Abstract
The sampling of cerebrospinal fluid (CSF) for chemical analysis is usually performed by a freehand lumbar puncture in a routine clinical procedure, while the suboccipital region is used for postmortem examinations. We introduce a new application for a semiautomated robotic, computed tomography (CT)-guided needle placement for postmortem lumbar and suboccipital CSF sampling. Postmortem computed tomography (PMCT) imaging was performed, and the data were sent to a custom-made Virtopsy Control Center and Virtopsy Planning and Navigation Station. Needle trajectories were planned for both suboccipital and lumbar approaches. Coaxial introducer needles were fully inserted automatically by the robotic system. PMCT was repeated to verify correct placement of the needles. Subsequently, CSF samples were taken using a syringe. By using this new application, the puncture of the spinal canal and cerebellomedullary cistern could be accurately performed to within a millimeter. Thus, failure of puncture and iatrogenic injury of the medulla oblongata may be prevented, and the risk of infection is significantly reduced. An early analysis of the CSF sample allows for a risk assessment in regard to the subsequent autopsy. Our application allows for minimally invasive, fast, efficient and safe CSF sampling prior to autopsy, enabling an analysis for metabolic imbalance, infection, etc.
Introduction
Whole-body postmortem computed tomography (PMCT) has already become a valuable addition and, in specific cases, even an alternative to autopsy in most Western institutes of forensic medicine (Ampanozi et al. Citation2019; Thali Citation2011; Thali et al. Citation2007; Dirnhofer et al. Citation2006; Thali et al. Citation2003).
PMCT data can be used along with a multifunctional robotic system for a semiautomated postmortem needle placement (Ebert et al. Citation2014; Ebert et al. Citation2012; Ebert et al. Citation2010). The placement of biopsy needles based on PMCT data has been shown to work with an accuracy of 1–3 mm in studies with phantoms (Ebert et al. Citation2014; Ebert et al. Citation2010). Among other tasks, the system has been used to extract tissue and liquid samples for toxicological examination (Brockbals et al. Citation2018; Staeheli et al. Citation2017).
The sampling of cerebrospinal fluid (CSF) for the analysis of cells, bacteria and other substances in a routine clinical analysis to diagnose infections, metabolic imbalances and other diseases is usually performed via a lumbar puncture, whereas in a postmortem diagnosis, the CSF is taken from the cerebellomedullary cistern (suboccipital). Both methods are usually performed freehand and without prior imaging. Only anatomical landmarks and haptic information from the tip of the needle are used for basic orientation. The discharge of a drop of CSF from the back of the needle verifies the correct placement. Therefore, the individual experience of a physician performing this intervention highly influences sampling success.
One drawback of postmortem CSF sampling is the risk of puncturing too deep with iatrogenic lesions of the medulla oblongata, potentially resulting in a misinterpretation of findings at autopsy. In addition, the risk of infection from the examined bodies is often unknown prior to autopsy. That means special personal protective measures, such as those used in a case of meningococcal sepsis, may be neglected, thus exposing both the autopsy technician and forensic pathologist to a high risk of infection.
An alternative to freehand needle placement is the use of CT-guided needle placement by hand, similar to procedures established in clinical radiology (for example in nerve root infiltration). Depending on the anatomical conditions of the subject, this procedure can still be time-consuming, requires experience and, depending on the technique used, causes radiation hazard to the personnel during the sampling procedure. These concerns might also apply to the potential use of fluoroscopy in this setting.
In this article, we introduce a new application for the Virtobot, a semiautomated robotic, CT-guided needle placement for postmortem CSF sampling lumbar and suboccipital. This application may significantly improve postmortem diagnosis, especially in terms of safety issues (risk of infection), efficiency (time and costs) and accuracy (independent of the operators’ experience).
Material and methods
Case
An 80-year-old male was found dead at the base of his cellar stairs and referred to the Institute of Forensic Medicine for the determination of cause and manner of death (exclusion of third-party involvement). Due to his preexisting condition of diabetes, a possible cause of death was considered to be a metabolic imbalance.
