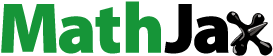
Abstract
The aim of this research was to investigate the phytochemical composition and antimalarial effects of the methanol extract of Erythrina senegalensis leaves (MEES) and its ameliorative effects on some haematological and biochemical alterations in Plasmodium berghei-infected mice. Thirty Swiss mice, 18–22 grams were placed in 6 groups of 5 mice. Group 1 served as normal control. Groups 2–6 were infected with P. berghei. Group 2 was untreated while group 3 was treated with 5 mg/kg body weight of chloroquine. Groups 4–6 were treated orally with 200, 400 and 600 mg/kg b.w. of the MEES for 4 days. The percentage parasitaemia, haematological and biochemical parameters were investigated. Phytochemical analysis indicated the presence of a number of phyto-constituents. The acute toxicity test of the MEES showed no toxicity up to a dose of 5000 mg/kg b. wt. P. berghei infection triggered marked increases in percentage parasitaemia, lipid peroxidation, liver enzymes, kidney function and lipid profile, while the antioxidant and haematological parameters declined in the untreated group. Treatment with the MEES led to significant (p < 0.05) reduction in percentage parasitaemia, lipid peroxidation, liver enzymes, kidney function and lipid profile, and a corresponding increase in antioxidant and haematological parameters, indicating that the MEES reversed complications associated with P. berghei infection.
Introduction
Malaria threatens almost one-third of the world’s population in 104 tropical countries and territories where it is considered an endemic disease (Pimenta et al. Citation2015). Malaria is caused by parasites of the genus Plasmodium and is one of the leading infectious diseases in many tropical regions, including Nigeria, where transmission occurs all year round (Adebayo and Krettli Citation2011). Five Plasmodium species cause malaria in human beings: Plasmodium falciparum, P. vivax, P. ovale, P. malariae and P. knowelsi. The most common and precarious type of malaria is caused by P. falciparum. P. vivax is a common cause of malaria in Latin America, Asia, and Oceania, but not Africa. P. malariae and P. ovale are much less common (Saifi et al. Citation2013). P. falciparum is responsible for the vast majority of deaths from malaria (Lothar et al. Citation2008). This is mainly as a result of two major complications; cerebral malaria and anaemia (Mohapatra Citation2001; Lothar et al. Citation2008). Other symptoms include fever, chills, nausea and flu-like illness. Splenomegaly, severe headache, hepatomegaly, haemoglobinuria with renal failure may occur (Trampuz et al. Citation2003). Severe malaria can progress extremely rapidly and cause coma or even death within hours or days (Trampuz et al. Citation2003). Malaria which is the most prevalent infectious disease in the tropical and subtropical regions of the world is of great public health importance (Mishra and Mohanty Citation2003). The World Health Organization (WHO) reports that malaria, the deadly parasitic disease is responsible for nearly 90% of deaths in Africa (Ogbodo et al. Citation2010). One-fifth of infants’ death in Africa is caused by malaria (World Health Organization [WHO] Citation2010). The WHO estimated that in 2015, there were about 212 million cases of malaria which caused about 429,000 deaths worldwide, with the majority of those affected being children less than 5 years old (WHO Citation2016). These figures rose to 216 million cases in 2016, leading to about 445,000 death cases, most of which occurred in Sub-Saharan Africa and India (WHO Citation2017). In 2018, the WHO estimated that about 3.2 billion people across 91 countries are still at risk of malarial infection (WHO Citation2018).
The onset and progression of malaria are characterised by vast alterations in haematological and biochemical parameters (Bidaki and Dalimi Citation2003; Yasmeen et al. Citation2015). Natural products have played a dominant role in the discovery of leads for the development of drugs to treat human diseases, and this fact anticipates that new antimalarial leads may certainly emerge from tropical plant sources (Batista et al. Citation2009). One of these plant source is Erythrina senegalensis DC., commonly grown in West Africa as an ornamental plant and one of the oldest known African medicinal plant (Tepongning et al. Citation2013). The bark and roots are used against stomach disorders and wounds. In Cameroon and Nigeria, preparations from different parts of E. senegalensis are used orally, in body baths or as fumigations to treat malaria and fevers, dysmenorrhea, pneumonia, cough, onchocerciasis, snake bites, gastrointestinal disorders, inflammation, back ache, nose bleeding, dizziness, jaundice and venereal diseases (Togola et al. Citation2008). The plant is commonly called coral flower and parrot tree (English), echichi (Igbo, South Nigeria), ologun sheshe (Western Nigeria), Murjiya, Showoh or Ankai (Hausa, Tiv and Jukun respectively, Northern Nigeria), and Nté (Mali, West Africa) (Doughari Citation2010). It is commonly known as Acheche in Igala language (North central, Nigeria).
Traditionally, E. senegalensis is used for the treatment of malaria, gastrointestinal disorders, body pain as well as liver diseases (Togola et al. Citation2008). The plant is also used as a diuretic and is indicated in the treatment of asthma, generalised oedema and against abortion (Burkill Citation1985). E. senegalensis is also known to cure urinary bilharzias and eye infections (Togola et al. Citation2005). Some pharmacological studies carried out on the plant shows that the plant possesses a number of pharmacological activities (Nene-Bi et al. Citation2013) some of which include; antibacterial, antifungal and antiplasmodial activities (Saidu et al. Citation2000; Atindehou et al. Citation2004; Koné et al. Citation2011), as well as anti-inflammatory (Koné et al. Citation2011), analgesic and antipyretic activities (Saidu et al. Citation2000). However, despite the studies on the traditional uses of this plant, there is a dearth of scientific information on the antimalarial effects of the leaf extract as well as the biochemical changes associated with malaria treated using this plant; this necessitated the study.
Materials and methods
Plant materials
Fresh leaves of E. senegalensis were collected from Ankpa Local Government Area of Kogi State, Nigeria. The leaves were authenticated by Mr Alfred Ozioko of the Bio-resources Development and Conservation Programme Research Centre (BDCP), University of Nigeria, Nsukka, Enugu State, Nigeria. The leaves were air-dried and pulverised into powdered form. The identity and authenticity of the plant was also confirmed with the one deposited in http://www.theplantlist.org/ and http://www.ipni.org/ databases (Figure ).
