ABSTRACT
Favipiravir and remdesivir, inhibitors of RNA-dependent RNA polymerase (RdRp) were recently suggested as a potential SARS-CoV2 inhibitors. Since favipiravir and remdesivir target a critical and a viral-specific process, discovering other small molecules that do the same to use as treatment could be beneficial in slowing the outbreak. Though there have been many suggested antivirals to treat SARS-CoV-2 infection, most treatments target host-associated pathways that may cause adverse effects, Small molecules inhibitors of RdRp may be the best remedy against the COVID-19 pandemic.
Introduction
Coronavirus Disease-2019 (COVID-19) is the disease caused by the novel severe acute respiratory syndrome-coronavirus 2 (SARS-CoV-2). SARS-CoV-2 is a member of the coronavirus family recently discovered in Wuhan, Hubei, China (Zhu et al. Citation2020). The coronavirus family was initially discovered in the later part of the 1960s (Kahn and McIntosh Citation2005). Since the discovery there have been several members of this virus that have caused serious respiratory tract infections, these include Severe Acute Respiratory Syndrome (SARS-CoV) in 2003 (Smith Citation2006), Middle East Respiratory Syndrome (MERS-CoV) in 2012 (World Health Organization Citation2014), and the current outbreak SARS-CoV-2 (previously known as 2019-nCoV) (Zhu et al. Citation2020).
Coronaviruses (CoVs) belong to the Nidovirales order and got their names from the unique ‘crown- like’ spikes that are visible on the outer membrane of the virions (Li Citation2016). Coronaviruses are large single-stranded, enveloped RNA viruses that are broken down into four classes: Alphacoronavirus, Betacoronavirus, Gammacoronavirus, and Deltacoronavirus. Coronaviruses are the largest RNA viruses known with a genome ranging between 27 and 32 kb (Perlman and Netland Citation2009). Like all the Nidovirales members, Coronaviruses have a highly conserved genomic organization, unique enzymatic activities, and expression of nonstructural genes by ribosomal frameshifting.
Since the initial infection of SARS-CoV-2 in Wuhan, the virus has spread globally. Though the WHO did not declare COVID-19 a pandemic until March 2020, recent evidences suggests that SARS-CoV-2 was spreading in areas outside China as early as December 2019 (Deslandes et al. Citation2020). Although the mortality rate of COVID-19 is comparatively lower than previous coronavirus outbreaks (SARS-CoV-10% and MERS-CoV-37%) (Wu et al. Citation2020), it is much higher than the 2018–2019 influenza season (0.96%). The first case in the United States was confirmed on January 20, 2020 in Washington State (Holshue et al. Citation2020) and has since spread to every states and territory (Coronavirus Disease Citation2019 (COVID-Citation19) Citation2019). This outbreak has been devastating to older population (over 60) which are at higher risk and show more severe symptoms when compared with younger population (Velavan and Meyer Citation2020). As with other coronaviruses, SARS-CoV-2 infects the lung epithelial cells of the alveola and can lead to severe complications including pneumonia (Zhou et al. Citation2020). COVID-19 has caused a global shut down of commerce and normalcy with governments calling for social distancing and even lockdown of entire cities and countries. It is thus important for the discovery of treatments for this novel virus to slow the outbreak and shorten the disease.
Viral components and life cycle
SARS-CoV-2 is an enveloped single-stranded positive-sense RNA virus composed of four structural proteins, envelope (E), membrane (M), spike (S), and nuclear capsid (N) (Chen et al. Citation2020) (Figure ). In addition to the structural proteins, the genome contains a 5’ cap structure along with a 3’ poly (A) tail that acts as an mRNA for translation of the replicase polyproteins. The replicase gene encodes the nonstructural proteins (NSPS) that form two-thirds of the genome. The genome also includes several accessory proteins that are not essential for viral replication but were shown to play a role in viral pathogenesis.
Figure 1. Schematic presentation of the COVID-19 structure. Structural proteins of SARS-CoV-2 where the location and names of the viral proteins are shown (S = spike; N = nucleocapside; M = membrane; E = Envelope).
