Abstract
This study investigated the application of crude methanol extract (CME) of Zapoteca portoricensis root and its methanol (MF) and ethyl acetate (EAF) fractions in managing benign prostatic hyperplasia (BPH). Forty-five albino rats (180-200 g) were divided into nine groups of five rats each: Group 1 served as normal control. BPH was induced in groups 2–9 using dihydrotestosterone and estradiol. Group 2 served as BPH-control and group 3 was dutasteride-treated. Groups 4 and 5, 6 and 7, and 8 and 9 were respectively treated with 200 and 400 mg/kg/d b.w. of CME, 200 and 400 mg/kg/d b.w. of MF, and 200 and 400 mg/kg/d b.w. of EAF, respectively for 14 days orally. The effects of the extracts on the hematological and biochemical indices of the rats were assessed. At 400 mg/kg/d b.w, p.o. using a gavage, CME, MF and EAF decreased prostatic specific antigen by 55.91%, 57.54% and 56.75%, respectively comparable to 58.80% by dutasteride (an indication of improved prostate status). The extracts also restored BPH-modified hematological and biochemical status of BPH-induced-extracts-treated rats compared to BPH-control. These findings suggest that Z. portoricensis root extracts normalize aberrations associated with BPH and may justify the plant’s folkloric use in the management of BPH.
Introduction
Benign prostatic hyperplasia (BPH), the most common condition in ageing men is a non-cancerous proliferation of epithelial and stromal cells of the prostate gland. It is characterized by hyperplasia of prostatic tissues in the periurethral transition zone of the prostate that surrounds the urethra (Roehrborn Citation2008). Clinically, BPH manifests as lower urinary tract symptoms (LUTS) consisting of irritating and obstructive symptoms. Prolonged obstructions may lead to acute urinary retention, recurrent urinary tract infection, hematuria, bladder calculi, and renal insufficiency (Foo Citation2017). The prevalence of LUTS due to BPH increases with age (Ezeanyika et al. Citation2006; Dhingra and Bhagwat Citation2011). The exact cause of BPH is not well elucidated and the risk factors are not clearly defined. A variety of factors chiefly age, genetics, lifestyle, hypertension, obesity, diabetes and insulin resistance have been linked to the development of BPH (Ejike and Ezeanyika Citation2008). Experts consider BPH to involve hyperplasia or hypertrophy of the prostatic stromal epithelial cells, resulting in the formation of a large or discrete nodule in the preurethral region of the prostate. When the nodules grow large, they compress the urethral canal leading to partial or sometimes total obstruction of the urethra. This thus interferes with the normal urine flow (Boyd Citation2010).
The contribution of sex steroids in the development of BPH has been documented (Asiedu et al. Citation2017; Ajayi and Abraham Citation2018). Metabolites of testosterone such as dihydrotestosterone (DHT) and estrogen have been implicated in BPH pathogenesis. Studies have shown that as men age, their plasma DHT level decreases while estrogen level increases (Da Silva and De Souza Citation2019; Rastrelli et al. Citation2019). Findings from animal studies showed that BPH results from high estrogen level within the prostate, and that the accumulation of DHT in the prostate increase the activity of growth factors that promote prostatic cell growth (Sun et al. Citation2008; Veeresh et al. Citation2010). Elevation in prostatic specific antigen (PSA) level is a major indicator of BPH and other prostate disorders (Cannon and Getzenberg Citation2008; Pudasaini et al. Citation2019).
Alterations in liver and kidney status in BPH have been a matter of concern. This is because of their role in mediating poor quality of life of BPH sufferers. Chung and Lin (Citation2011) opined that liver cirrhosis increases the risk of development BPH while Akdogan et al. (Citation2002) reported the existence of abnormal PSA level in patients with liver cirrhosis, suggesting a link between BPH and liver dysfunction. In agreement with this suggestion, Meludu et al. (Citation2017) reported elevated serum activities of liver function enzymes in BPH patients. Similarly, a correlation between BPH and renal dysfunction has been documented (Basatac and Cicek Citation2015; Chilumula et al. Citation2018). It becomes necessary to evaluate the status of the liver and kidney of BPH patients during treatment as additional indices of treatment progress.
BPH is commonly treated with surgical procedures that will allow normal voluntary expression of urine. Additionally, medications such as α-blockers and 5α-reductase inhibitors that target reduction in prostate mass or relaxation in muscle tone are used to manage BPH (Braeckman and Denis Citation2017). However, these drugs have many side effects (Trost et al. Citation2013; Hagberg et al. Citation2016). This warrants a search for additional drugs that are effective and safe. Plant products are currently being explored for the management of BPH patients; a good number of men diagnosed with BPH use complementary and alternative medicines (Steemkamp Citation2003; Ejike and Ezeanyika Citation2011).
Several plants have been reported to exhibit positive outcome in experimentally-induced BPH in animal models (Akinyemi et al. Citation2012). Zapoteca portoricensis (jacq) H. M Hernandez (Fabaceae), commonly called ‘white stick’ is a perennial, seasonal shrub with slender unarmed branches. It is widespread in West Africa, West Indies and Atlantic coast of America, and is widely used as a medicinal plant in Eastern and Southern Nigeria. Extracts from different parts of the plant has been traditionally used in the management of diarrhoea, convulsion, tonsillitis and BPH (Agbo et al. Citation2010). Root extracts of the plant have been shown to have trypanocidal (Nwodo et al. Citation2014), antiulcer (Ukwe et al. Citation2010), hepatoprotective and antioxidant (Agbafor et al. Citation2014), antimicrobial and anti-inflammatory activities. Recently, we reported that methanol extract of the plant root and its fractions are rich in phytochemicals and are safe up to 5,000 mg/kg body weight (Ikeyi et al. Citation2019). Considering the hypothesis that inflammation contributes to BPH development (Chughtai et al. Citation2011; Elkahwaji Citation2013; De Nunzio et al. Citation2016; Krušlin et al. Citation2017) and that Z. portoricensis has anti-inflammatory activity (Nwodo and Uzochukwu Citation2008; Agbo et al. Citation2010), we suggest that the plant may be beneficial in the management of BPH and hence, the aim of this study.
Material and methods
Plant materials and extraction procedure
Fresh roots of Zapoteca portoricensis were sourced from a habitat in Udenu Local Government Area, Enugu State, Nigeria. The plant materials were authenticated by Mr. Alfred Ozioko, a taxonomist with Bioresource Development and Conservation Program (BDCP) Research Center Nsukka, Enugu State, Nigeria. The authenticity of the identification was confirmed by comparison with features in plant databases (http://www.theplantlist.org/ and http://www.ipni.org/). The fresh roots were air-dried to constant weight and milled into coarse powder. Crude methanol extract of the plant material was prepared by pulverizing 2.0 Kg of powdered root sample in 10 L of methanol for 72 h, after which the suspension was filtered. The extraction procedure was repeated twice and all the filtrates were combined and dried (rotary evaporator at 450C under reduced pressure) to obtain the crude methanol extract (CME). Part of CME was fractionated by column chromatography using methanol and ethyl acetate as solvents to obtain methanol (MF) and ethyl acetate (EAF) fractions, respectively.
