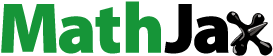
ABSTRACT
The treatment of a mature landfill leachate has been studied by the electrocoagulation process using aluminum electrodes. In this study, Doehlert experimental design was applied for the determination of the optimal operating conditions. The results were evaluated through the construction of isoresponse contour plots and the latter showed that an initial pH of 6, an electrolysis time of 90 min and a current density of 15 mA‧cm−2 were optimal, leading to an almost total leachate remediation. Indeed, under these conditions removal rates of 94% of COD and 97% of color were achieved. In addition, the proposed models were found appropriate for the results prediction. The electrocoagulation can be recommended as a feasible and effective technology for the removal of pollutants from aqueous medium.
1. Introduction
Landfill leachate is the liquid produced when moisture enters the refuse in a landfill or open dump, extracts contaminants into the liquid phase, and produces moisture content sufficiently high to initiate liquid [Citation1]. Landfill sites contain both household and commercial rubbish. The major components are food waste, paper, cardboard, plastic, rags, textile, metal and glass, although demolition and construction debris is often included in collected waste, as are small quantities of hazardous waste, such as discarded medicines, chemicals, lightbulbs and batteries. Because of the complex nature of waste discharged, their degree of decomposition, their moisture rate, and also the age of the landfills, leachates are highly toxic and can cause significant contamination of soils, rivers, groundwater and other bodies of water. This pollution affects trees, marine animals and humans. As a result, when implementing policies to minimize environmental effects, leachate collection and treatment for open dumpsites and landfills should be a top priority [Citation2].
Biological processes for young and biodegradable percolates, as well as physicochemical processes for old and little to no biodegradable effluents were used. Overall, the results are satisfactory, but the processes for removing residual Chemical Oxygen Demand (COD) are often costly and unreliable, necessitating the production of other more efficient and less expensive processes [Citation3,Citation4].
The most recent advances in the treatment of wastewaters concern a new range of electrochemical processes, including the electrocoagulation. This technique is very feasible and promising since it achieves high treatment yields with low costs. It consists of pairs of metal often made with aluminum or iron, which are arranged in pairs of anode and cathode [Citation5]. Using the principles of electrochemistry, the anode is oxidized due to an applied current (EquationEquation (1)(1)
(1) ), while the water is reduced at the cathode generating hydroxide ions (EquationEquation (3)
(3)
(3) ) [Citation5]. Metal cations produced at the anode react with hydroxide ions to form mainly neutral amorphous metal hydroxides
(EquationEquation (5)
(5)
(5) ) [Citation5]. These amorphous species, also called ‘flocculation field’, are poorly soluble, and have a large surface area. This is suitable for the rapid adsorption of organic compounds and the trapping of colloidal particles, via electrostatic forces of the Vander-Waals type [Citation6]. This leads to the formation of flocs that will be easily separated from the aqueous medium either by sedimentation or by flotation favored by the gene(at the anode)ration of bubbles of oxygen (O2) (EquationEquation (2)
(2)
(2) ) and hydrogen (H2) (EquationEquations (3)
(3)
(3) and (Equation4
(4)
(4) )) [Citation7].
The main purpose of this study was to treat the landfill leachate by the electrocoagulation process using aluminum electrodes in an electrochemical reactor containing 300 mL of effluent. Doehlert experimental design was applied to determine the optimal operating conditions for the reduction of the chemical oxygen demand and discoloration of leachate.
Experimental design is an efficient method of optimizing the experimental conditions. This procedure is applied to maximize the studied factors number and the obtained useful information with the minimum number of experiments. Moreover, experimental design provides a more efficient and complete optimization compared with the ‘vary one factor at a time’ approach with other factors assigned fixed values [Citation8].
2. Materials and methods
2.1. Characterization of the landfill leachate
The landfill leachate used in this study was collected in a container, closed and stored in obscurity at 4°C. This effluent contained heavy metals, such as Pb (1.8 mg L−1), Fe (10.7 mg L−1), Cu (0.56 mg L−1), Cd (0.003 mg L−1); and was characterized by a conductivity of 39.7 mS/cm, a pH of 8.8, a chemical oxygen demand of 8200 mg L−1, and a total suspended solids of 270 mg L−1. Before treatment, the leachate was diluted ten times.