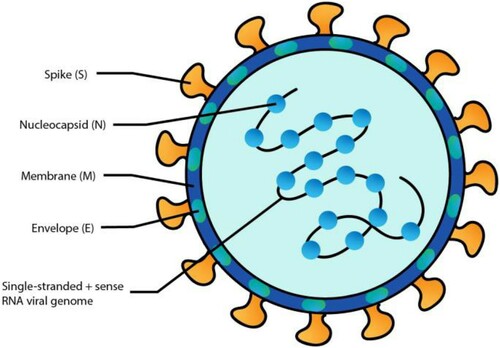
Even though, the E protein is not conserved between coronaviruses, however it is important for assembly and release of the virus. The M protein gives the virion its spherical shape and is the most abundant protein. The S protein has been shown to be important in host cell interactions and virulence (Yan et al. Citation2020). The N protein binds to the single-strand RNA inside the capsid to protect it in a beads-on-a-string conformation. SARS-CoV-2 differs from other coronaviruses because of its S protein. The S1 domain of the S protein has been shown to have a 45% difference in the amino acid sequence compared to that of SARS-CoV (Vankadari and Wilce Citation2020).
It has been found that the virus enters the body via droplets that travel to the respiratory tract, where it can infect cells containing angiotensin-converting enzyme 2 (ACE2) including lung epithelial cells (Li et al. Citation2020). The S1 domain of the S protein has been shown to interact with ACE2 on the surface of cells (Yan et al. Citation2020). The S protein has also been predicted to interact with the Glucose Regulated Protein 78 (GRP78) (Ibrahim et al. Citation2020). After binding to a receptor on the cell surface, the S protein is cleaved by a cellular protease: cathepsin (Fehr and Perlman Citation2015) or TMPRSS2 (Hoffmann et al. Citation2020) to expose the fusion peptide allowing the viral membrane to fuse with the cellular membrane. The viral genome is then released into the cytoplasm. Next, the host ribosomes translate the viral positive-sense RNA into 16 nonstructural proteins (NSPS) (Chen et al. Citation2020). Some of these NSPS are involved in ensuring viral RNA is translated efficiently without host interference. Other NSPS are important for producing mature virions. The rest of the NSPS make the replicase-transcriptase complex (RTC). The RTC is composed of proteins that are required to replicate viral RNA both genomic and subgenomic through anti-sense RNA intermediates (Chen et al. Citation2020).
After viral genomic RNA replication and viral protein translation from subgenomic RNA, the viral structural proteins are inserted into the endoplasmic reticulum (ER). After this insertion, the proteins follow the secretory pathway and form the endoplasmic reticulum-Golgi intermediate compartment (ERGIC) (Hoffmann et al. Citation2020). Once the ERGIC is formed the viral RNA genomes, which are encapsulated by the N protein, are incorporated into the ERGIC which then buds into mature virions. The mature virions exit the cell via exocytosis and can infect other cells or be spread to other hosts.
Viral RNA replication as a therapeutic target
Currently, clinicians can only treat the various symptoms of COVID-19 because there is no targeted treatment for the SARS-CoV-2. Nevertheless, drugs used to treat Malaria and HIV-1 such as chloroquine and lopinavir/ritonavir, respectively, have been shown to possibly influence SARS-CoV-2 replication (Dong et al. Citation2020).
Hydroxychloroquine has been shown to inhibit the glycosylation of ACE2 and thus prevent SARS-CoV-2 from binding to cells (Savarino et al. Citation2006). Studies revealed no cytotoxicity in vitro, however the glycosylation of ACE2 is important in the regulation of the renin-angiotensin system (Warner et al. Citation2005). Deregulation of this system could cause some adverse effects.
Similarly, lopinavir/ritonavir is a protease inhibitor that could block the cleavage of the S protein of SARS-CoV-2 thus preventing fusion of the viral and cellular membranes (Cao et al. Citation2020). However, there are many known adverse effects of this drug and it has shown no significant improvements over standard care in treating COVID-19.