Animals
A total of thirty (30) Swiss mice weighing between 18 and 22 g were used for the study. They were purchased from the Animal House of the Faculty of Veterinary Medicine, University of Nigeria, Nsukka, and acclimatised for one week prior to the commencement of the experiment, with 12-h light/dark cycle maintained. The animals were placed on regular feed (commercial grower’s mash) and potable drinking water ad libitum, and were handled in accordance with the national and international ethical recommendations for care and use of laboratory animals (National Academy of Sciences [NAS] Citation2011).
Chemicals and reagents
All chemicals and reagents used for this study were of analytical grade and products of Sigma Aldrich, USA; British Drug House (BDH) England; Burgoyne, India; Harkin and Williams, England; Qualikems India; Fluka, Germany; May and Baker, England. Reagents used for the assays were commercial kits and products of Randox and Teco diagnostics (TC), USA. The standard antimalarial drug used in this study was chloroquine procured from a reputable pharmaceutical and drug store in Nsukka, Nigeria.
Plant extraction
Fresh but mature leaves of E. senegalensis were procured, destalked, cleaned and shade-dried for several weeks with regular turning until crispy. The dried leaves were pulverised into coarse form using a mechanical grinder. A known weight, 800 g, of the pulverized leaves was macerated in 3000 ml of methanol for 72 h. The mixture was filtered into a flat-bottomed flask using a muslin cloth. Further filtration was achieved with Whatman No. 1 filter paper so as to remove the fine and suspended residue. The filtrate was concentrated using a rotary evaporator at 40°C to obtain crude methanol extract, subsequently called the methanol extract of E. senegalensis leaves (MEES).
Percentage yield of the MEES
The percentage yield of the MEES was determined from the weight of the dried pulverised leaves before maceration, and the weight of the crude extracts after concentration using the formula below:
Phytochemical analysis of the MEES
The phytochemical analysis of MEES was determined in order to confirm the presence of plant secondary metabolites in the MEES. This followed the protocol of Trease and Evans (Citation1989), and Harborne (Citation1998).
Acute toxicity study of the MEES
The acute toxicity study or median lethal dose (LD50) of the MEES was carried out by the method of Lorke (Citation1983).
Parasite inoculation and determination of percentage parasitaemia
The rodent malaria parasite (P. berghei ANKA), mouse model was employed for this study. The determination of malaria parasitaemia (Mp+) was carried out according to the method described by Dacie and Lewis (Citation2011). Donor mouse blood infected with P. berghei (chloroquine sensitive ANKA-65 strain) was obtained from the Faculty of Veterinary Medicine, University of Nigeria, Nsukka, Enugu State, Nigeria. The mice were infected by obtaining parasitised blood from cut-tip of the tail of infected mice (3–4 drops) and diluted in 0.9 ml of phosphate buffer (pH 7.4). They were inoculated via intraperitoneal route with 0.2 ml P. berghei parasitised red blood cells (PRBCs) maintained by passage and were considered to have cerebral malaria (CM) if they displayed neurological symptoms such as paralysis, deviation of the head, ataxia, convulsions or coma upon infection. The percentage of parasitaemia was then determined by blood film (smears) made by collecting blood from cut-tip of the tail of the mice on days 0 and 3, Giemsa stained and calculated as the number of parasitised red blood cells (pRBC) per 100 RBC (Ekpo and Ekanemesang Citation2016).
Experimental design: a curative study
Following successful parasite inoculation, a total of thirty (30) Swiss mice (18–22 grams) were randomly selected and distributed into 6 groups of 5 mice each, and they received treatment as follows:
Group 1: Normal control (received normal saline orally)
Group 2: Negative control (infected with P. beghei and untreated)
Group 3: Standard control (P. beghei-infected and treated with 5 mg/kg body weight of Chloroquine)
Group 4: P. beghei-infected + 200 mg/kg body weight MEES
Group 5: P. beghei-infected + 400 mg/kg body weight MEES
Group 6: P. beghei-infected + 600 mg/kg body weight MEES
Before treatment, mice in groups 2–6 were inoculated with P. beghei via intraperitoneal route with 0.2 ml of P. berghei parasitised erythrocytes and were left to incubate. Malarial infection was confirmed after 72 h according to Dacie and Lewis (Citation2011). Treatment lasted for 4 days (Peters et al. Citation1975) and the animals were sacrificed on day 5. Blood samples were collected with the aid of a capillary tube from the ocular vein for haematological and biochemical analyses.
Determination of haematological and biochemical indices
The total red blood cell (RBC) count, total white blood cell (WBC) count, packed cell volume (PCV) and haemoglobin (Hb) concentrations were determined by the method of Ochei and Kolhatkar (Citation2008). Lipid peroxidation was determined using spectrophotometric method, by measuring the level of the lipid peroxidation product, malondialdehyde (MDA), a method of Wallin et al. (Citation1993). Glutathione (GSH) concentration was determined by the method of King and Wootton (Citation1959). Total cholesterol concentration was determined by the method of Abell et al. (Citation1952). The concentration of low-density lipoprotein (LDL) was determined using the method of Arsman et al. (Citation1984). High-density lipoprotein (HDL) concentration was determined by the method of Kameswara et al. (Citation1999). The concentration of triacylglycerol (TAG) was determined using the method of Otvos (Citation1999). Alkaline phosphatase (ALP) activity was assayed by the method of Englehardt (Citation1970). The activities of alanine aminotransferase (ALT) and aspartate aminotransferase (AST) were assayed using the method of Reitman and Frankel (Citation1957). Total bilirubin concentration was determined by the method of Jendrassik and Grof (Citation1938) as outlined in the Randox Kit. Serum urea and creatinine concentrations were determined using the method of Bartels and Bohmer (Citation1972).