2.2. Analytical determinations
During the treatment, two parameters were exploited in order to follow the removal of pollutants from the landfill leachate. The chemical oxygen demand (COD) was measured according to the procedure described by the standard method [Citation9] using the HI 839800 COD test tube heater and the HI 83214 COD meter and multi-parameter photometer. The ultraviolet absorbance (UVA) measurements were performed at 390 nm using the Beckman DU 530 UV-Vis spectrophotometer [Citation10].
2.3. Experimental procedure
2.3.1. Electrocoagulation process
The treatment of the landfill leachate by the electrocoagulation process was carried out in a 300 mL glass cell with water jacket. This cell was equipped with six aluminum plates (60 mm × 40 mm × 0.5 mm of 99% purity). Three plates were connected as anodes and three others as cathodes, in a monopolar mode, to a DC power supply (Metrix, model AX 322) operating in galvanostatic mode to control the current intensity. The pH of the effluent was adjusted by sulfuric acid (H2SO4) and sodium hydroxide (NaOH).
2.3.2. Doehlert experimental design
Doehlert experimental design [Citation11]. was used to determine the optimal operating conditions for the treatment of the landfill leachate. The influence of three factors: initial pH (U1), electrolysis time (U2) and current density (U3) were studied. The analyzed responses Y1 and Y2 were the COD removal (%) and the color removal (%), respectively. The Doehlert matrix consists of N experiments with N = K2 + K + 1, where K is the number of variables [Citation9]. For K = 3, the matrix comprised 13 experiments which were uniformly distributed within the space of the coded variables (Xi) [Citation12]. The number of replicates in the central point of the design was fixed at 3 in order to obtain an estimation of the experimental error [Citation12]. The transformation of natural variables (Ui) into coded variables (Xi) was made according to the following equation [Citation13]:
Where Ui (0) is the value of Ui at the center of the study domain, ΔUi is the variation step and αi is the maximum coded value of Xi: α1 = 1; α2 = 0.866; α3 = 0.816 [Citation12].
For the Doehlert experimental design construction, centers and variations steps of the retained domain for each variable () were determined after preliminary tests (data not shown). The experimental response associated to the Doehlert matrix is represented by a quadratic polynomial model:
Table 1. Experimental domain for Doehlert design
Where Y is the experimental response, b0 is a constant of the model, bi is the estimation of the main effects of the factor i, bii is the estimation of the second-order effects and bij is the estimation of the interactions between factor i and factor j [Citation12].
The calculation of coefficients is carried out through the least-squares method by means of:
Where B is the vector of estimates of the coefficients, X is the model matrix, XT is the transposed model matrix and Y is the vector of measured response [Citation12].
The variance analysis (ANOVA) was used to check the model’s statistical significance. The isoresponse curves and the three-dimensional response surfaces were plotted to demonstrate the relationship between the response and the experimental variables. The treatment and calculation of data were made by means of NEMRODW Software [Citation14].
3. Results and discussion
3.1. Experimental design and statistical analysis
The performances of the electrocoagulation process depend on various operating parameters, like pH, electrolysis time and current density. The optimal conditions of these variables, for the removal of COD and discoloration of landfill leachate, have been determined by means of Doehlert matrix. The experimental design is reported in . The obtained responses were used for the calculation of the polynomial model coefficients, leading to propose the following model equations for the COD and color removals (%), respectively..
Table 2. Doehlert experimental design and the obtained responses
In order to verify the reproducibility of the investigated process and to validate the proposed models, the experiment at the domain center was carried out three times and represented in . The obtained results indicated a good reproducibility and the reactive standard deviation (RSD) was less than 3%.
Table 3. Repeated experiments in the center of the investigated domain
The variance analysis was performed on each response separately (). The obtained results showed that the two models (Y1 and Y2) were statistically meaningful (their P-value are less than 0.05) [Citation15]. Moreover, the correlation coefficients (R2) for the responses Y1 and Y2 were, respectively, 0.94 and 0.96. These data reveal that the correlation was good, indicating a good fit of the models, for which at least R2 = 0.80 is suggested [Citation16,Citation17]. Accordingly, one can deduce that each second-order model is appropriate to describe each response and can be used as a prediction equation in the studied domain.
Table 4. ANOVA result for COD and color removal (%) under electrocoagulation treatment
According to the equation (EquationEquation (11)(11)
(11) ) the current density is the most influential factor in the removal of COD, the electrolysis time is the second most influential factor and the initial pH is the third important factor. The interactions between initial pH and electrolysis time, initial pH and current density, and electrolysis time and current density also have a positive effect on leachate treatment by electrocoagulation process, but less influence than the three principal factors.