Ideally, it is better to target a step of the virus life cycle preventing it from forming mature virions and infecting other cells without damaging host cell function that could cause adverse side-effects. In order to reduce adverse effects, viral-specific processes can be inhibited or reversed. SARS-CoV-2 relies on host cellular mechanisms to reproduce mature virions. However, RNA replication from an RNA template is unique to the virus thus making this step a possible therapeutic target. Inhibiting the virus at the RNA replication step would result in (1) no genomic RNA replication, (2) no subgenomic replication and subsequent structural protein production, and (3) no mature virion formation and release essentially stopping the productive infection. By only targeting the RNA replication of the virus, NSPS can still be produced at low levels inside the cell but infection would end there. A possible drug candidate (small molecules such as favipiravir and remdesivir) that inhibits the RNA-dependent RNA polymerase (RdRp) that would result in the inhibition of SARS-CoV-2 is/are needed. With the exception of small number of studies, most research papers focusing on small molecules with the ability to inhibit SARS-CoV-2 replication were performed in silico (Tiwari et al. Citation2020).
Favipiravir
Favipiravir (6-fluoro-3-hydroxy-2-pyrazinecarboxamide) also known as T-705, avigan or favilavir, is an antiviral agent that was first developed by Toyama Chemical Co. against the flu (Furuta et al. Citation2002). Favipiravir is a pyrazinecarboxamide derivative that can inhibit the RdRp of influenza virus and has also been shown to have antiviral activities beyond the flu. In animals, favipiravir has shown activity against viruses such as Influenza, West Nile, Yellow fever, Foot and Mouth Disease, and Rift Valley as well as other Flaviviruses, Arenaviruses, Bunyaviruses, Alphaviruses (e.g. Chikungunya [CHIKV]; Sindbis [SINV]; Western equine encephalitis [WEEV]; and Semliki Forest [SFV] viruses), and Enteroviruses (Furuta et al. Citation2009; Furuta et al. Citation2013; Caroline et al. Citation2014). Favipiravir has been shown to have an effect in vitro and in vivo against Zaire Ebola (Kerber et al. Citation2019), Rabies (RABV) (Yamada et al. Citation2015) and Zika viruses (Cai et al. Citation2017). Favipiravir was described to reduce the morbidity and mortality associated with RABV infection in mice. In a Syrian hamster model that mirrors the human disease, favipiravir reduced encephalitis, hemorrhagic fever, respiratory difficulties and mortality rate caused by Nipah virus infection (a Bat virus) (Dawes et al. Citation2018).
Favipiravir is structurally similar to ribavirin (antiviral drug used to treat Respiratory Syncytial Virus [RSV] infection, Hepatitis C, and some viral hemorrhagic fevers) (Drabikowska et al. Citation1979). Favipiravir and ribavirin share a carboxamide (C-[O]-NH2) moiety, however favipiravir is a more specific version of ribavirin. Both drugs target the viral RNA polymerase; ribavirin primarily targets the Inosine-5′-monophosphate dehydrogenase (IMPDH), while favipiravir interacts with RNA polymerase.
Mechanistically, favipiravir is a prodrug that does not inhibit influenza RNA polymerase activity until it is phosphoribosylated in cells forming favipiravir-ribofuranosyl-50-triphosphate (favipiravir-RTP) (Furuta et al. Citation2013). Favipiravir-RTP then binds to the active site of RdRp to stop RNA replication. It has been suggested that the human hypoxanthine guanine phosphoribosyl-transferase (HGPRT) plays a role in the favipiravir activation (Naesens et al. Citation2013). The active form of favipiravir is recognized by the catalytic domain of the viral RNA-dependent RNA polymerase and blocks its enzymatic activity. This results in inhibition of the RNA-dependent RNA polymerase and effectively ending the infectious cycle of SARS-CoV-2 (Figure ). Note that favipiravir is not toxic to mammalian cells and does not inhibit RNA or DNA synthesis within these cells (Furuta et al. Citation2002).