Assay for serum catalase activity
Serum catalase (CAT) activities were assayed according to the method of Aebi (Citation1983). The principle relies on the ultraviolet absorption of hydrogen peroxide (H2O2) at 240 nm. Upon catalytic decomposition of H2O2 by catalase in the sample, the absorption of the sample decreases with time and from this decline, the catalase activity is monitored. Phosphate buffer (pH 7.0), 0.2 M Hydrogen peroxide, 5% potassium dichromate and 5% glacial acetic acid were used for the assay. The procedure involves pipetting 2.5 ml of phosphate buffer into replicate set of test tubes, followed by addition of 2.0 ml of H2O2 and 0.5 ml of the serum. The mixture was gently shaken to allow proper mixture of all constituents, after which, 2.0 ml of dichromate acetic acid reagent was added to 1.0 ml portion of the reaction mixture. The absorbance of the sample was read at 240 nm at an interval of 1 min against the corresponding reagent blank. Where 0.23 and 0.00693 are constants, the serum catalase activity was determined using the equation given below:
Assay for superoxide dismutase activity
The activity of superoxide dismutase (SOD) in serum was assayed according to the method of Misra and Fridovich (Citation1972). SOD reduces superoxide radical to H2O2. The principle of this indirect method is based on the inhibition of epinephrine auto-oxidation to an adrenochrome in an alkaline environment. The auto-oxidation of epinephrine was initiated by the addition of 1.0 ml of the Fenton reagent (a solution of H2O2 and FeSO4) to a 4 ml mixture of 0.3 mM epinephrine, 1 mM Na2CO3 solution, 0.3 mM ethylenediaminetetraacetic acid (EDTA), and 1 ml of distilled water bringing the final volume to 6 ml. the autoxidation was then monitored every 30 sec for 5 min at 480 nm using a spectrophotometer. The assay was repeated with 1.0 ml of the serum from different blood samples collected from mice drawn from different experimental groups. A plot of absorbance against time was generated for each set of data obtained, and the initial rate of auto-oxidation calculated. One unit of SOD activity was defined as the concentration of the enzyme (IU/L) in the sample that caused 50% reduction in the auto-oxidation of epinephrine (Jewett and Rocklin Citation1993). The SOD activity was subsequently calculated for each sample.
Statistical analysis
The data obtained were analysed using the IBM Statistical Product and Service Solutions (SPSS), version 21. Tests of statistical significance were carried out using the one-way analysis of variance (ANOVA) and the students t-test. The results were expressed as mean ± standard deviation. p values < 0.05 were considered statistically significant. The t-test was carried out on the percentage parasitaemia of mice infected with P. berghei.
Results
Eight hundred grams (800 g) of the powdered E. senegalensis leaves extracted with 80% methanol yielded 19.34 g of the methanol extract which constituted 2.42% of the total weight of dried plant sample used. Qualitative and quantitative phytochemical analysis of the methanol extract of E. senegalensis leaves indicated presence of relative quantities of steroids, saponins, tannins, resins and acidic compounds. Alkaloids, terpenoids, protein and carbohydrates were present at moderate amount while flavonoids and phenolic compounds were present in high quantities (Table ).
Table 1. Phytochemical composition of the MEES.
Acute toxicity of the MEES
The acute toxicity test of the MEES showed no mortality or adverse reaction within the test population at a dose range of 10–5000 mg/kg body weight. This showed the relative safety or non-toxic nature of the leaf extract.
Effect of the MEES on percentage parasitaemia of P. berghei-infected mice
Table shows the percentage parasitaemia of mice treated with the MEES. Baseline percentage parasitaemia of the groups infected with P. berghei (Groups 2–6) were found to be significantly (p < 0.05) higher when compared to the normal control. There was no significant (p > 0.05) difference between the groups infected with P. berghei when compared with one another. Following treatment with graded doses of the MEES, the percentage parasitaemia of the treated groups were found to be significantly (p < 0.05) lower when compared to the negative control (untreated group).
Table 2. Effect of the MEES on percentage parasitaemia of P. berghei-infected mice.
Effect of the MEES on haematological profile of P. berghei-infected mice
Table shows the haematological profile of mice treated with MEES. The RBC count, Hb and PCV concentrations of the negative control (parasitised-untreated group) were observed to be significantly (p < 0.05) lower when compared to the normal control. Treatment with increasing doses of the MEES indicated a significant (p < 0.05) increase in the RBC count which is comparable to that of the normal control. The WBC count of the negative control was observed to be significantly (p < 0.05) higher when compared to the normal control. However, treatment with graded doses of the MEES recorded a significantly (p < 0.05) lower WBC count when compared to the untreated group. There was no significant (p > 0.05) difference in the RBC, WBC, Hb and PCV of the treated groups when compared with one another.
Table 3. Effect of the MEES on haematological profile of P. berghei-infected mice.
Effects of the MEES on liver functions of P. berghei-infected mice
Table showed the total bilirubin concentration and activities of AST, ALT, and ALP of the negative control (untreated group) to be significantly (p < 0.05) higher when compared with the normal control. However, treatment with graded doses of the MEES indicated significant (p < 0.05) decreases in total bilirubin concentration, AST, ALT, and ALP activities when compared to the negative control. There was no significant (p > 0.05) difference in the total bilirubin concentration, AST, ALT, ALP activities of the MEES treated groups and standard control when compared with one another.
Table 4. Effect of the MEES on the activities of liver enzymes P. berghei-infected mice.
Effect of the MEES on lipid peroxidation and antioxidant indices of P. berghei-infected mice
The MDA concentration of the negative control was observed to be significantly (p < 0.05) higher when compared to the normal control and the MEES treated groups. Treatment with the MEES at 200, 400 and 600 mg/kg b.w. respectively led to a significant (p < 0.05) decrease in MDA concentration which is comparable to that of the normal control. There was no significant (p > 0.05) difference in the SOD activity of the negative control when compared to the normal control and the treated groups. The activity of CAT and the GSH concentration of the negative control was observed to be significantly (p < 0.05) lower when compared to the normal control. Treatment with graded doses of the MEES led to significant (p < 0.05) increases in CAT activities and GSH concentration respectively (Table ).
Table 5. Effect of the MEES on lipid peroxidation and antioxidant indices of P. berghei-infected mice.
Effect of the MEES on kidney function parameters of P. berghei-infected mice
The urea and creatinine concentrations of the negative control (P. berghei-infected and untreated) were observed to be significantly (p < 0.05) higher when compared to the normal control. However, treatment with graded doses of the MEES led to significant (p < 0.05) decrease in the urea and creatinine concentrations (Table ).
Table 6. Effect of the MEES on urea and creatinine concentrations of P. berghei-infected mice.
Effect of the MEES on lipid profile of P. berghei-infected mice
Table shows the lipid profile of infected mice treated with the MEES. The TAG, total cholesterol and LDL concentrations of the negative control were observed to be significantly (p < 0.05) higher when compared to the normal control. Treatment with increasing doses of the MEES indicated a significantly (p < 0.05) higher TAG, total cholesterol and LDL concentrations which is comparable to the normal control. The effect of the MEES leaves on HDL concentration showed a significantly (p < 0.05) lower HDL concentration of the negative control when compared to the normal control. However, the groups treated with grade doses of the MEES recorded significantly (p < 0.05) higher HDL concentration when compared to the negative control. There was no significant (p > 0.05) difference in the HDL concentration of the treated groups when compared with one another.