3.2. Determination of the optimal conditions for the landfill treatment
The isoresponse contour plots of COD and color removal rates, as well as the corresponding three-dimensional (3D) surface plots are represented in .
Figure 1. (a) Contour plots of COD removal versus the electrolysis time (min) and the current density (mA‧cm−2); (b) corresponding 3D surface plot; (c) contour plots of COD removal versus the initial pH and the current density (mA‧cm−2); (d) corresponding 3D surface plot; (e) contour plots of COD removal versus the initial pH and the electrolysis time (min); (f) corresponding 3D surface plot. Results obtained from the Doehlert matrix ()
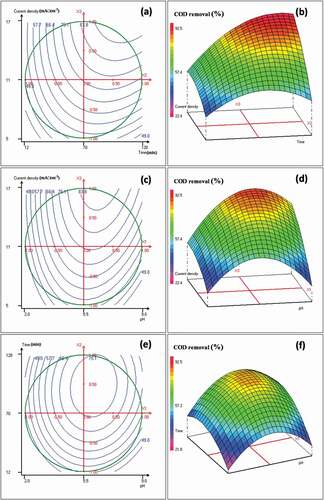
Figure 2. (a) Contour plots of Color removal versus the electrolysis time (min) and the current density (mA‧cm−2); (b) corresponding 3D surface plot; (c) contour plots of Color removal versus the initial pH and the current density (mA‧cm−2); (d) corresponding 3D surface plot; (e) contour plots of Color removal versus the initial pH and the electrolysis time (min); (f) corresponding 3D surface plot. Results obtained from the Doehlert matrix ()
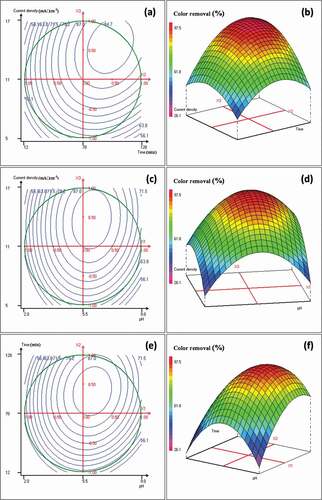
These figures indicated that the electrolysis time was very meaningful for the remediation of the landfill leachate; its effect is positive (). As can be seen an increase of the electrolysis time enhances the COD and color removal rates. Indeed, higher rates of COD and color removal were achieved when the duration of reaction has been varied from 81 to 113 minutes ().
This result can be explained by the continuous generation of Al3+ and hydroxide ions, during the treatment, that would promote aluminum hydroxide (Al (OH)3) production. These amorphous species, also called ‘flocculation field’, have a large surface area suitable for the rapid adsorption of soluble organic compounds and the trapping of colloidal particles, via electrostatic forces of the Vander-Waals type [Citation6,Citation18]. In the case of high anode potential, the oxidation of organic compounds and Cl− present in the leachate, may occur at the anode [Citation19]. The produced chlorine ((EquationEquation (13)(13)
(13) ) is a strong oxidant capable to cause the oxidation of organic compounds or to induce the formation of ClOH ((EquationEquation (14)
(14)
(14) ) which is also an oxidizing agent [Citation19,Citation20].
In addition, an increase of the applied current density increases the treatment efficiency. The maximum COD and color removal rates, in the studied domain, were reached when the current density was between 11 and 17 mA‧cm−2 (). This behavior can be attributed to the generation rate increase of Al3+ cations that would promote aluminum hydroxide production. Besides, the increase of current density, increases bubble density and decreases their size, inducing a greater upwards flux and a faster removal of pollutants and sludge flotation [Citation19]. Indeed, the bubble density affects the system hydrodynamics, which would influence the mass transfer between pollutants, coagulant and gas micro-bubbles, and consequently promotes collision rate of coagulated particles that results in flocs formation [Citation20].
When the applied current density was low, it was observed that the electrolysis time needed to achieve similar efficiencies increased. This result can be attributed to the charge loading (Q = It) that affects the treatment efficiency [Citation21,Citation22]. However, a very high current density may reduce the electrocoagulation efficiency, leading to a decrease of the removal yields. Indeed, the excessive aluminum ions generation can reverse the charge of colloidal particles and re-disperse them, which would decrease the coagulant efficiency and reduce the electrode lifetime [Citation20,Citation23].