Figure 2. SARS-CoV-2 life cycle and RdRp inhibitor: mechanism of action. Numbers in black indicate the normal life cycle of SARS-CoV-2 from (1) binding to ACE2 receptors and then fusion with the cellular membrane, (2) release of the viral genome in the form of (+) sense RNA and the subsequent production of nonstructural proteins (NSPS), (3) translation and formation of the replicase-transcriptase complex (RTC) including RdRp and RNA replication, (4) transcription of genomic and subgenomic RNA, (5) translation of that RNA into structural proteins, (6) nucleocapsid combination with S, E, and M proteins in the ERGIC, (7) formation a mature virion, and (8) exocytosis of mature virions. Numbers in red indicate the mechanism of action of RdRp inhibitors from (1) entry into the cell in the inactive form, (2) cell metabolism into the active form, and (3) binding to RdRp to block its active site and stop RNA replication.
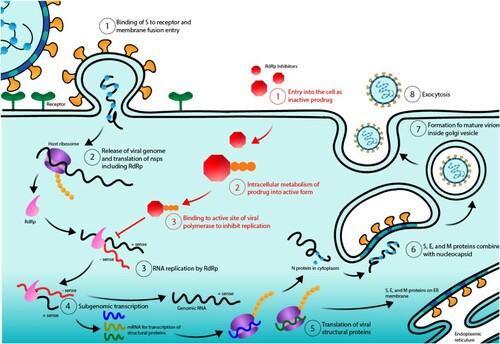
All these features make favipiravir a broad-spectrum inhibitor of RdRp, the polymerase responsible for replicating RNA and the major functional unit of the RTC in SARS-CoV2 making this drug one of the best available drug to fight the spread of COVID-19.
Currently, favipiravir has been approved in Japan as an influenza treatment and has been shown to be among the efficient treatment against COVID-19 disease in Wuhan, China (Dong et al. Citation2020). A clinical trial in China assessed the antiviral ability of favipiravir and has shown a reduction in viral clearance time in 80 patients to four days compared to eleven days for the control group (Chinese Clinical Trial registry ChiCTR2000029600) (Cai et al. Citation2020; Li and De Clercq Citation2020). Even though, the study lacked controls for bias, 91.43% of patients had improved CT scans with few side-effects. These results encouraged other countries such as Turkey to start using favipiravir as a potential treatment against COVID-19 disease.
Remdesivir
Remdesivir (GS-5734) is another RdRp inhibitor that was recently approved by FDA and carries the same feature as favipiravir. Developed by Gilead Sciences in 2009 as a Hepatitis C drug (although it failed to affect Hep C), then used as a potential drug against Ebola and Marburg viruses’ diseases (Warren et al. Citation2016).
Remdesivir enters the cells as an inactive prodrug and then is processed intracellularly to produce the active nucleoside monophosphate (nucleotide analogue) form (Eastman et al. Citation2020). This form is an ATP analogue that gets incorporated into the RNA strand and binds the RdRp’s active site thus preventing the next RNA subunit from being incorporated (Huang et al. Citation2020) (Figure ). The drug was able to block Ebola virus in Rhesus macaques (Warren et al. Citation2016) and a phase 3 clinical trial to assess the efficacy of remdesivir as well as other antivirals in human has been completed in 2019 (ClinicalTrial.gov NCT03719586). The primary end point in this study was mortality. Remdesivir was found to have a mortality rate above 50% – higher that the other therapies tested (Mulangu et al. Citation2019). Researchers suggested that a high mortality rate could be due to the fact that remdesivir was described to patients with advance or severe disease or because it was administered to patients over multiple days compared to other drugs given as a single infusion on Day 1.
Remdesivir is now being looked at as a potential antiviral against SARS-CoV-2. It was evaluated in vitro along with chloroquine in SARS-CoV2-infected Vero-E6 cells and has shown to significantly reduce the viral copy number within 48 h (Wang et al. Citation2020).
In April 2020, remdesivir was approved by FDA to be used against COVID-19. However, remdesivir antiviral efficacy was assessed in a phase 3 clinical trial that started in February 2020 (ClinicalTrial.gov NCT04280705). The trial also known as ACTT-1 enrolled patients from 60 sites spread between US, Denmark, UK, Greece, Germany, Mexico, Spain, Japan and Singapore. The study lasted 29 days and preliminary results showed that the recovery time was shorter (median 11 days) for patients taken remdesivir compared to those taking the placebo (median 15 days) (Beigel et al. Citation2020). Note that the study only enrolled hospitalized patients with severe COVID-19 (oxygen saturation: SpO2 ≤ 94%).