Study animals
Adult healthy male rats of Wistar strain (130-150 g) obtained from the Animal Holding Unit of the Department of Zoology and Environment Biology, University of Nigeria, Nsukka were used for this study. The rats were housed under standard conditions (25 ± 20C and 12 h light/dark cycle), fed commercial rodent pellets (Grand Cereals Ltd., Enugu, Nigeria) and given unrestricted access to portable water. Institutional, national, and international guidelines for the care and use of animals for research were followed in this study.
Pilot study
A pilot study was conducted to confirm the reproducibility of the earlier reported method of BPH induction (Sun et al. Citation2008; Nandecha et al. Citation2010; Veeresh et al. Citation2010). Eighty-four rats randomized into 14 groups of 8 rats were used. The PSA levels of all the rats were determined on day 0 (prior to induction). One group served as normal control (not induced) while BPH was induced in the other 13 groups by daily subcutaneous administration of DHT and estradiol (400 and 80 µg/ml, respectively) in olive oil for 28 days. On day 29, the PSA levels of the rats were determined and the prostate of 4 rats in each of the induced groups were compared with control. Rats with significantly higher PSA levels in addition to macroscopically observed enlargement in prostate gland compared to normal control (non-induced rats) were defined to have BPH. The remaining 4 rats in each group were treated as follows: group 2 served as BPH-control (BPH-induced but not treated). Groups 3–6 were administered 100, 200, 400 and 600 mg/kg/d b.w. CME, respectively. Groups 7–10 received 100, 200, 400 and 600 mg/kg/d b.w. MF, respectively while groups 11–14 were treated with 100, 200, 400 and 600 mg/kg/d b.w. EAF, respectively. All treatment was by oral and lasted for 14 days. On day 15, the PSA levels and the prostate size of the 4 rats in each treatment group (groups 3-14) were compared with groups 1 and 2 and as well as the results of day 29 (the day after completion of BPH induction). Treatment doses (200 and 400 mg/kg/d b.w.) were selected because they optimally reduced the PSA levels and prostate gland size compared to BPH-control and normal control, and results prior to treatment.
Experimental design for the effect of the extracts on benign prostatic hyperplasia
Forty-five rats used for this study were divided into nine groups of five rats each: Group 1 served as normal control (normal rats, not BPH-induced and not treated with extract but vehicle only), group 2 served as BPH control (BPH-induced and treated with vehicle only) and group 3 served as standard control (BPH-induced and treated with dutasteride (Avodart®)). Groups 4 and 5 were BPH-induced and treated with 200 and 400 mg/kg body weight of CME, respectively. Groups 6 and 7 were BPH-induced and treated with 200 and 400 mg/kg body weight of MF, respectively. Groups 8 and 9 were BPH-induced and treated with 200 and 400 mg/kg body weight of EAF, respectively. The plant extracts and standard drug were dissolved in 3% tween-80 in normal saline as vehicle. All treatments were administered once daily via gavage for 14 days, after which fasted blood samples were drawn from each rat for hematological and biochemical assays.
Determination of prostate status: Serum PSA levels were analysed with a PSA ELISA kit (Biocheck Inc. CA, USA) and the absorbance was read at 450 nm using an ELISA Figure reader (BioTek 800TM absorbance reader). The activity of serum acid phosphatase (ACP) was assayed using the colorimetric method of Tietz (Citation1995) as outlined in Randox Kit (UK). The principle is that α-naphthol released when acid phosphatase cleaves α-naphthyl phosphate is coupled with Fast-Red-TR to produce a coloured complex that absorbs maximally at 405 nm.
Determination of hematological indices: Hematological indices such as haemoglobin (Hb), packed cell volume (PCV), red blood cells (RBC) and white blood cell (WBC) counts were determined following the method described by Ochei and Kolhatkar (Citation2008) (Sysmex KX-21N automated hematology analyser).
Determination of liver status: Liver status was assessed by evaluating liver function parameters using Randox diagnostics kits (UK) as follows: The activity of aspartate aminotransferase (AST) was assayed by colorimetric method of Reitman and Frankel (Citation1957). AST activity was assayed by monitoring the formation of oxaloacetate hydrazine with 2,4-dinitrophenylhydrazine whose absorbance was read at 546 nm. Similarly, the activity of alanine aminotransferase (ALT) was assayed by colorimetric method of Reitman and Frankel (Citation1957). ALT activity was measured by monitoring the concentration of pyruvate hydrazine formed with 2,4-dinitrophenylhydrazine whose absorbance was read at 546 nm. The activity of alkaline phosphatase (ALP) was assayed by colorimetric method of Babson et al. (Citation1996). ALP acted upon AMP-buffered sodium thymolphthalein monophosphate while the addition of an alkaline reagent stops enzyme activity and simultaneously develops a blue colour, which was read at 405 nm.
Determination of kidney status: The kidney status was determined by assessing renal function parameters using Randox diagnostics kits (UK) according to the methods and principles outlined briefly below: The concentration of serum creatinine was determined using the colorimetric method of Tietz (Citation1995). Creatinine in alkaline solution reacts with picric acid to form a coloured complex which absorbs maximally at 510 nm. The amount of the complex formed is directly proportional to the creatinine concentration. The concentration of serum uric acid was determined according to the colorimetric method of Fossati et al. (Citation1980). Uric acid is converted to allantoin and hydrogen peroxide, which under the catalytic influence of peroxidase, oxidizes 3,5-dichloro-2-hydroxybenzenesulfonic acid and 4-aminophenazone to form a red-violet quinoneimine compound whose absorbance were read at 546 nm. The concentration of serum urea was determined using colorimetric method of Tietz (Citation1995). Urea in serum is hydrolyzed to ammonia in the presence of urease. The ammonia is then measured spectrophotometrically by Berthelot’s reaction at 546 nm. The serum total protein level was determined using colorimetric method of Tietz (Citation1995). Cupric ions in an alkaline medium interact with protein peptide bonds resulting in the formation of a coloured complex whose absorbance was read at 500 nm. Serum electrolytes such as bicarbonate (HCO3-), chloride (Cl-), sodium (Na+) and potassium (K+) levels were determined according to the colorimetric method of Tietz et al. (Citation1976) while selenium level was determined using colorimetric method of Bonhorst and Mattice (Citation1959).