Moreover, the initial pH of the landfill leachate is an important factor that influences the electrocoagulation process performance; mainly the coagulation mechanism because it governs the hydrolyzed aluminum species produced in the reactor and affects the electrocoagulation prevailing mechanisms [Citation20]. As illustrated in ), the COD and color removal rates were improved when the initial pH was located between 4 and 8. However, the treatment efficiency was very poor whether at low (<2) or high pH (>8). This behavior is due to the amphoteric character of aluminum hydroxide (Al(OH)3) that does not precipitate at pH less than 2, but leads to soluble Al3+ cations [Citation19,Citation21]. For high initial pH (>8), the solubility of Al(OH)3 species increases, and the formation of soluble Al(OH)4− take place, but these soluble species are useless for leachate treatment [Citation19]. When the initial pH was ranged from 4 to 8, the removal rates of COD and color increased, due to the increase of insoluble aluminum hydroxide amount. Indeed, all aluminum cations produced at the anode formed various polymeric species such as Al6(OH)153+, Al7(OH)174+ and Al13O4(OH)247+, which transformed into Al(OH)3 according to complex precipitation kinetics leading to a more effective treatment [Citation24,Citation25]. show that the highest removal rates were reached at pH values in the range 5–7.
On the other hand, an increase of pH was observed during the electrocoagulation treatment for initial pH values lower than 7. This phenomenon is related to the release of CO2 from leachate owing to H2 bubble disturbance [Citation19]. Indeed, at low pH, CO2 is over saturated in the leachate and can release during the H2 evolution at cathodes, leading to an increase of the pH [Citation26]. However, when the initial pH was higher than 8, the final pH did not vary very much and a slight drop was recorded [Citation26].
The landfill leachate was treated by the electrocoagulation process under the optimal operating conditions determined from the Doehlert matrix. These conditions, namely pHinitial = 6, electrolysis time = 90 min and current density = 15 mA‧cm−2, led to an almost total elimination of the pollutants. Indeed, 94% and 97% of COD and color removals were, respectively, obtained. These results show that electrocoagulation is an effective process for the elimination of toxic pollutants, organic and inorganic, contained in the landfill leachate.
4. Conclusion
Landfill leachate contains all kinds of harmful pollutants, which are known to cause environmental issues as well as serious harm to human health. The present work has shown the efficiency of electrocoagulation process in the treatment of leachate. The effect of important factors such as current density, electrolysis time and initial pH has been studied. The results analysis indicates that all three factors have a positive effect on the leachate remediation as well as their interaction. Moreover, the electrocoagulation treatment was optimized by means of a Doehlert experimental design, showing that 15 mA‧cm−2 current density, 90 min electrolysis time and pH 6 were optimal, leading to an almost total COD and color removal, 94 and 97%, respectively. The obtained quadratic polynomial models were found therefore suitable to be used as prediction equations in the studied domain.
Author contributions
All authors contributed to the work presented in the manuscript.
Acknowledgments
The authors express their sincere thanks to the University of Hail, Kingdom of Saudi Arabia for financing this study. Thanks go also to the Chemistry Department at the Science college of Hail City for their valuable assistance in providing with the required information.
Disclosure statement
No potential conflict of interest was reported by the author(s).
Additional information
Funding
References
- Aziz HA, Yusoff MS, Adlan MN, et al. Physico-chemical removal of iron from semi-aerobic landfill leachate by limestone filter. Waste Manage. 2004;24(4):353–358.
- Rajoo KS, Karam DS, Ismail A, et al. Evaluating the leachate contamination impact of landfills and open dumpsites from developing countries using the proposed Leachate Pollution Index for Developing Countries (LPIDC). Environ Nanotech Monit Manag. 2020;14:100372.
- Shou-liang H, Bei-dou X, Hai-chan Y, et al. In situ simultaneous organics and nitrogen removal from recycled landfill leachate using an anaerobic–aerobic process. Bioresour Technol. 2008;99(14):6456–6463.
- Luna Y, Otal E, Vilches LF, et al. Use of zeolitised coal fly ash for landfill leachate treatment: a pilot plant study. Waste Manage. 2007;27(12):1877–1883.