In a different clinical trial, remidesivir was administered for five and ten days with no placebo (ClinicalTrial.gov NCT04292899). The study found no difference in recovery between the two time (Goldman et al. Citation2020). Overall, remdesivir showed some potential in reducing recovery time in patients diagnosed with severe disease but failed to reduce mortality rate, despite its significant effect on animal infected with SARS-CoV-2 and MERS, which lead to suggest that it should be used in conjunction with other therapies (Beigel et al. Citation2020).
Conclusion and future directions
COVID-19 caused by the novel coronavirus, SARS-CoV-2, spreads quickly across the globe. Molecules with the ability to inhibit RdRp are an ideal targeted treatment since they could be viral-specific and have already been used in clinics. Modified favipiravir and remdesivir or any potential molecule(s) that target and block viral RNA-dependent RNA polymerase (RdRp) – used by the virus to replicate and produce mature virions – should be considered for future treatment. Blocking this step in the viral life cycle won’t prevent infection but will shorten the duration and reduce the severity. It may also shorten the time a patient is contagious. New/ongoing clinical trials need to be completed to further explore the possibility of new inhibitors of RdRp as an effective treatment for the spread and severity of SARS-CoV-2 as current research seems promising that a modified favipiravir (Dehelean et al. Citation2020) and/or remdesivir (Gordon et al. Citation2020; Pruijssers et al. Citation2020) could be useful treatments.
In conclusion, inhibitors of RdRp have great potential to be effective in treating COVID-19 and should be fast-tracked into clinical trials in the US. Such drugs can shorten infection and will help reduce the number of fatalities that SARS-CoV-2 is estimated to cause in the near future during any ‘second wave’ and further outbreaks.
Acknowledgements
This work is supported by an NIH-NIA (R01-AG054411) awarded to BES.
Disclosure statement
No potential conflict of interest was reported by the author(s).
Data availability statement (DAS)
The data that support the findings of this study are available in pubmed.ncbi.nlm.nih.gov and BioRxiv.org that issue datasets with DOIs.
Additional information
Funding
References
- Beigel JH, Tomashek KM, Dodd LE, Mehta AK, Zingman BS, Kalil AC, Hohmann E, Chu HY, Luetkemeyer A, Kline S, Lopez de Castilla D, Finberg RW, Dierberg K, Tapson V, Hsieh L, Patterson TF, Paredes R, Sweeney DA, Short WR, Touloumi G, Lye DC, Ohmagari N, Oh MD, Ruiz-Palacios GM, Benfield T, Fätkenheuer G, Kortepeter MG, Atmar RL, Creech CB, Lundgren J, Babiker AG, Pett S, Neaton JD, Burgess TH, Bonnett T, Green M, Makowski M, Osinusi A, Nayak S, Lane HC. 2020. ACTT-1 Study Group Members. Remdesivir for the Treatment of Covid-19 - Final Report. N Engl J Med. 383:992–994. doi: https://doi.org/10.1056/NEJMc2022236
- Cai L, Sun Y, Song Y, Xu L, Bei Z, Zhang D, Dou Y, Wang H. 2017. Viral polymerase inhibitors T-705 and T-1105 are potential inhibitors of Zika virus replication. Arch Virol. 162:2847–2853. doi: https://doi.org/10.1007/s00705-017-3436-8
- Cai Q, Yang M, Liu D, Chen J, Shu D, Xia J, Liao X, Gu Y, Cai Q, Yang Y, et al. 2020. Experimental treatment with favipiravir for COVID-19: an open-label control study. Engineering (Beijing) Online.
- Cao B, Wang Y, Wen D, Liu W, Wang J, Fan G, Ruan L, Song B, Cai Y, Wei M, et al. 2020. A trial of lopinavir-ritonavir in Adults hospitalized with severe covid-19. N Engl J Med. 382:1787–1799. doi: https://doi.org/10.1056/NEJMoa2001282
- Caroline AL, Powell DS, Bethel LM, Oury TD, Reed DS, Hartman AL. 2014. Broad spectrum antiviral activity of favipiravir (T-705): protection from highly lethal inhalational rift valley fever. PLoS Negl Trop Dis. 8:e2790. doi: https://doi.org/10.1371/journal.pntd.0002790
- Chen Y, Liu Q, Guo D. 2020. Emerging coronaviruses: genome structure, replication, and pathogenesis. J Med Virol. 92:418–423. doi: https://doi.org/10.1002/jmv.25681
- Coronavirus Disease 2019 (COVID-19). 2019. Centers for disease control and prevention. Centers for Disease Control and Prevention.