Table 7. Effect of the MEES on lipid profile of P. berghei-infected mice.
Discussion
The preliminary qualitative and quantitative analyses of the MEES reveal the presence of phytoconstituents such as steroids, saponins, tannins, resins and acidic compound in relative quantities while alkaloids, terpenoids, and proteins were present at moderate level. Flavonoids and phenols were present in high quantities while glycosides were not detected. This is consistent with the report of Nnama et al. (Citation2017), and Osuntokun et al. (Citation2016), who reported that the leaf extract of E. senegalensis contains phytochemicals such as saponins, flavonoids, tannins, terpenoids, glycosides and alkaloids. Alkaloids have been shown to have many pharmacological activities including; antihypertensive effects (indole alkaloid), antiarrhythmic effect, antimalarial activity (quinine), anticancer activity (Togola et al. Citation2008; Mamta et al. Citation2013) and wound healing property (Ilodigwe Citation2014). Koné et al. (Citation2011) reported that Erythrina spp. are used to treat frequent parasitic, microbial diseases/infections, cancer and wounds. This could be ascribed to the presence of flavonoids and alkaloids in the plant. Flavonoids have been reported to exert multiple biological properties including; antimicrobial, anti-inflammatory as well as antitumor activities but the best described property of almost every group of flavonoids is their capacity to act as powerful antioxidants which can protect the human body from free radicals and reactive oxygen species (ROS) (Mamta et al. Citation2013). Terpenoids have been reported by Nita et al. (Citation2014) to play a role in traditional herbal remedy. Doughari (Citation2010) reported that the stem bark extract of E. senegalensis demonstrated antimicrobial activities against bacteria and fungi. The presence of steroids could be responsible for the anti-inflammatory effect of the plant as reported by Togola et al. (Citation2008). The presence of phenolics also relates to the antioxidant role of the stem bark of the E. senegalensis as reported by Atsamo et al. (Citation2011) and as such could be used for the treatment of cardiovascular diseases. Many saponins are known to be antimicrobials, and this could be ascribed to the antimalarial potentials of the stem bark of the extract as reported by Doughari (Citation2010).
The acute toxicity (LD50) test of the methanol extract of E. senegalensis leaves showed that the MEES did not cause any physical sign of toxicity, behavioural changes or mortality. This indicates that the plant is safe for consumption and for medicinal purposes. Following malaria parasite infection, we observed a significant (p < 0.05) increase in the percentage parasitaemia count of the untrated group when compared to the normal control. Treatment with increasing doses of the MEES led to a significant (p < 0.05) reduction in the percentage parasitaemia count of the treated mice groups when compared to the untreated group. This finding agrees with the report that E. senegalensis can be used traditionally to treat malaria (Togola et al. Citation2008; Tepongning et al. Citation2013). The antimalarial activity of the MEES leaves extract could be attributed to the presence of bioactive compounds such as alkaloids, saponins and terpenoids in the plant leaves. Mamta et al. (Citation2013) in his report revealed that alkaloids have antimalarial activities. The haematological parameters reveal that the MEES significantly (p < 0.05) increased the RBC, PCV and Hb levels in the treated groups relative to the untreated group. The WBC concentration was found to be significantly (p < 0.05) lower when compared to the untreated mice. This is in contrast with the study of Obidah et al. (Citation2014), who reported that the leaf extract of E. sengalensis has no effect on the haematological parameters of P. berghei-infected mice. This could be accrued to the differences in the dosages administered. A low RBC and PCV is an indication of anaemia, blood loss, liver cirrhosis, vitamin or mineral deficiency (Cheesbrough Citation2006). The increased PCV and RBC counts observed in our study showed that the MEES could be anti-anaemic or may be an indication of improvement of bone marrow function. According to Omodeo-Salè et al. (Citation2003) , oxidative changes in P. falciparium infected red blood cells seem to be associated with the accelerated ageing of these cells and contribute to the development of anaemia. Haemoglobin is the iron containing oxygen-transport metalloprotein in RBCs of all vertebrates. Iron is essential in haemoglobin synthesis as it is needed to produce the haem component of haemoglobin (Cheesbrough Citation2006). Osuntokun et al. (Citation2016) reported the presence of Iron (Fe) and other minerals in the leaves of E. senegalensis. Insufficient iron for haemoglobin production often leads to anaemia and consequently, a low RBC production (Cheesbrough Citation2006). The increase in the haemoglobin concentration (p < 0.05) of the treated groups noticed in this study, when compared to the untreated group could be attributed to the recovery of the treated mice due to the presence of iron in the E. senegalensis leaves. The reduction in the haemoglobin concentration of the untreated mice group could be attributed to its depletion owing to the fact that the parasite relies on haemoglobin for its food as it generates amino acids on degradation of haemoglobin (Percário et al. Citation2012). White blood cells (WBCs) are important as they determine the body’s ability to defend its host against foreign bodies or infections. In this study, we observed a significant (p < 0.05) decrease in WBC levels in the MEES treated groups relative to the untreated group. This shows that the MEES could be useful in maintaining immune functions.
Liver enzymes are commonly used in the evaluation of liver function. In this study, malaria infected mice (untreated group) recorded marked elevation in the levels of serum AST, ALT and ALP which is indicative of hepatocellular damage. This might possibly be due to the release of these enzymes from the cytoplasm into the blood circulation following loss of the plasma membrane integrity and cellular damage arising from oxidative state caused by P. berghei. Oral administration of the MEES for 4 consecutive days significantly (p < 0.05) lowered the activities of these liver enzymes in the treated groups. This result agrees with the report of Donfack et al. (Citation2008), who reported that the stem bark extract of E. senegalensis reduced serum levels of AST and ALT in CCl4-induced hepatitis in rat liver. This indicates that the MEES could protect against liver damage by maintaining the structural integrity of the plasma membrane thereby suppressing the leakage of the enzymes into the circulation, thus, exhibiting hepatoprotective effects. A number of scientific reports indicate that certain flavonoids, terpenoids and steroids have protective effects on the liver due to their antioxidant properties (Jeruto et al. Citation2011). Phytochemically, the MEES contain these phyto-constituents and may have played active roles in the hepatoprotective activity we noticed in this study. The increase in bilirubin concentration of the parasitised-untreated group is an index of jaundice, a condition of liver injury, possibly due to increased production or decreased uptake by the liver, decreased conjugation and decreased secretion from the liver or blockage of bile duct. This increase in bilirubin concentration was observed in the untreated group after inoculation with the parasitised RBCs. We noticed a significant (p < 0.05) decrease in total bilirubin concentratin of the treated groups at the highest dose administered when compared to the untreated group, indicating the potential of the MEES in curbing diseases associated with overproduction of bilirubin.