- Bel Hadj Hmida ES, Mansour D, Bellakhal N. Treatment of lixiviate from Jebel Chakir-Tunis by electrocoagulation. Desalin Water Treat. 2010;24(1–3):266–272.
- Janpoor F, Torabian A, Khatibikamal V. Treatment of laundry waste-water by electrocoagulation. J Chem Technol Biot. 2011;86(8):1113–1120.
- Naje AS, Chelliapan S, Zakaria Z, et al. A review of electrocoagulation technology for the treatment of textile wastewater. Rev Chem Eng. 2017;33(3). DOI:https://doi.org/10.1515/revce-2016-0019
- Poole CF, Poole SK. Comprehensive sampling and sample preparation. Chem Mol Sci Chem Eng. 2012;2:273–297.
- Knechtel RJ. A more economical method for the determination of chemical oxygen demand. Journal of the Water Pollution Control Federation. 1978.
- Singleton VL, Rossi JA. Colorimetry of total phenolics with phosphomolybdic-phosphotungstic acid reagents. Am J Enol Vitic. 1965;16(3):144.
- Doehlert DH. Uniform shell designs. J R Stat Soc Ser C. 1970;19(3):231–239.
- Mansour D, Fourcade F, Soutrel I, et al. Mineralization of synthetic and industrial pharmaceutical effluent containing trimethoprim by combining electro-fenton and activated sludge treatment. J Taiwan Inst Chem Eng. 2015;53:58–67.
- Hammami S, Ouejhani A, Bellakhal N, et al. Application of Doehlert matrix to determine the optimal conditions of electrochemical treatment of tannery effluents. J Hazard Mater. 2009;163(1):251–258.
- Mathieu D, Nony J, Phan-Tan-Luu R. New efficient methodology for research using optimal design (NEMRODW) software. LPRAI, Univ. Aix-Marseille III, France. LPRAI, Univ. Aix-Marseille III, France; 2000.
- Bensalah W, Elleuch K, Feki M, et al. Optimization of mechanical and chemical properties of sulphuric anodized aluminium using statistical experimental methods. Mater Chem Phys. 2008;108(2–3):296–305.
- Torrades F, Using Central G-MJ. Composite experimental design to optimize the degradation of real dye wastewater by fenton and photo-fenton reactions. Dyes Pigm. 2014;100:184–189.
- Arslan-Alaton I, Tureli G, Olmez-Hanci T. Optimization of the photo-fenton-like process for real and synthetic azo dye production wastewater treatment using response surface methodology. Photochem Photobiol Sci. 2009;8(5):628.
- Zidane F, Drogui P, Lekhlif B, et al. Decolourization of dye-containing effluent using mineral coagulants produced by electrocoagulation. J Hazard Mater. 2008;155(1–2):153–163.
- Adhoum N, Monser L. Decolourization and removal of phenolic compounds from olive mill wastewater by electrocoagulation. Chem Eng Process. 2004;43(10):1281–1287.
- Hakizimana JN, Gourich B, Chafi M, et al. Electrocoagulation process in water treatment: a review of electrocoagulation modeling approaches. Desalination. 2017;404:1–21.
- Chakchouk I, Elloumi N, Belaid C, et al. A combined electrocoagulation-electrooxidation treatment for dairy wastewater. Braz Chem Eng J. 2017;34(1):109–117.
- Chen X, Chen G, Yue PL. Separation of pollutants from restaurant wastewater by electrocoagulation. Sep Purif Technol. 2000;19(1–2):65–76.
- Palahouane B, Keffous A, Naceur MW, et al. Electrocoagulation performance of industrial wastewater with high fluoride concentrations and characteristics of the sludge generated. Desalin Water Treat. 2020;183:240–247.
- Khosravi R, Hazrati S, Fazlzadeh M. Decolorization of AR18 dye solution by electrocoagulation: sludge production and electrode loss in different current densities. Desalin Water Treat. 2016;57(31):14656–14664.
- Kobya M, Can OT, Bayramoglu M. Treatment of textile wastewaters by electrocoagulation using iron and aluminum electrodes. J Hazard Mater. 2003;100(1–3):163–178.
- Bazrafshan E, Ownagh KA, Mahvi AH. Application of electrocoagulation process using iron and aluminum electrodes for fluoride removal from aqueous environment. Eur J Adv Chem. 2012;9(4):2297–2308.