- Dawes BE, Kalveram B, Ikegami T, Juelich T, Smith JK, Zhang L, Park A, Lee B, Komeno T, Furuta Y, Freiberg AN. 2018. Favipiravir (T-705) protects against Nipah virus infection in the hamster model. Sci Rep. 8:7604. doi: https://doi.org/10.1038/s41598-018-25780-3
- Dehelean CA, Lazureanu V, Coricovac D, Mioc M, Oancea R, Marcovici I, Pinzaru I, Soica C, Tsatsakis AM, Cretu O. 2020. SARS-CoV-2: Repurposed drugs and novel therapeutic approaches-insights into chemical structure-biological activity and toxicological screening. J Clin Med. 9:2084. doi: https://doi.org/10.3390/jcm9072084
- Deslandes A, Berti V, Tandjaoui-Lambotte Y, Alloui C, Carbonnelle E, Zahar JR, Brichler S, Cohen Y. 2020. SARS-Cov-2 was already spreading in France in late December 2019. Int. J. Antimicrob Agents. 55:106006. doi: https://doi.org/10.1016/j.ijantimicag.2020.106006
- Dong L, Hu S, Gao J. 2020. Discovering drugs to treat coronavirus disease 2019 (COVID-19). Drug Discov Ther. 14:58–60. doi: https://doi.org/10.5582/ddt.2020.01012
- Drabikowska AK, Dudycz L, Shugar D. 1979. Studies on the mechanism of antiviral action of 1-(beta-D-ribofuranosyl)-1,2,4-triazole-3-carboxamide (ribavirin). J Med Chem. 22:653–657. doi: https://doi.org/10.1021/jm00192a009
- Eastman RT, Roth JS, Brimacombe KR, Simeonov A, Shen M, Patnaik S, Hall MD. 2020. Remdesivir: a review of its discovery and development leading to emergency use authorization for treatment of COVID-19. ACS Cent Sci. 6:672–683. doi: https://doi.org/10.1021/acscentsci.0c00489
- Fehr AR, Perlman S. 2015. Coronaviruses: an overview of their replication and pathogenesis. Methods Mol Biol. 1282:1–23. doi: https://doi.org/10.1007/978-1-4939-2438-7_1
- Furuta Y, Gowen BB, Takahashi K, Shiraki K, Smee DF, Barnard DL. 2013. Favipiravir (T-705), a novel viral RNA polymerase inhibitor. Antiviral Res. 100:446–454. doi: https://doi.org/10.1016/j.antiviral.2013.09.015
- Furuta Y, Takahashi K, Fukuda Y, Kuno M, Kamiyama T, Kozaki K, Nomura N, Egawa H, Minami S, Watanabe Y, et al. 2002. In vitro and in vivo activities of anti-influenza virus compound T-705. Antimicrob Agents Chemother. 46:977–981. doi: https://doi.org/10.1128/AAC.46.4.977-981.2002
- Furuta Y, Takahashi K, Shiraki K, Sakamoto K, Smee DF, Barnard DL, Gowen BB, Julander JG, Morrey JD. 2009. T-705 (favipiravir) and related compounds: novel broad-spectrum inhibitors of RNA viral infections. Antiviral Res. 82:95–102. doi: https://doi.org/10.1016/j.antiviral.2009.02.198
- Goldman JD, Lye DC, Hui DS, Marks KM, Bruno R, Monetjano R, Spinner CD, Galli M, Ahn MY, Nahass RG, et al. 2020. Remdesivir for 5 or 10 days in patients with severe Covid-19. N Engl J Med. Online. https://doi.org/https://doi.org/10.1056/NEJMoa2015301.