The accumulation of lipid peroxidation product in human tissues is a major cause of tissular and cellular dysfunction, and plays a major role in oxidative stress-related diseases such as malaria (Anne et al. Citation2010). Malondialdehyde (MDA) is a reliable and commonly used marker of lipid peroxidation levels, and quantifying the presence of oxidative stress in biological systems (Kayar et al. Citation2015). From the result obtained in our study, the MDA concentration of the treated groups significantly (p < 0.05) decreased when compared to the untreated group. The reduction in MDA level observed in this study shows that the MEES was able to inhibit lipid peroxidation in the treated group, thus, giving credence to its antioxidant capacity. The increase in lipid peroxidation levels during malaria as observed in the present study may be due to the inefficient antioxidant system prevalent during malaria parasite infection. Treatment with graded doses of the MEES markedly reduced the lipid peroxidation level of the treated groups when compared to the untreated mice group. Thus, diseases associated with oxidative stress such as cancer, arthritis, cardiovascular diseases among others can be circumvented with the MEES. Superoxide dismutase is an antioxidant enzyme that protects the cells from superoxide radical (O2−). Under the action of SOD, O2− is transformed into H2O2 and hydroxyl radical (McCord and Fridovich Citation1988). The reduction in the level of SOD in the untreated group could be attributed to its role in combating the free radicals generated during the parasite infection. Treatment with the MEES led to a significant (p < 0.05) increase in the SOD activity at the highest dose (600 mg/kg b.w). Catalase is an essential enzyme in the decomposition of intracellular H2O2). This study revealed a significant (p < 0.05) increase in CAT activities of the treated groups when compared to that of the untreated group. The increase in the CAT activities noticed in the treated groups shows that the MEES was able to restore the level of CAT utilised in decomposing H2O2. Improvements in the activities of the antioxidant enzymes could be related to the ability of the MEES to scavenge ROS generated by parasite inoculation, due to high presence of phenols and flavonoids in the MEES, thus preventing further damage to membrane lipids. Glutathione is one of the most important antioxidants that play a vital role in maintaining the redox state of the cell. The increase in GSH concentration of the treated groups relative to the untreated group shows the ability of the MEES to boosts the endogenous antioxidant defense system of the host organism thereby protecting against parasite infection.
Serum urea and creatinine concentrations are kidney markers used to test the functionality of the kidney in a diseased state. Urea is a by-product of protein metabolism, usually excreted through the urine, while creatinine is a product of creatine phosphate, produced in the body in proportion to body mass. In the present study, the elevated levels of urea and creatinine in the serum of the parasitised-untreated mice signifies a possible impaired kidney function (Perrone et al. Citation1992). The reduction in urea and creatinine levels of the treated groups when compared to the untreated group reveal the ability of the MEES to scavenge initiators of lipid peroxidation, so as to maintain the structural integrity of the kidney membrane, thus, leading to improved kidney function.
A standard lipid profile includes measurement of plasma or serum concentrations of total cholesterol, LDL, HDL and TAG (Børge et al. Citation2016). Cholesterol is a lipid produced by the liver and is vital for the normal functioning of the body. However, when it is produced in excess, it may cause cardiovascular complications such as arteriosclerosis, resulting in myocardial infarction (Kurian and Perumal Citation2013). In the present study, infection of the mice with malaria parasite caused a significant (p < 0.05) decrease in the HDL concentration of the untreated group and a corresponding increase in LDL, TAG and total cholesterol concentrations when compared with the normal control. This finding is consistent with the work of Enechi et al. (Citation2019) who also reported a significant (p < 0.05) decline in HDL concentration and a significantly (p < 0.05) higher concentration of LDL, TAG and total cholesterol in the parasitised-untreated mice group, following malaria parasite infection. A study conducted by Kiru et al. (Citation2018), showed that malaria infected human subjects recorded higher LDL, TAG, HDL, very low-density lipoprotein (VLDL), and total cholesterol levels when compared with the normal control. The decrease in HDL level, and the corresponding increase in LDL, TAG and total cholesterol concentrations following malaria parasite infection in the mice could be as a result of the increase in the parasitaemia of the untreated group (Akanbi Citation2013). It has been reported that the level of parasitaemia could determine the extent of changes in lipid profiles in malaria positive patients (Akanbi et al. Citation2012).
Also, the use of cholesterol and phospholipids by malaria parasites was hypothesized as a reason for the decrease in the level of serum HDL concentration following malaria parasite infection (Adamu and Jigam Citation2019). Changes in serum lipid profile during malaria infection and the underlying biological mechanisms remain unclear (Visser et al. Citation2013). The RBC membrane is made up of a complex mixture of lipids and proteins with cholesterol and distinct phospholipids making the core of the membrane which are arranged in a lipid bilayer (Kuypers Citation2007). During malaria parasite infection, the rupture of the RBCs can result in the elevation of plasma lipid levels (Adamu and Jigam Citation2019). However, treatment of the parasitised mice with graded doses of the MEES significantly (p < 0.05) increased the HDL concentration when compared with the parasitised-untreated mice group. On the other hand, treatment of the parasitised mice with the MEES significantly (p < 0.05) decreased the LDL, TAG and total cholesterol concentrations of the infected and treated mice groups when compared with untreated mice group. This finding agrees with the study of Enechi et al. (Citation2019), who also reported treatment-related increase in HDL concentration and declines in LDL, TAG and total cholesterol concentrations of the parasitised-untreated mice group. Many observational studies have confirmed an inverse relationship between HDL and cardiovascular risk in people irrespective of sex, race or ethnicity (Toth et al. Citation2013). Thus, the increase in HDL level and subsequent declines in LDL, TAG and total cholesterol level of the treated mice groups when compared to the untreated group shows that the MEES leaves could serve as a good therapeutic agent against cardiovascular diseases. This agrees with work of Atsamo et al. (Citation2011), who reported that the methanol extract of the stem bark of E. senegalensis possess beneficial effects for the treatment of cardiovascular ailments.