Determination of serum lipid profile: the serum lipid profile panel was determined using Randox diagnostics kits (UK) according to the methods and principles outlined briefly below: Serum total cholesterol (CHOL) concentration was determined according to the method of Roeschlau et al. (Citation1974). The principle of this test is the enzymatic hydrolysis and oxidation of cholesterol to produce hydrogen peroxide. The action of peroxidase on hydrogen peroxide in the presence of phenol and 4-aminoantipyrine forms a coloured indicator, a quinoneimine whose absorbance was read at 546 nm. Serum triacylglycerols (TAGs) concentration was determined according to the colorimetric method of Tietz et al. (Citation1976). TAGs are determined after enzymatic hydrolysis with lipases. The indicator is a quinoneimine formed from hydrogen peroxide, 4-aminophenazone and 4-chlorophenol under the catalytic influence of peroxidase. The absorbance was measured at 546 nm. Serum high density lipoproteins (HDL) concentration was determined by the method of Albers et al. (Citation1978). Very low density (VLDL) and low density (LDL) lipoproteins in the sample were precipitated with phosphotungstate and magnesium ions, leaving the supernatant which contains HDL which absorbs maximally at 500 nm. Serum LDL concentration was determine as the difference between total cholesterol and the cholesterol content of the supernatant after precipitation of the LDL fraction by polyvinyl sulphate (PVS) in the presence of polyethyleneglycol monomethyl ether. Serum LDL concentration was calculated using the Friedewald formula (Friedewald et al. Citation1972) while serum VLDL concentration was calculated as previously reported (Friedewald et al. Citation1972; Martin et al. Citation2013).
Determination of antioxidant and lipid peroxidation status: The superoxide dismutase (SOD) activity was assayed by the method of Arthur and Boyne (Citation1985). The method employed xanthine and xanthine oxidase to generate superoxide radicals which reacts with 2(-4-iodophenyl)-3(-4-nitrophenol)-5-phenyltetrazolium chloride (INT) to form a red formazan dye that absorbs maximally at wavelength 505 nm. The superoxide dismutase activity was measured by the degree of inhibition of the above reaction. The activity of catalase (CAT) was assayed by the method of Sinha (Citation1972). Dichromate in acetic acid is reduced to chromic acetate and when heated in presence of hydrogen peroxide (H2O2), there is a formation of chromic acid as an unstable intermediate. The activity of CAT was assayed by measuring absorbance of chromic acetate at wavelength of 570 nm. Similarly, the glutathione concentration was determined by the method of King and Wootton (Citation1959) while vitamin C (ascorbic acid) concentration was determined using the method of Tietz (Citation1983). Ascorbic acid is oxidized and converted to diketoglutamic acid, a strong acid that reacts with 2,4-dinitrophenylhydrazine to form a diphenyl hydrazine (a red coloured compound) whose absorbance was read at a wavelength of 540 nm. Lipid peroxidation was estimated by measuring the level of malondialdehyde (MDA), lipid peroxidation product as described by Wallin et al. (Citation1993).
Histopathological investigation: After sacrifice, the prostate tissue of a rat in each group was removed and fixed with 10% formalin. The histo-architecture of the tissue sections were examined according to Disbrey and Rack (Citation1974) and Drury and Wallington (Citation1967) protocols using standard hematoxylin and eosin solutions as stains.
Statistical analysis
Laboratory data were analysed using one-way analysis of variance (ANOVA) in Statistical Product and Service Solutions (SPSS), version 18. The post hoc Duncan's test was used to compare means between test and control groups. Results were expressed as mean ± standard deviation (SD) and test of significance was determined at p < 0.05.
Results and discussion
Effects of crude methanol extract and its fractions on prostate status of BPH-induced rats
Treatment of BPH-induced rats with CME, MF and EAF significantly (p < 0.05) decreased PSA levels and ACP activities when compared with BPH-control. The decrease in PSA levels by the extract is comparable to that obtained with standard drug, dutasteride (Table ). This result indicates that the extracts have modulatory effects on prostate enlargement (BPH). The result agrees with a recent report that treatment of BPH-induced rats with ethanol extract of Z. portoricensis stem has positive outcome by reducing PSA level and prostate weight (Joshua et al. Citation2018). PSA is generally elevated in BPH and other prostate disorders and is a reliable marker of BPH. This is because reductions in PSA level and ACP activity are associated with reduced prostate hyperplasia (Ejike and Ezeanyika Citation2011). The mechanism of action of these Z. portoricensis extracts may be by inhibition of 5α-reductase and/or its anti-inflammatory activities as earlier reported (Agbo et al. Citation2010). In line with our findings, reports of earlier studies, both human and animal studies showed that herbal-based drugs from Serenoa repens, Cucurbita pepo, Epilobium parviflorum, Urtica dioica, Pygeum africanum (Chatelain et al. Citation1999), Lepidium meyenii (Gasco et al. Citation2007) and others improve urologic symptoms and quality of lives in BPH. In combination as ProstateEZE Max, the above plants showed significant positive effect on BPH symptoms (Coulson et al. Citation2013). Our result supports the observations by Park et al. (Citation2019) that aqueous extract of Lespedeza cuneate has ameliorative effects on BPH. Our results also agree with the findings of Mohamed et al. (Citation2016) who recorded positive outcome on experimentally-induced BPH administered ethanol extract of flaxseed, sesame seed, safflower seed and soybean. In addition and in consonance with our report, Liu et al. (Citation2019) showed that Pao pereira extract attenuates complications associated with BPH by inhibiting 5α-reductase activity. From these observations, it can be inferred that plant-derived agents could be sources of new generation of drugs for managing BPH.
Table 1. Effect of crude extract and its fractions on the prostatic specific antigen level and alkaline phosphatase activity of BPH-induced rats.
Effects of crude methanol extract of Zapoteca portoricensis root and its fractions on hematological indices of experimental rats
Significantly (p < 0.05) higher Hb concentration, PCV, and RBC and WBC counts were recorded in BPH-induced rats treated with CME, MF and EAF than in BPH-control (Table ). The increase in WBC count on administration of the extracts showed that the extract may possess immunomodulatory effects (Iweala and Ogidigo Citation2015a). The observed increase in Hb concentration, PCV and RBC count in BPH-induced-extracts-treated rats indicates that the plant extracts have stimulatory effect on erythropoiesis.
Table 2. Effect of extract on the hematological induces of BPH-induced rats.
Effects of crude methanol extract of Zapoteca portoricensis root and its fractions on serum liver markers of experimental rats
Hepatotoxicity is one of the side effects of several synthetic drugs. To assess the modulation of liver alterations by BPH and the effect of the extracts on the liver, we assayed the activities of AST, ALT and ALP in serum of BPH-induced rats treated with Z. portoricensis root extracts as biomarkers of hepatic function. The results showed that the plant extracts did not cause significant (p > 0.05) change in AST, ALT and ALP activities (Table ), indicating that the extracts are not hepatotoxic. This is characterized by no significant difference when the liver status of normal control and BPH-induced rats treated with extracts were compared. Elevations in activities of these enzymes in serum are largely due to liver damage by hepatotoxins. Liver damage is characterized by liver cell permeability, congestion, cell rupture, disruption in the integrity of plasma membrane of the hepatocytes, loss of cellular compounds into the blood, blockade in the bile duct or injury in the liver (Erhirhie et al. Citation2015; Moke et al. Citation2015).