- Gordon CJ, Tchesnokov EP, Woolner E, Perry JK, Feng JY, Porter DP, Götte M. 2020. Remdesivir is a direct-acting antiviral that inhibits RNA-dependent RNA polymerase from severe acute respiratory syndrome coronavirus 2 with high potency. J Biol Chem. 295:6785–6797. doi: https://doi.org/10.1074/jbc.RA120.013679
- Hoffmann M, Kleine-Weber H, Schroeder S, Krüger N, Herrler T, Erichsen S, Schiergens TS, Herrler G, Wu N-H, Nitsche A, et al. 2020. SARS-CoV-2 cell entry depends on ACE2 and TMPRSS2 and is blocked by a clinically proven protease inhibitor. Cell. 181:271–280. doi: https://doi.org/10.1016/j.cell.2020.02.052
- Holshue ML, Debolt C, Lindquist S, Lofy KH, Wiesman J, Bruce H, Spitters C, Ericson K, Wilkerson S, Tural A, et al. 2020. First case of 2019 novel coronavirus in the United States. N Engl J Med. 382:929–936. doi: https://doi.org/10.1056/NEJMoa2001191
- Huang J, Song W, Huang H, Sun Q. 2020. Pharmacological therapeutics targeting RNA-dependent RNA polymerase, proteinase and spike protein: from mechanistic studies to clinical trials for COVID-19. J. Clin. Med. 9:1131. doi: https://doi.org/10.3390/jcm9041131
- Ibrahim IM, Abdelmalek DH, Elshahat ME, Elfiky AA. 2020. COVID-19 spike-host cell receptor GRP78 binding site prediction. J Infect. 80:554–562. doi: https://doi.org/10.1016/j.jinf.2020.02.026
- Kahn JS, McIntosh K. 2005. History and recent advances in coronavirus discovery. Pediatr Infect Dis J. 24:S223–S227. discussion S226. doi: https://doi.org/10.1097/01.inf.0000188166.17324.60
- Kerber R, Lorenz E, Duraffour S, Sissoko D, Rudolf M, Jaeger A, Cisse SD, Camara AM, Miranda O, Castro CM, et al. 2019. Laboratory findings, compassionate use of Favipiravir, and outcome in patients with Ebola virus disease, Guinea, 2015-A retrospective observational study. J Infect Dis. 220:195–202. doi: https://doi.org/10.1093/infdis/jiz078
- Li F. 2016. Structure, function, and evolution of coronavirus spike proteins. Annu Rev Virol. 3:237–261. doi: https://doi.org/10.1146/annurev-virology-110615-042301
- Li G, De Clercq E. 2020. Therapeutic options for the 2019 novel coronavirus (2019-nCoV). Nat Rev Drug Discov. 19:149–150. doi: https://doi.org/10.1038/d41573-020-00016-0
- Li G, Fan Y, Lai Y, Han T, Li Z, Zhou P, Pan P, Wang W, Hu D, Liu X, et al. 2020. Coronavirus infections and immune responses. J Med Virol. 92:424–432. doi: https://doi.org/10.1002/jmv.25685
- Mulangu S, Dodd LE, Davey RT, Mbaya OT, Proschan M, Mukadi D, Manzo ML, Nzolo D, Oloma AT, Ibanda A, et al. 2019. A randomized, controlled trial of ebola virus disease therapeutics. N Engl J Med. 381:2293–2303. doi: https://doi.org/10.1056/NEJMoa1910993
- Naesens L, Guddat LW, Keough DT, van Kuilenburg AB, Meijer J, Vande Voorde J, Balzarini J. 2013. Role of human hypoxanthine guanine phosphoribosyltransferase in activation of the antiviral agent T-705 (favipiravir). Mol Pharmac. 84:615–629. doi: https://doi.org/10.1124/mol.113.087247
- Perlman S, Netland J. 2009. Coronaviruses post-SARS: update on replication and pathogenesis. Nat Rev Microbiol. 7:439–450. doi: https://doi.org/10.1038/nrmicro2147