A study conducted by Visser et al. (Citation2013) indicated that changes in serum lipids are a characteristic features of malaria. Increase in cholesterol levels, decrease in HDL level, and elevation in LDL level, which are accompanied by increased levels of TAGs and VLDLs are common biochemical changes noticed in patients with malaria. These lipid abnormalities are transient and occur in the most prevalent species of Plasmodium as well as in complicated or non-complicated cases. This is because lipids play a crucial role in the metabolism of Plasmodium sp., in both phases of its life cycle (Visser et al. Citation2013). The actual mechanisms behind the observed dyslipidaemia in malaria may be partly related to an acute phase response to parasite invasion by the host (Rosenson et al. Citation2007), parasite related (Hanscheid et al. Citation2007) or a combination of both mechanisms. In this study, we observed that malaria infection induced dyslipidaemia which was normalised by daily oral administration of the MEES. This suggests that the MEES could be useful in the development of pharmacological agents for the treatment of malaria and its associated complications.
Conclusion
The methanol extract of E. senegalensis leaves have been shown to be useful in the treatment of malaria in the mouse model studied. This gives scientific evidence to claims in different parts of the country, that the plant could be used in the management and treatment of malarial infection. The study also showed that the extract exhibited hepatoprotective properties by normalising the hepatic marker enzyme activities and antioxidant levels by restoring the antioxidant imbalance. The MEES also improved haematological profile, kidney function as well as lipid profile of P. berghei-parasitised mice. This shows that the MEES possess potent antiplasmodial effect and may, therefore, serve as an alternative source for the development of a safe, effective and affordable antimalarial drug.
Disclosure statement
The authors declare no potential conflict of interest(s) in this publication.
Correction Statement
This article has been corrected with minor changes. These changes do not impact the academic content of the article.
References
- Abell LL, Levey BB, Brodie BB, Kendall FE. 1952. Extraction of cholesterol. J Biol Chem. 195(1):357–366.
- Adamu J, Jigam AA. 2019. Effects of malaria infection on some haematological and biochemical parameters in the general population and pregnant malaria patients attending two district hospitals in Niger State, Nigeria. Glob J Infect Dis Clin Res. 5(1):001–005. doi: https://doi.org/10.17352/gjidcr.000021
- Adebayo JO, Krettli AU. 2011. Potential antimalarials from Nigerian plants: a review. J Ethnopharmacol. 133:289–302. doi: https://doi.org/10.1016/j.jep.2010.11.024
- Aebi HE. 1983. Catalase. In: Bergmeyer HU, editor. Methods of enzymatic analysis. Weinhem: Verlag Chemie; p. 273–286.
- Akanbi OM. 2013. Effect of malaria infection on oxidative stress and lipid profile in pregnant women. J Med Med Sci. 4(3):128–133.
- Akanbi OM, Omonkhua AA, Cyril-Olutayo CM, Fasimoye RY. 2012. The antiplasmodial activity of Anogeissus leiocarpus and its effect on oxidative stress and lipid profile in mice infected with Plasmodium bergheii. Parasitol Res. 110:219–226. doi: https://doi.org/10.1007/s00436-011-2472-7
- Anne NS, Auge N, Ayala V, Basaga H, Boada J, Brenke R, Chapple S, Cohen G, Feher J, Grune T, et al. 2010. Pathological aspects of lipid peroxidation. Free Rad Res. 44(10):1125–1171. doi: https://doi.org/10.3109/10715762.2010.498478
- Arsman G, Jabs HU, Kuhnert U, Nolte W, Schriewer H. 1984. LDL-cholesterol, polyvinyl sulphate method. Clin Chem Acta. 140:77–83. doi: https://doi.org/10.1016/0009-8981(84)90153-0
- Atindehou KK, Schmid C, Brun R, Koné MW, Traore D. 2004. Antitrypanosomal and antiplasmodial activity of medicinal plants from Côte d’Ivoire. J Ethnopharmacol. 90:221–227. doi: https://doi.org/10.1016/j.jep.2003.09.032
- Atsamo AD, Nguelefack TB, Datté JY, Kamanyi A. 2011. Acute and sub-chronic oral toxicity assessment of the aqueous extract from the stem bark of Erythrina senegalensis DC (Fabaceae) in rodents. J Ethnopharmacol. 134(3):697–702. doi: https://doi.org/10.1016/j.jep.2011.01.023
- Bartels H, Bohmer M. 1972. In vitro determination of creatinine and urea. Clin Chem. 2:37–193.
- Batista R, Jesus AD, Júnior S, Oliveira ABD. 2009. Plant-derived antimalarial agents: new leads and efficient phytomedicines. Part II. Non-alkaloidal natural products. Molecules. 14(8):3037–3072. doi: https://doi.org/10.3390/molecules14083037
- Bidaki ZM, Dalimi AA. 2003. Biochemical and haematological alteration in vivax malaria in Kahnouj city. J Rafsanjan Univ Med Sci. 3:17–24.
- Børge GN, Anne L, Samia M, Genovefa K, Hannsjorg B, Eric B, Gerald FW, Grazyna S, Olov W, Jan B, et al. 2016. Fasting is not routinely required for determination of a lipid profile: clinical and laboratory implications including flagging at desirable concentration cut-points. Eur Hrt J Adv. 37(25):1944–1958.
- Burkill HM. 1985. The useful plants of West Tropical Africa. 2nd ed. Vol. 3, Families A–D. Kew: Royal Botanic Gardens.
- Cheesbrough M. 2006. District laboratory practice in tropical countries. Cambridge: Cambridge University Press; p. 62.
- Dacie JV, Lewis SM. 2011. Practical haematology. 11th ed. London: Churchill Livingstone; p. 57–67.
- Donfack JH, Njayou FN, Rodrigue TK, Chuisseu DDP, Tchana NA, Vita FP, Tchouanguep MF, Ngadjui TB, Moundipa FP. 2008. Study of a hepatoprotective and antioxidant fraction from Erythrina senegalensis stem bark extract: in vitro and in vivo. Pharm Online. 1:120–130.
- Doughari JH. 2010. Evaluation of antimicrobial potentials of stem bark extracts of Erythrina senegalensis DC. Afr J Microbiol Res. 4(17):1836–1841.
- Ekpo DE, Ekanemesang UM. 2016. Antiplasmodial/antimalarial effect of ethanol extracts of leaves of Vernonia amygdalina and Gongronema latifolium on the activity of catalase in Plasmodium berghei-parasitized mice. Int J Biochem Res Rev. 10(4):1–9.