Table 3. Effect of extract and its fractions on the liver status of BPH-induced rats.
Effects of crude methanol extract of Zapoteca portoricensis root and its fractions on serum kidney markers of experimental rats
Considering the documented link between BPH and kidney (Basatac and Cicek Citation2015; Chilumula et al. Citation2018), and also to check the effects of the extract on kidney, we evaluated the indicators of kidney status of BPH-induced rats treated with the extracts. There were significant (p < 0.05) decreases in creatinine and uric acid concentrations and no significant (p > 0.05) difference among urea and total protein concentrations of BPH-induced rats treated with extracts when compared with BPH-control (Table ). Elevated levels of creatinine, urea, uric acid and total protein in urine and serum are indicators of impaired renal function. Therefore, in the presence of chronic or severe BPH, abnormalities in creatinine and uric acid levels as seen in Table are indicators of kidney damage (Vyas and Argal Citation2012). This observation agrees with the earlier report on the link between BPH and renal damage (Rule et al. Citation2005a; Rule et al. Citation2005b). Our results indicate that the plant extracts modulate impaired kidney function in BPH-induced rats. A significant (p < 0.05) elevation in serum sodium and chloride concentrations of BPH-induced rats treated with the extracts when compared with BPH-control was observed. Induction of BPH does not significantly (p < 0.05) affect serum bicarbonate, potassium and selenium concentrations (Table ). Elevation in serum electrolytes results in metabolic or respiratory acidosis or alkalosis and the extracts appear to have cellular protective properties. The result of this study indicates a reversal effect of the plant root extracts on electrolyte imbalance caused by BPH.
Table 4. Effect of extract and its fractions on the kidney status of BPH-induced rats.
Table 5. Effect of extract and its fractions on the serum electrolytes of BPH-induced rats.
Effects of crude methanol extract of Zapoteca portoricensis root and its fractions on serum lipid profile of experimental rats
To assess the modulatory effect of the extracts on lipid profile of BPH subjects, total cholesterol, triacylglycerol, high density lipoprotein, low density lipoprotein and very low density lipoprotein concentrations of BPH-induced rats treated with the extracts were compared with BPH-control. Compared to normal control, BPH-induced rats had higher total cholesterol, triacylglycerol, low density lipoprotein and very low density lipoprotein concentrations and lower high density lipoprotein concentration. However, a significant (p < 0.05) reduction in total cholesterol, triacylglycerol and low density lipoprotein concentrations, and an elevation in high density lipoprotein concentration were observed upon treatment of BPH-induced rats with extracts (Table ). Studies have shown an association among dyslipidemia, obesity and BPH (Nandeesha et al. Citation2006; Tewari et al. Citation2011; Citation2014), which represents potential risk factors in BPH (Oh et al. Citation2007; Liu et al. Citation2010; Duru et al. Citation2015). Our result suggests that the extract showed some anti-hyperlipidemic and modulatory effects on dyslipidemia induced by BPH.
Table 6. Effect of extract and its fractions on the serum lipid profile of BPH-induced rats.
Effects of crude methanol extract of Zapoteca portoricensis root and its fractions on antioxidant and lipid peroxidation status of experimental rats
Lipid peroxidation is an established mechanism of cellular injury and malondialdehyde (MDA), a product of lipid peroxidation is commonly used in monitoring its level (Aydin et al. Citation2006). The development of BPH has been associated with increased lipid peroxidation, inflammatory process and decreased antioxidant status (Mbaka et al. Citation2013; Iweala and Ogidigo Citation2015b). Polyunsaturated fatty acid peroxides generate MDA and 4-hydroxyalkanals upon decomposition (Esterbauer et al. Citation1991). High level of these peroxides causes oxidative damage to cells leading to mutagenesis and uncontrolled cell growth (Chelikani et al. Citation2004). We evaluated the antioxidant potentials of the extracts by examining serum levels of GSH and vitamin C, and activities of SOD and CAT in the BPH-induced rats treated with the extracts. We also assessed the modulatory effects of the extracts by measuring the level of MDA in BPH-induced rats treated with the extracts. There were significant (p < 0.05) decrease in MDA concentration and significant (p < 0.05) increase in SOD activity in BPH-induced and extracts-treated rats when compared with BPH-control (Table ). In cells, glutathione is maintained in its reduced form by glutathione reductase. GSH in turn serve as co-enzymes to some enzymes (like glutathione peroxidase and glutathione-S-transferase), and reacts directly with oxidants. In general, the results indicate that Z. portoricensis root extracts have antioxidant potential and reduce the rate of lipid peroxidation. This antioxidant property might be attributed to the rich phytochemical constituents of the extracts such as the flavonoids, saponins, phenols and terpenoids (Ikeyi et al. Citation2019), that are involved in scavenging free radicals and reducing lipid peroxidation.
Table 7. Effect of extract and its fractions on the antioxidant and lipid peroxidation status of BPH-induced rats.
Effects of crude methanol extract of Zapoteca portoricensis root and its fractions on prostate histology of BPH-induced rats
Generally, it can be deduced from the result of the histological examination that prostate tissue from normal control (Figure ) did not show any observable pathological change; its normal architectural appearances were intact. The observed concretions seen in BPH control (Figure ) may have caused the ducts to dilate as usually seen in benign prostate glands. The presence of concretions is microscopically used to identify benign prostate. It depicts possible abnormal mass development that have become calcified and/or due to condensation of secretory materials. The reduced stroma or degeneration of stroma observed in this study indicates possible reduction of prostatic fluid. This is because the connective tissues which usually surround the individual glandular units contain numerous smooth muscle cells, called fibromuscular stroma which aids in the rapid discharge of prostatic fluids at ejaculation. The presence of few inflammatory neutrophil infilterates compared to BPH control, cribriforms pattern and reduction in intraluminal secretions and papillary infolding, especially in test groups treated with 400 mg/kg methanol fraction (Figure ) and 400 mg/kg ethylacetate fraction (Figure ) are suggestive of overall histological improvement when compared with BPH control (Figure ). The histomorphological changes in prostate tissue sections as seen in Figure when compared with Figure agrees with the features earlier reported (Hassan et al. Citation2013; Mbaka et al. Citation2017). The mechanism of hyperplasia observed may be related to accumulation of DHT in the prostate, which binds to nuclear hormone receptor and triggers cellular growth. Most of the hyperplasia is contributed by glandular proliferation, but the stroma is also increased in thickness. These histological observations support the results of serum analyses that treatment of BPH-induced rats with crude methanol extract of Z. portoricensis root and its fractions normalizes aberrations associated with BPH (Figures –).
Figure 1. Photomicrograph of prostate tissue section of a rat from group 1 (normal control) showing intact seminal vesicles (black arrow), epithelium, coagulating glands (blue arrow) and vas deferens (red arrow). H&E. mag. 400X.
