metal pollution, Pakistan. Ecotoxicology 2009;18(5):522–536.
- Furness RW, Camphuysen K. Seabirds as monitors of the marine environment. ICES J Mar Sci. 1997;54(4):726–737.
- Renedo M, Amouroux D, Duval B, et al. Seabird tissues as efficient biomonitoring tools for hg isotopic investigations: implications of using blood and feathers from chicks and adults. Environ Sci Technol. 2018;52(7):4227–4234.
- Tsygankov VY, Lukyanova ON, Boyarova MD. Organochlorine pesticide accumulation in seabirds and marine mammals from the northwest pacific. Mar Pollut Bull. 2018;128:208–213.
- Klein R, Bartel-Steinbach M, Koschorreck J, et al. Standardization of egg collection from aquatic birds for biomonitoring - a critical review. Environ Sci Technol. 2012;46(10):5273–5284.
- Van den steen E, Pinxten R, Jaspers VLB, et al. Brominated flame retardants and organochlorines in the european environment using great tit eggs as a biomonitoring tool. Environ Int. 2009;35(2):310–317.
- Hothem RL, Trejo BS, Bauer ML, et al. Cliff swallows petrochelidon pyrrhonota as bioindicators of environmental mercury, cache creek watershed, California. Arch Environ Contam Toxicol. 2008;55(1):111–121.
- Orłowski G, Kamiński P, Karg J, et al. Variable contribution of functional prey groups in diets reveals inter- and intraspecific differences in faecal concentrations of essential and non-essential elements in three sympatric avian aerial insectivores: a re-assessment of usefulness of bird faeces in metal biomonitoring. Sci Total Environ. 2015;518-519:407–416.
- Custer CM, Yang C, Crock JG, et al. Exposure of insects and insectivorous birds to metals and other elements from abandoned mine tailings in three summit county drainages, Colorado. Environ Monit Assess. 2008;153(1–4):161.
- Brasso RL, Cristol DA. Effects of mercury exposure on the reproductive success of tree swallows (tachycineta bicolor). Ecotoxicology. 2008;17(2):133–141.
- Hedde M, Van Oort F, Lamy I. Functional traits of soil invertebrates as indicators for exposure to soil disturbance. Environ Pollut. 2012;164:59–65.
- Fernie KJ, Marteinson SC, Chen D, et al. Elevated exposure, uptake and accumulation of polycyclic aromatic hydrocarbons by nestling tree swallows (tachycineta bicolor) through multiple exposure routes in active mining-related areas of the athabasca oil sands region. Sci Total Environ. 2018;624:250–261.
- Bryant DM, Turner AK. Central place foraging by swallows (hirundinidae): the question of load size. Anim Behav. 1982;30(3):845–856.
- Jin R, Zheng M, Lammel G, et al. Chlorinated and brominated polycyclic aromatic hydrocarbons: sources, formation mechanisms, and occurrence in the environment. Prog Energy Combust Sci. 2020;76:100803.
- Ohura T. Environmental behavior, sources, and effects of chlorinated polycyclic aromatic hydrocarbons. ScientificWorldJournal. 2007;7:372–380.
- Ohura T, Fujima S, Amagai T, et al. Chlorinated polycyclic aromatic hydrocarbons in the atmosphere: seasonal levels, gas-particle partitioning, and origin. Environ Sci Technol. 2008;42(9):3296–3302.
- Ohura T, Kitazawa A, Amagai T, et al. Occurrence, profiles, and photostabilities of chlorinated polycyclic aromatic hydrocarbons associated with particulates in urban air. Environ Sci Technol. 2005;39(1):85–91.
- Kamiya Y, Ikemori F, Ohura T. Optimisation of pre-treatment and ionisation for gc/ms analysis for the determination of chlorinated pahs in atmospheric particulate samples. Int J Environ Anal Chem. 2015;95(12):1157–1168.
- Okuda T, Fujimori E, Hatoya K, et al. Rapid and simple determination of multi-elements in aerosol samples collected on quartz fiber filters by using edxrf coupled with fundamental parameter quantification technique. Aerosol Air Qual Res. 2013;13(6):1864–1876.
- Ljung K, Otabbong E, Selinus O. Natural and anthropogenic metal inputs to soils in urban Uppsala, Sweden. Environ Geochem Health. 2006;28(4):353–364.
- Ajmone-Marsan F, Biasioli M. Trace elements in soils of urban areas. Water Air Soil Pollut. 2010;213(1–4):121–143.
- John SG, Genevieve Park J, Zhang Z, et al. The isotopic composition of some common forms of anthropogenic zinc. Chem Geol. 2007;245(1–2):61–69.
- del Castilho P, Chardon WJ, Salomons W. Influence of cattle-manure slurry application on the solubility of cadmium, copper, and zinc in a manured acidic, loamy-sand soil. J Environ Qual. 1993;22(4):689–697.
- Liu X, Zhao S, Sun L, et al. P and trace metal contents in biomaterials, soils, sediments and plants in colony of red-footed booby (sula sula) in the dongdao island of South China Sea. Chemosphere. 2006;65(4):707–715.
- Zhou D-M, Hao X-Z, Wang Y-J, et al. Copper and zn uptake by radish and pakchoi as affected by application of livestock and poultry manures. Chemosphere. 2005;59(2):167–175.
- Ancora S, Volpi V, Olmastroni S, et al. Assumption and elimination of trace elements in adélie penguins from antarctica: a preliminary study. Mar Environ Res. 2002;54(3–5):341–344.
- Hong W-J, Li Y-F, Li W-L, et al. Soil concentrations and soil-air exchange of polycyclic aromatic hydrocarbons in five asian countries. Sci Total Environ. 2020;711:135223.
- Miguel AH, Kirchstetter TW, Harley RA, et al. On-road emissions of particulate polycyclic aromatic hydrocarbons and black carbon from gasoline and diesel vehicles. Environ Sci Technol. 1998;32(4):450–455.
- Agarwal T. Concentration level, pattern and toxic potential of pahs in traffic soil of Delhi, India. J Hazard Mater. 2009;171(1–3):894–900.
- Ohura T, Suhara T, Kamiya Y, et al. Distributions and multiple sources of chlorinated polycyclic aromatic hydrocarbons in the air over Japan. Sci Total Environ. 2019;649:364–371.