- Enechi OC, Amah CC, Okagu IU, Ononiwu CP, Azidiegwu VC, Ugwuoke EO, Onoh AP, Ndukwe EE. 2019. Methanol extracts of Fagara zanthoxyloides leaves possess antimalarial effects and normalizes haematological and biochemical status of Plasmodium berghei-passaged mice. Pharm Biol. 57(1):577–585. doi: https://doi.org/10.1080/13880209.2019.1656753
- Englehardt A. 1970. Measurement of alkaline phosphatase. Aerztl Lab. 16:42–43.
- Hanscheid T, Egan TJ, Grobusch MP. 2007. Haemozoin: from melatonin pigment to drug target, diagnostic tool, and immune modulator. Lancet Infect Dis. 7(10):675–685. doi: https://doi.org/10.1016/S1473-3099(07)70238-4
- Harborne JB. 1998. Textbook of phytochemical method. 3rd ed. London: Chapman and Hall Ltd.
- Ilodigwe EE, Okonkwo BE, Agbata CA, Ajaghaku DL, Eze PM. 2014. Wound healing activity of ethanol leaf extract of Erythrina senegalensis. Br J Pharm Res. 4(4):531–540. doi: https://doi.org/10.9734/BJPR/2014/7260
- Jendrassik L, Grof P. 1938. In vitro determination of total and direct bilirubin. Biochemica. 297:81.
- Jeruto P, Mutai C, Catherine L, Ouma G. 2011. Phytochemical constituents of some medicinal plants used by the Nandis of South Nandi district, Kenya. J Anim Plant Sci. 9(3):1201–1210.
- Jewett SL, Rocklin AM. 1993. Variation in one unit of activity with oxidation rate of organic substrate in indirect superoxide dismutase assays. Ann Rev Biochem. 212:55–59.
- Kameswara RB, Kesavulu MM, Giri CH. 1999. Anti-diabetic and hypolipidemic effects of Momordica cymbalania hook fruit powder in alloxan-induced diabetic rats. J Ethnopharmacol. 67:103–109. doi: https://doi.org/10.1016/S0378-8741(99)00004-5
- Kayar A, Dokuzeylul B, Kandemir FM, Kirbas A, Bayrakal A, Or ME. 2015. Total oxidant and antioxidant capacities, nitric oxide and malondialdehyde levels in cats seropositive for the feline coronavirus. Vet Med. 60(5):274–281. doi: https://doi.org/10.17221/8180-VETMED
- King EJ, Wootton IDP. 1959. Microanalysis in medical biochemistry. 3rd ed. London: Churchill; p. 14.
- Kiru AI, Bala RK, Abdulazeez AM, Bello SY, Adam AL, Suleiman SM, Shamsu A, Abdulkadir ML. 2018. Lipid profile and electrolyte level in malaria patients attending Muhammadu Abdullahi Wuse Specialist Hospital, Kano State. JOCAMR. 5(4):1–7. doi: https://doi.org/10.9734/JOCAMR/2018/41215
- Koné WM, Kakou Ngazoa ES, Dosso M. 2011. Assessing sub-saharan Erythrina for efficacy: traditional uses, biological activities and phytochemistry. Pak J Biol Sci. 14(10):560–571. doi: https://doi.org/10.3923/pjbs.2011.560.571
- Kurian J, Perumal J. 2013. Nature’s remedies made simple. Bangkok: Sirivatana Interprint Public Company Limited; p. 9–11.
- Kuypers FA. 2007. Membrane lipid alterations in hemoglobinopathies. Hematol Am Soc Hematol Educ Prog. 2007(1):68–73. doi: https://doi.org/10.1182/asheducation-2007.1.68
- Lorke D. 1983. A new approach to practical acute toxicity testing. Arch Toxicol. 55:275–287. doi: https://doi.org/10.1007/BF01234480
- Lothar W, Casper H, Milena P, Nikolai K, Jorgen AK. 2008. Human erythropoietin increases survival and reduces neuronal apoptosis in a murine model of cerebral malaria. Malaria J. 7:3. doi: https://doi.org/10.1186/1475-2875-7-3
- Mamta S, Jyoti S, Rajeev N, Dhatmendra S, Abhishek G. 2013. Phytochemistry of medicinal plants. J Pharmacogn Phytochem. 1(6):168–182.
- McCord J, Fridovich I. 1988. Superoxide dismutase: the first twenty years (1968–1988). Free Rad Biol Med. 5:363–369. doi: https://doi.org/10.1016/0891-5849(88)90109-8
- Mishra SK, Mohanty S. 2003. Problems in the management of severe malaria. J Trop Med. 1:1–10.
- Misra HP, Fridovich I. 1972. The role of superoxide anion in the autoxidation of epinephrine and a simple assay for superoxide dismutase. J Biol Chem. 247(10):3170–3175.
- Mohapatra KM. 2001. Profile of severe falciparum malaria in diabetics. Int J Diab Dev Ctries. 2:156–161.
- [NAS] National Academy of Sciences. 2011. Guide for the care and use of laboratory animals. 8th ed. Washington, DC: National Academy of Sciences.
- Nene-Bi SA, Soro TY, Zahoui OS, Traore F. 2013. Pharmacological effects of the roots and the stem barks extracts of Erythrina senegalensis DC (Fabaceae) in mammalians. Int J Pharmacogn Phytochem Res. 5(4):254–258.
- Nita Y, Rajesh Y, Anju GI. 2014. Chemistry of terpenoids. Int J Pharm Sci Rev Res. 27(2):272–278.
- Nnama T, Asomugha A, Asomugha R, Umesalugo K, Mgbemena I. 2017. Phytochemical analysis and acute toxicological study of Erythrina senegalensis ethanolic leaf extract in albino Wistar rats. Anat Physiol. 7:248–250. doi:https://doi.org/10.4172/2161-0940.1000248.
- Obidah W, Henry LB, Joseph A, Hakeino P, Husseini B. 2014. Effects of Erythrina senegalensis aqueous leaf extract in rats. Am J Res Commun. 2(4):179–185.
- Ochei J, Kolhatkar A. 2008. Medical laboratory sciences: theory and practice. New York, NY: Tata McGraw Hill; p. 663–665.
- Ogbodo SO, Okeke AC, Obu HA, Shu EN, Chukwurah EF. 2010. Nutritional status of parasitemic children from malaria endemic rural communities in eastern Nigeria. Curr Pediatr Res. 14:131–135.