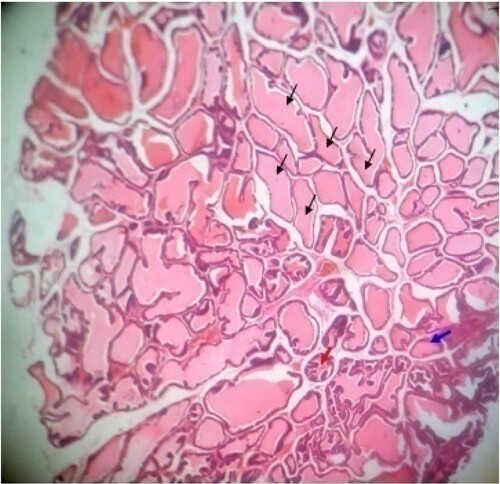
Figure 2. Photomicrograph of prostate tissue section of a rat from group 2 (BPH control; BPH-induced and untreated rats) showing (1) Slight disruption of architectural appearances: There is loss of intracellular papillary infoldings (blue arrow) and are haphazardly distributed with abnormal projection. There were numerous cribriform patterns (black arrow) in keeping for possible adenoma and there is presence of neutrophil infilterates in keeping with possible acute inflammation. H&E. mag. 400X.
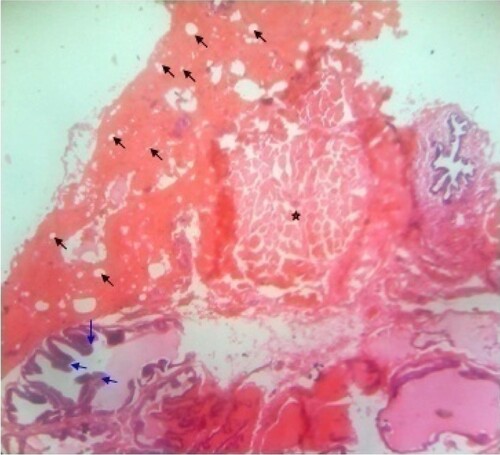
Figure 3. Photomicrograph of prostate tissue section of a rat from group 1 (BPH-induced and treated with Avodart®) showing multiple infoldings and papillary projections into the lumen (black arrow) of the seminal vesicles duct and some of the seminal vesicles were demarcated with fibrous capsules (star) and at the same time compressing surrounding tissues. However, there is normal amount of intraluminal secretions. H&E. mag. 400X.
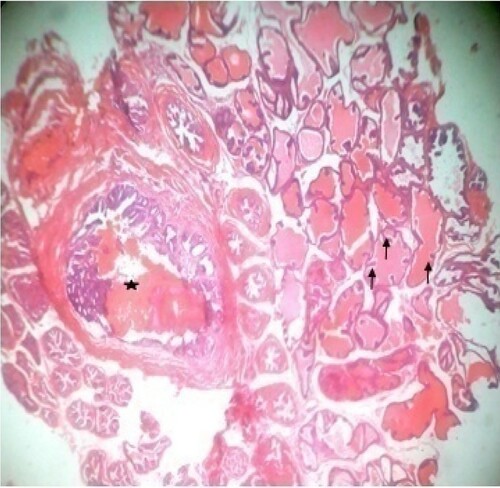
Figure 4. Photomicrograph of prostate tissue section of a rat from group 4 (BPH-induced and treated with 200 mg/kg of CME) showing mild focal adenoma with areas of atypical hyperplasia and mild squamous papilloma appearances. H&E. mag. 400X.
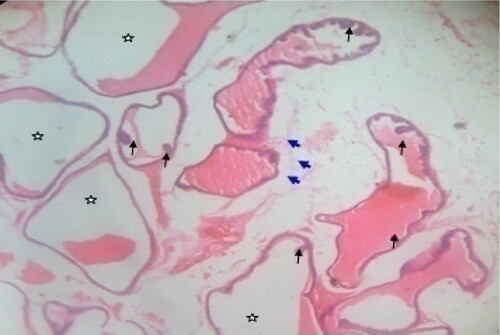
Figure 5. Photomicrograph of prostate tissue section of a rat from group 5 (BPH-induced and treated with 400 mg/kg of CME) showing reduced intraluminal secretions (star) in the seminal vesicle ducts which keeps for possible focal squamous metaplasia and projections of papillary (appears hyperplastic and arranged as stalks protruding in lumen of the duct) (black arrow) keeping for possible benign condition. Also, the ducts are distended due to the presence of papilloma usually called squamous papilloma. There is presence of few inflammatory infilterates (blue arrow) keeping for possible hyperplasia usually called reactive hyperplasia found in the seminal vesicles. H&E. mag. 400X.
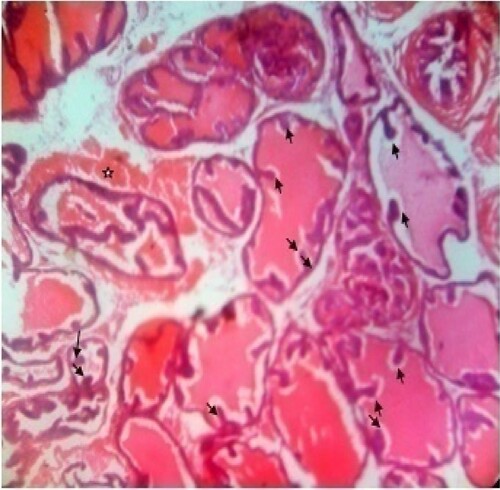
Figure 6. photomicrograph of prostate tissue section of a rat from group 6 (BPH-induced and treated with 200 mg/kg of MF) showing extended papillary projections (black arrow) although there are more intra acinar papillary infoldings with reduced intraluminal secretions (star). H&E. mag. 400X.
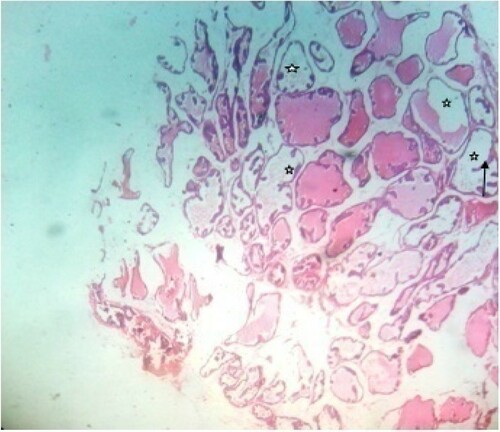
Figure 7. Photomicrograph of prostate tissue section of a rat from group 7 (BPH-induced and treated with 400 mg/kg of MF) showing hyperplasia of prostrate ducts with intact fibromuscularstroma (star) together with invasion of intraprostatic perineural spaces (black arrow). There is concretions especially corpora amylacea. The gland pattern appears small with loss of columnar or cubiodal cells (yellow arrow) H&E. mag. 400X.