- Pruijssers AJ, George AS, Schäfer A, Leist SR, Gralinksi LE, Dinnon K3, Yount BL, Agostini ML, Stevens LJ, Chappell JD, et al. 2020. Remdesivir inhibits SARS-CoV-2 in human lung cells and chimeric SARS-CoV expressing the SARS-CoV-2 RNA polymerase in mice. Cell Rep. 32:107940. doi: https://doi.org/10.1016/j.celrep.2020.107940
- Savarino A, Trani LD, Donatelli I, Cauda R, Cassone A. 2006. New insights into the antiviral effects of chloroquine. Lancet Infect Dis. 6:67–69. doi: https://doi.org/10.1016/S1473-3099(06)70361-9
- Smith RD. 2006. Responding to global infectious disease outbreaks: lessons from SARS on the role of risk perception, communication and management. Soc Sci Med. 63:3113–3123. doi: https://doi.org/10.1016/j.socscimed.2006.08.004
- Tiwari V, Beer JC, Sankaranarayanan NV, Swanson-Mungerson M, Desai UR. 2020. Discovering small-molecule therapeutics against SARS-CoV-2. Drug Discov Today. 25:1535–1544. doi: https://doi.org/10.1016/j.drudis.2020.06.017
- Vankadari N, Wilce JA. 2020. Emerging WuHan (COVID-19) coronavirus: glycan shield and structure prediction of spike glycoprotein and its interaction with human CD26. Emerg Microbes Infect. 9:601–604. doi: https://doi.org/10.1080/22221751.2020.1739565
- Velavan TP, Meyer CG. 2020. The COVID-19 epidemic. Trop Med Int Health. 25:278–280. doi: https://doi.org/10.1111/tmi.13383
- Wang M, Cao R, Zhang L, Yang X, Liu J, Xu M, Shi Z, Hu Z, Zhong W, Xiao G. 2020. Remdesivir and chloroquine effectively inhibit the recently emerged novel coronavirus (2019-nCoV) in vitro. Cell Res. 30:269–271. doi: https://doi.org/10.1038/s41422-020-0282-0
- Warner FJ, Lew RA, Smith AI, Lambert DW, Hooper NM, Turner AJ. 2005. Angiotensin-converting enzyme 2 (ACE2), But Not ACE, is preferentially localized to the apical surface of polarized kidney cells. J Biol Chem. 280:39353–39362. doi: https://doi.org/10.1074/jbc.M508914200
- Warren TK, Jordan R, Lo MK, Ray AS, Mackman RL, Soloveva V, Siegel D, Perron M, Bannister R, Hui HC, et al. 2016. Therapeutic efficacy of the small molecule GS-5734 against Ebola virus in rhesus monkeys. Nature. 531:381–385. doi: https://doi.org/10.1038/nature17180
- World Health Organization. 2014. Case-control study to assess potential risk factors related to human illness caused by the Middle East Respiratory Syndrome Coronavirus (MERS-CoV). 2014-03-28.
- Wu D, Wu T, Liu Q, Yang Z. 2020. The SARS-CoV-2 outbreak: what we know. Int J Infect Dis pii:. S1201-9712(20):30123–5.
- Yamada K, Noguchi K, Komeno T, Furuta Y, Nishizono A. 2016. Efficacy of favipiravir (T-705) in Rabies Postexposure Prophylaxis. J Infect Dis. 213:1253–1261. doi: https://doi.org/10.1093/infdis/jiv586
- Yan R, Zhang Y, Li Y, Xia L, Guo Y, Zhou Q. 2020. Structural basis for the recognition of the SARS-CoV-2 by full-length human ACE2. Science pii: eabb2762.
- Zhou P, Yang X-L, Wang X-G, Hu B, Zhang L, Zhang W, Si H-R, Zhu Y, Li B, Huang C-L, et al. 2020. A pneumonia outbreak associated with a new coronavirus of probable bat origin. Nature. 579:270–273. doi: https://doi.org/10.1038/s41586-020-2012-7
- Zhu N, Zhang D, Wang W, Li X, Yang B, Song J, Zhao X, Huang B, Shi W, Lu R, et al. 2020. A novel coronavirus from patients with pneumonia in China, 2019. N Engl J Med. 382:727–733. doi: https://doi.org/10.1056/NEJMoa2001017