- Omodeo-Salè F, Motti A, Basilico N, Parapini S, Olliaro P, Taramelli D. 2003. Accelerated senescence of human erythrocytes cultured with Plasmodium falciparum. Blood. 102:705–711. doi: https://doi.org/10.1182/blood-2002-08-2437
- Osuntokun TO, Ajayi AO, Olorunnipa TA, Thonda OA, Taiwo OV. 2016. Phytochemical screening and antimicrobial properties of partially purified ethyl acetate extracts of Erythrina senegalensis leaf and bark against selected clinical isolates. J Med Plants Stud. 4(3):259–269.
- Otvos J. 1999. Measurement of triglyceride-rich lipoproteins by nuclear magnetic resonance spectroscopy. Clin Cardiol J. 22(6):1121–1127.
- Percário S, Moreira DR, Moreira DR, Gomes BA, Ferreira ME, Gonçalves AC, Laurindo PS, Vilhena TC, Dolabela MF, Green MD. 2012. Oxidative stress in malaria. Int J Mol Sci. 13(12):16346–16372. doi:https://doi.org/10.3390/ijms131216346.
- Perrone RD, Madias NE, Levey AS. 1992. Serum creatinine as an index of renal function: new insights into old concepts. Clin Chem. 38(1):1933–1953. doi: https://doi.org/10.1093/clinchem/38.10.1933
- Peters W, Portus JH, Robinson BL. 1975. The chemotherapy of rodent malaria XXII. The value of drug resistant strains of P. berghei in screening for blood schizonticidal activity. Ann Trop Med Parasitol. 69:155–171. doi: https://doi.org/10.1080/00034983.1975.11686997
- Pimenta FP, Orfano AS, Bahia AC, Duarte AP, Ríos-Velásquez CM, Melo FF, Pessoa AC, Oliveira GA, Campos MM, Villegas LM, et al. 2015. An overview of malaria transmission from the perspective of Amazon Anopheles vectors. Mem Inst Oswaldo Cruz . 110(1):23–47. doi:https://doi.org/10.1590/0074-02760140266.
- Reitman S, Frankel S. 1957. A colorimetric method for the determination of serum glutamic oxaloacetate and glutamic pyruvic transaminases. Am J Clin Pathol. 28:56–63. doi: https://doi.org/10.1093/ajcp/28.1.56
- Rosenson RS, Wolff DA, Huskin AL, Helenowski IB, Rademaker AW. 2007. Fenofibrate therapy ameliorates fasting and postprandial lipoproteinemia, oxidative stress, and the inflammatory response in subjects with hypertriglyceridemia and the metabolic syndrome. Diabetes Care. 30(8):1945–1951. doi: https://doi.org/10.2337/dc07-0015
- Saidu K, Onah J, Orisadipe A, Olisola A, Wambebe C, Gammel K. 2000. Antiplasmodial and analgesic and anti-inflammatory activities of aqueous extract of stem bark of Erythrina senegaleusis. J Ethnopharmacol. 71(1):273–280.
- Saifi MA, Beg T, Harrath AH, Suleman F, Altayalan H, Quraishy SA. 2013. Antimalarial drugs: mode of action and status of resistance. Afr J Pharm Pharmacol. 7(5):148–156. doi: https://doi.org/10.5897/AJPPX12.015
- Tepongning RN, Yerbanga SR, Dori GU, Lucantoni L, Lupidi G, Habluetzel A. 2013. In vivo efficacy and toxicity studies on Erythrina senegalensis and Khaya ivorensis used as herbal remedies for malaria prevention in Cameroon. Eur J Med Plants. 3(3):454–464. doi: https://doi.org/10.9734/EJMP/2013/3928
- Togola A, Austarheim I, Theïs A, Diallo D, Paulsen B. 2008. Ethnopharmacological uses of Erythrina senegalensis: a comparison of three areas in Mali, and a link between traditional knowledge and modern biological science. J Ethnobiol Ethnomed. 4(1):6–17. doi: https://doi.org/10.1186/1746-4269-4-6
- Togola A, Diallo D, Dembele S, Barsett H, Paulsen BS. 2005. Ethnopharmacological survey of different uses of seven medicinal plants from Mali, (West Africa) in the regions Doila, Kolokani and Siby. J Ethnobiol Ethnomed. 1(1):7. doi: https://doi.org/10.1186/1746-4269-1-7
- Toth PP, Philip JB, Robert SR, William EB, Chapman MJ, Marina C, Ralph BDA, Michael HD, Davidson WS, Jay WH, et al. 2013. High-density lipoproteins: a consensus statement from the National Lipid Association. J Clin Lipidol. 7:484–525. doi: https://doi.org/10.1016/j.jacl.2013.08.001
- Trampuz A, Jereb M, Muzlovic I, Prabhu R. 2003. Severe and complicated malaria. Trans Roy Soc Trop Clin Rev Severe Crit Care. 4:315–323.
- Trease GE, Evans WC. 1989. Pharmacognosy. 13th ed. London: Bailliere Tindall Books Publishers; p. 1–105.
- Visser BJ, Wieten RW, Nagel I, Grobusch MP. 2013. Serum lipids and lipoproteins in malaria – a systematic review and meta-analysis. Malar J. 12:442–454. doi: https://doi.org/10.1186/1475-2875-12-442
- Wallin B, Rosengren B, Shertzer HG, Camejo G. 1993. Lipoprotein oxidation and measurement of TBARS formation in single microlitre plate; it’s use for evaluation of antioxidants. Anal Biochem. 208:10–15. doi: https://doi.org/10.1006/abio.1993.1002
- [WHO] World Health Organization. 2010. Malaria: fact sheet no. 94. Geneva: WHO Media Centre.
- [WHO] World Health Organization. 2016. World malaria report. Geneva: World Health Organization.
- [WHO] World Health Organization. 2017. World malaria report. [accessed 2019 Nov 10]. www.who.int/news-room/fact-sheets/detail/malaria/2017.
- [WHO] World Health Organization. 2018. World malaria report. [accessed 2019 Nov 10]. www.who.int/news-room/fact-sheets/detail/malaria/2018.
- Yasmeen K, Richa P, Karen S, Gaurav A, Nitin C. 2015. Haematological and biochemical alterations in malaria and their correlation with parasitic index. IOSR J Pharm. 5(9):53–56.