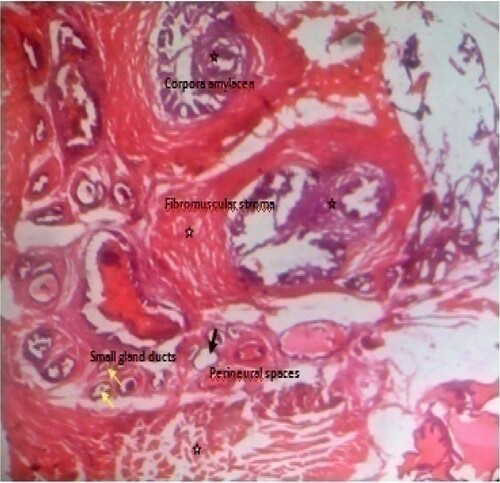
Figure 8. Photomicrograph of prostate tissue section of a rat from group 8 (BPH-induced and treated with 200 mg/kg of EAF) showing diffuse adenomas which appear in a cribriform pattern (black arrows) in the ventral prostrate which are visible and also reduced stroma mainly fibromuscular was equally evident. H&E. mag. 400X.
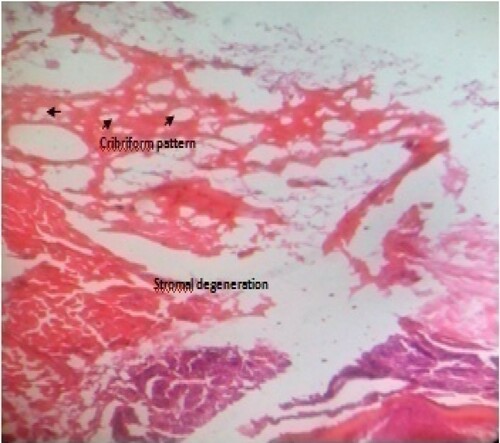
Figure 9. Photomicrograph of prostate tissue section of a rat from group 9 (BPH-induced and treated with 400 mg/kg of EAF) showing concretions especially corpora amylacea which appears as a hard compact mass usually ovoid in shape (black star) with loss of fibromuscular stroma (white star) and reduced convolutions usually papillary infoldings. At the same time there is reduced intraluminal secretions (red star) H&E. mag. 400X.
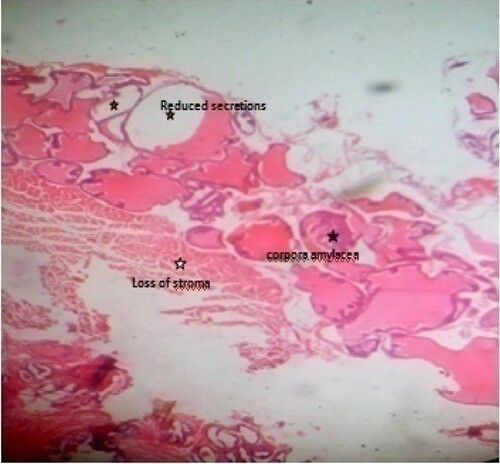
Conclusion
Results of this study suggest that crude methanol extract of Zapoteca portoricensis root and its methanol and ethyl acetate fractions normalize hematological and biochemical aberrations associated with BPH. This bioactivity exhibited by the extracts may be attributed to their phytochemical constituents as already reported. These findings may be the scientific backup to the folkloric use alcoholic extracts of Z. portoricensis root in the management of BPH in South Eastern Nigeria. Further studies are warranted to isolate and characterize the specific bioactives responsible for the above observed activities; these bioactives may serve as promising candidates for treatment of BPH and other prostate related disorders.
Disclosure statement
No potential conflict of interest was reported by the author(s).
Data availability
Data used for this study have been embedded in the manuscript.
References
- Agbafor KN, Ogbanshi ME, Akubugwo EI. 2014. Phytochemical screening, hepatoprotective and antioxidant effect of leaf extract of Zapoteca portoricensis. Adv Biol Sci. 4:35–39.
- Agbo MO, Okoye FBC, Nwodo JN. 2010. In vivo anti-inflammatory effect of Zapoteca portoricensis. Int J Health Res. 3(1):29–35.
- Ajayi A, Abraham K. 2018. Understanding the role of estrogen in the development of benign prostatic hyperplasia. Afr J Urol. 24:93–97.
- Akdogan M, Hassoun BS, Gurakar A, El-Sahwi K, Jazzar A, Wright H, Sebastian A, Nour B. 2002. Prostate-specific antigen levels among cirrhotic patients. Int J Biol Markers. 17(3):161–164.
- Akinyemi RA, Huthman IO, Adesanya OA, Akpan HB, Adefule AK. 2012. Effect of the methanolic extract of Trichosanthes cucumerina seed (Snake Gourd/tomato) on experimentally-enlarged prostate gland in adult wistar rats. Res Rev J Med Health Sci. 1(1):10–17.
- Albers JJ, Warmick GR, Cheng MC. 1978. Determination of high density lipoprotein (HDL)-cholesterol. Lipids. 13:926–932.
- Arthur JR, Boyne R. 1985. Superoxide dismutase and glutathione peroxidase activities in neutrophil from selenium deficient and copper deficient cattle. Life Sci. 36:1569–1575.
- Asiedu B, Anang Y, Nyarko A, Doku DA, Amoah BY, Santa S, Ngala RA, Asare GA. 2017. The role of sex steroid hormones in benign prostatic hyperplasia. Aging Male. 20(1):17–22.
- Aydin A, Arsova-Sarafinovska Z, Sayal A, Eken A, Erdem O, Erten O, Ozok Y, Dimovski A. 2006. Oxidative stress and antioxidant status in non-metastatic prostate cancer and benign prostatic hyperplasia. Clin Biochem. 39:176–179.
- Babson AL, Greely SJ, Coleman CM, Philips GE. 1996. Phenolphthalein monophosphate as a substrate for serum alkaline phosphatase. Clin Chem. 12(18):482–490.
- Basatac C, Cicek MC. 2015. A huge benign prostatic hyperplasia presenting with renal failure. J Surg Case Rep. 6:1–2.
- Bonhorst CW, Mattice JJ. 1959. Colorimetric determination of selection in biological materials. Anal Chem. 31(12):2106–2107.
- Boyd L. 2010. What causes BPH? Accessed August 10, 2019 www.livestrong.com/article/87491/causes-bph.
- Braeckman J, Denis L. 2017. Management of BPH then 2000 and now 2016: from BPH to BPO. Asian J Urol. 4:138–147.
- Cannon GW, Getzenberg RH. 2008. Biomarkers for benign prostatic hyperplasia progression. Curr Urol Rep. 9(4):279–283.
- Chatelain C, Autet W, Brackman F. 1999. Comparison of once and twice daily dosage forms of Pygeum africanum extract in patients with benign prostatic hyperplasia: a randomized, double-blind study, with long-term open label extension. Urology. 54:473–478.
- Chelikani P, Fita I, Loewen P. 2004. Diversity of structures and properties among catalases. Cell Mol Life Sci. 61(2):192–208.
- Chilumula R, Devaraj R, Sriramoju V, Dondapati T, Prakash A. 2018. Analysis of risk factors and outcome of renal failure in benign prostatic hyperplasia. J Clin Diagn Res. 12(1):PC01–PC03.
- Chughtai B, Lee R, Te A, Kaplan S. 2011. Role of inflammation in benign prostatic hyperplasia. Rev Urol. 13(3):147–150.
- Chung SD, Lin HC. 2011. Increased risk of benign prostatic enlargement among patients with liver cirrhosis: a nationwide population-based study. J Androl. 32(2):159–164.
- Coulson S, Rao A, Beck SL, Steels E, Gramotney H, Vitetta L. 2013. A phase II randomized double-blind placebo-controlled clinical trial investigating the efficacy and safety of ProstateEZE Max: A herbal medicine preparation for the management of symptoms of benign prostatic hypertrophy. Complement Ther Med. 21:172–179.
- Da Silva MHA, De Souza DB. 2019. Current evidence for the involvement of sex steroid receptors and sex hormones in benign prostatic hyperplasia. Res Rep Urol. 11:1–8.
- De Nunzio C, Presicce F, Tubaro A. 2016. Inflammatory mediators in the development and progression of benign prostatic hyperplasia. Nature Rev Urol. 13:613–626.
- Dhingra N, Bhagwat D. 2011. Benign prostatic hyperplasia: An overview of existing treatment. Indian J Pharmacol. 43(1):6–11.
- Disbrey BD, Rack JH. 1974. Histological laboratory methods. Edinburgh: Livingstone; p. 56–128.
- Drury RAB, Wallington EA. 1967. Careton’s histological technique, 4th ed. London: Oxford University Press; p. 120–123.
- Duru RC, Njoku OU, Maduka IC, Ugonabo MC, Ugwueme FO. 2015. Artherogenic lipid markers and testosterone levels in Nigerian men with prostate disorders. Asian Pacific J Health Sci. 2:48–55.
- Ejike CE, Ezeanyika LUS. 2008. Metabolic syndrome in sub-Saharan Africa: smaller twin of a regions prostatic disease. Int Urol Nephrol. 40(4):909–920.
- Ejike CE, Ezeanyika LUS. 2011. Management of experimental BPH in rats using a food based therapy containing Telfairia occidentalis seeds. AJTCAM. 8(4):398–340.
- Elkahwaji JE. 2013. The role of inflammatory mediators in the development of prostatic hyperplasia and prostate cancer. Res Rep Urol. 5:1–10.
- Erhirhie EO, Ilodigwe EE, Ajaghaku DL, Mbagwu IS, Moke EG. 2015. Toxicity evaluation of a commercial herbal preparation used in Nigeria. Eur J Med Plant. 5(2):176–190.
- Esterbauer H, Schaur RJ, Zollner H. 1991. Chemistry and biochemistry of 4-hydroxynonenal, malonaldehyde and related aldehydes. Free Radic Biol Med. 11(1):81–128.
- Ezeanyika LUS, Ejike CECC, Obidoa O, Elom SO. 2006. Prostate disorders in an apparent normal Nigerian population: prevalence. Biokemistri. 18(2):127–132.
- Foo KT. 2017. Pathophysiology of clinical benign prostatic hyperplasia. Asian J Urol. 4(3):152–157.
- Fossati P, Prencipe L, Berti G. 1980. Use of 3, 5-dichloro- 2-hydroxybenzenesulfonic acid/4-ami nophenazone chromogenic system in direct enzymic assay of uric acid in serum and urine. Clin Chem. 26(2):227–231.
- Friedewald WT, Levy RI, Fredrickson DS. 1972. Estimation of low-density lipoprotein cholesterol in plasma, without use of the preparative ultracentrifuge. J Clin Chem. 18:499–502.
- Gasco M, Villegas L, Yucra S, Rubio J, Gonzales GF. 2007. Dose–response effect of Red Maca (Lepidium meyenii) on benign prostatic hyperplasia induced by testosterone enanthate. Phytomedicine. 14:460–464.
- Hagberg KW, Divan HA, Persson R, Nickel JC, Jick SS. 2016. Risk of erectile dysfunction associated with use of 5-α reductase inhibitors for benign prostatic hyperplasia or alopecia: population based studies using the Clinical Practice research Datalink. Br Med J. 354:i4823.
- Hassan AU, Hassan G, Zubeida ZR. 2013. Aims and objectives of histological studies of prostate. Uni J Clin Med. 1(2):13–21.
- Ikeyi AP, Ezeanyika LUS, Alumanah EO, Okagu IU. 2019. Proximate and phytochemical compositions, and toxicity studies on Zapoteca portoriscensis root methanol extract and its fractions. Pharmacologyonline. 2019(1):50–56.
- Iweala EEJ, Ogidigo JO. 2015a. Prostate specific antigen, antioxidant and hematological parameters in prostatic rats fed Solanum macrocarpon L. leaves. Asian J Biol Sci. 8:30–41.
- Iweala EEJ, Ogidigo JO. 2015b. Effect of Celosia argentea F. Cristata (L.) Schinz. on prostate specific antigen, antioxidant status and hematological parameters in rats induced with benign prostate hyperplasia. Asian J Biochem. 10:42–51.
- Joshua PE, Ezugwu CH, Chilaka FC, Nwodo OFC, Dasofunjo K, Ezugwu MU. 2018. Effect of ethanol extract of Zapoteca portoricensis stem on testosterone-induced benign prostate hyperplasia (BPH) in adult male albino rats. Australian J Basic Applied Sci. 12(12):9–18.
- King KJ, Wootton IDP. 1959. Microanalysis in medical biochemistry. New York, NY: McGrew Hill; p. 14.
- Krušlin B, Tomas D, Džombeta T, Milković-Periša M, Ulamec M. 2017. Inflammation in prostatic hyperplasia and carcinoma-basic scientific approach. Front Oncol. 7:77.
- Liu J, Fang T, Li M, Song Y, Li J, Xue Z, Li J, Bu D, Liu W, Zeng Q, et al. 2019. Pao pereira extract attenuates testosterone-induced benign prostatic hyperplasia in rats by inhibiting 5α-reductase. Sci Rep. 9:19703.
- Liu Y, Zuckier LS, Ghesani NV. 2010. Dominant uptake of fatty acid over glucose by prostate cells: a potential new diagnostic and therapeutic approach. Anticancer Res. 30:369–374.
- Martin SS, Blaha MJ, Elshazly MB, Brinton EA, Toth PP, McEvoy JW, Joshi PH, Kulkarni KR, Mize PD, Kwiterovich PO, et al. 2013. Friedewald-estimated versus directly measured low-density lipoprotein cholesterol and treatment implications. CME J Am Coll Cardiol. 62(8):732–739.
- Mbaka G, Anunobi C, Ogunsina S, Osiagwu D. 2017. Histomorphological changes in induced benign prostatic hyperplasia with exogenous testosterone and estradiol in adult male rats treated with aqueous ethanol extract of Secamone afzelii. Egyptian J Basic Applied Sci. 4:15–21.
- Mbaka GO, Ogbonnia SO, Olawunmi O. 2013. The effects of ethanol extract of Raphia hookeri seed on exogenous testosterone and estradiol induced benign prostatic hyperplasia in adult male rats. J Morphol Sci. 30(4):235–243.
- Meludu SC, Ezenwelu VI, Manafa PO, Onah CE, Ekuma-Okereke O. 2017. Biochemical characteristics of liver enzymes, prolactin, zinc and selenium in benign prostatic hyperplasia and cancer of the prostate patients attending urology clinic at Nnamdi Azikiwe University Teaching Hospital, Nnewi. IJIR. 3(7):223–232.
- Mohamed DA, Rashed MM, Shallan M, Fouda K, Hanna LM. 2016. Amelioration of benign prostate hyperplasia in rats through plant foods. Int J Pharmacogn Phytochem Res. 8(12):2063–2070.
- Moke EG, Ilodigwe EE, Okonta JM, Emudainohwo JOT, Ajaghaku DL, Erhirhie OE, Chinwuba P, Ahante E. 2015. Antidiabetic activity and toxicity evaluation of aqueous extracts of Spondias mombin and Costus afer on wistar rats. British J Pharm Res. 6(5):333–342.
- Nandecha C, Nahata A, Dixit VK. 2010. Effect of Benincasa hispida fruits on testosterone-induced prostatic hypertrophy in albino rats. Cur Therapeut Res. 71:331–343.
- Nandeesha H, Koner BC, Dorairajan LN, Sen SK. 2006. Hyperinsulinemia and dyslipidemia in non-diabetic benign prostatic hyperplasia. Clin Chim Acta. 370:89–93.
- Nwodo NJ, Okoye FBC, Lai D, Debbab A, Brun R, Proksch P. 2014. Two trypanocidal Dipeptides from the roots of Zapoteca portricensis (Fabaceae). Molecules. 19:5470–5477.
- Nwodo NJ, Uzochukwu IC. 2008. Studies on anti-inflammatory and antimicrobial activities of crude methanol extracts of Zapoteca portoricensis. Jacq. H. Hernandez. Rec Prog Med Plants. 19:61–69.
- Ochei J, Kolhatkar A. 2008. Medical laboratory sciences, theory and practice. New Delhi: Tata McGraw-Hill Publishing Co. Ltd; p. 321–324.
- Oh HY, Lee EJ, Yoon S, Chung BH, Cho KS, Hong SJ. 2007. Cholesterol level of lipid raft microdomains regulates apoptotic cell death in prostate cancer cells through EGFR-mediated Akt and ERK signal transduction. Prostate. 67:1061–1069.
- Park BK, Kim CW, Kwon JE, Negi M, Koo YT, Lee SH, Baek DH, Noh YH, Kang SC. 2019. Effects of Lespedeza cuneate aqueous extract on testosterone-induced prostatic hyperplasia. Pharm Biol. 57(1):90–98.
- Pudasaini S, Subedi N, Shrestha NM. 2019. Evaluation of prostate specific antigen levels and its correlation with histopathological findings. J Pathol Nepal. 9:1485–1489.
- Rastrelli G, Vignozzi L, Corona G, Maggi M. 2019. Testosterone and benign prostatic hyperplasia. Sex Med Rev. 7(2):259–271.
- Reitman S, Frankel D. 1957. A colometric method of determination of serum glutamic oxaloacetic and glutamic pyruvic transaminase. Am J Pathol. 28:56–62.
- Roehrborn CG. 2008. Pathology of benign prostatic hyperplasia. Int J Impot Res. 20:S11–S18.
- Roeschlau P, Bernt E, Gruber WA. 1974. Enzymatic determination of total cholesterol in serum. Z Klin Chem Klin Biochem. 12(5):226–228.
- Rule AD, Jacobson DJ, Roberts RO, Girman CJ, McGree ME, Lieber MM, Jacobsen SJ. 2005a. The association between benign prostatic hyperplasia and chronic kidney disease in community-dwelling men. Kidney Int. 67:2376–2382.
- Rule AD, Lieber MM, Jacobsen SJ. 2005b. Is benign prostatic hyperplasia a risk factor for chronic renal failure? J Urol. 173(3):691–696.
- Sinha KA. 1972. Colorimetric assay of catalase. Ann Biochem. 47(2):389–394.
- Steemkamp V. 2003. Phytomedicines for the prostate. Fitoterapia. 74(6):545–552.
- Sun H, Li TJ, Sun LN, Qiu Y, Huang BB, Yi B, Chen WS. 2008. Inhibitory effect of traditional Chinese medicine Zi-Shen Pill on benign prostatic hyperplasia in rats. J Ethnopharmacol. 115:203–208.
- Tewari R, Mohini C, Shankar MN, Apul G, Divakar D, Madhu M, Singh R. 2014. Significant association of metabolic indices, lipid profile, and androgen levels with prostate cancer. Asian Pac J Cancer Prev. 15(22):9841–9846.
- Tewari R, Prabhat P, Natu SM. 2011. Association of benign prostatic hyperplasia (BPH) with metabolic syndrome (MS) and its components: A growing dilemma. J Men’s Health. 8(2):66–71.
- Tietz NW. 1983. Methods for the determination of ascorbic acid. In: Kay D, Kilmer D, editor. Textbook of clinical chemistry. Philadelphia: W.B. Saunders Company; p. 932–933.
- Tietz NW. 1995. Clinical guide to laboratory test, 3rd ed. Philadelphia: W.B. Saunders Company; p. 518–519.
- Tietz NW, Pruden EL, Siggaard-Andersen O. 1976. Electrolytes, blood gases and acid-base balance. In: Tietz NW, editor. Textbook of clinical chemistry. Philadelphia: W.B. Saunders Company; p. 1188–1215.
- Trost L, Saitz TR, Hellstrom WJG. 2013. Side effects of 5-alpha reductase inhibitors: A comprehensive review. Sex Med Rev. 1(1):24–41.
- Ukwe CV, Ubaka CM, Adibe MO, Okonkwo CJ, Akah PA. 2010. Antiulcer activity of roots of zapoteca portoricensis (fam. fabiaceae). JBCP. 1(3):183–186.
- Veeresh BSV, Veeresh B, Patil AA, Warke YB. 2010. Lauric acid and myristic acid prevent testosterone induced prostatic hyperplasia in rats. Eur J Pharmacol. 626:262–265.
- Vyas N, Argal A. 2012. Nephroprotective effect of ethanolic extract of roots and oleanolic acid isolated from roots of Lantana camas. Int J Pharmacol Clin Sci. 1:54–60.
- Wallin B, Rosengren B, Shertzer HG, Camejo G. 1993. Lipoprotein oxidation and measurement of TBARS formation in single microlitre Figure, its use for evaluation of antioxidants. Anal Biochem. 208:10–15.