Postmortem computed tomography (PMCT)
PMCT imaging was performed using a 128-slice CT scanner (SOMATOM Definition Flash, Siemens Medical Solutions, Forchheim, Germany). The parameters were a 120 kVp tube voltage with an automated dose modulation (CAREdose4DTM, Siemens, Forchheim, Germany) (Flach et al. Citation2014). For planning of the needle placement, a scan was performed with a slice thickness of 1.5 mm.
Virtobot
We used the second prototype of the Virtobot (Figure ) with a specific tool changing unit (Schunk SWS40, SCHUNK GmbH & Co. KG, Lauffen/Neckar, Germany) and a custom-made biopsy module (Austrian Center for Medical Innovation and Technology Gmbh, Wiener Neustadt, Austria) (Ebert et al. Citation2010). Trajectory planning and execution were conducted using a custom-made Virtopsy Control Center (VCC) and Virtopsy Planning and Navigation Station (Ebert et al. Citation2014). The VCC was used for robot control, coordinating the workflow and general data handling. The Virtopsy Planning and Navigation Station was used for trajectory planning using the PMCT data. The trajectories established during the planning were then returned to the VCC for robot control.
Figure 1 The Virtobot positioned above the CT gantry. The biopsy tool mounted to the robotic arm is located immediately above the dummy (red arrow).
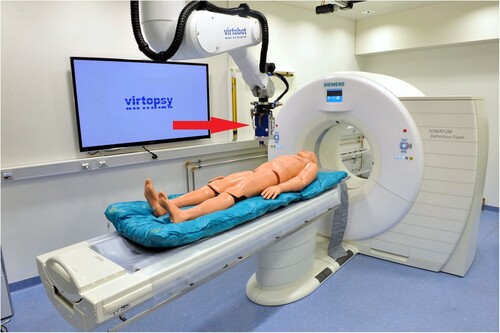
Coaxial introducer needles (13G, 10.3 cm) (BARD® Biopsy, Tempe, Arizona, USA) were used with the above system.
Workflow
Initial PMCT was performed with the body in a prone position and started head-first. The acquired PMCT data were sent to the Virtopsy Planning and Navigation Station.
In contrast to the procedure described by Ebert et al. (Citation2014), registration was not performed using optical radiodense markers but by registering a predefined table position using a phantom. This approach was both faster and less prone to errors because the table position had to be calibrated only once. The disadvantage was that the table position during needle placement could not be adjusted.
Both needle trajectories were planned for both the suboccipital and lumbar approaches (Figure ). After verification of the planned positions, the needles were fully inserted automatically by the robotic system. PMCT was repeated to verify the correct placement of the needles and measure the placement error (Figure ). Subsequently, CSF samples were taken using a syringe (Figure ).
Figure 2 PMCT of our case in the prone position: (a) Sagittal reconstruction with the red line representing the desired suboccipital access; (b) Axial reconstruction with the red line representing the desired lumbar access.
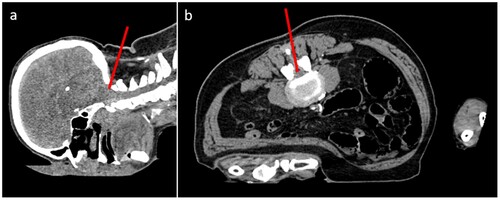
Figure 3 (a)–(c): 3D reconstruction of the intervention as planned in the Planning and Navigation Station, gray-level mapping adjusted manually to a bone window for better visualization. The white lines represent the needle placement as planned by the robotic system: (a) overview, (b) close-up for suboccipital sampling and (c) lumbar sampling. (d) Sagittal reconstruction of the postinterventional CT shows the correct placement of the suboccipital needle.
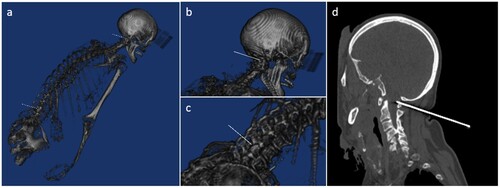
Discussion
We successfully performed the first CT-guided, robotic needle placement for postmortem CSF sampling. By using this new application, the puncture of the spinal canal and the cerebellomedullary cistern can be accurately performed to within a millimeter. Puncture failure as well as iatrogenic injury of the medulla oblongata may thereby be prevented. Furthermore, since no person needs to be present inside the CT room during robotic needle placement, so the risk of infection for autopsy technicians or forensic pathologists is also significantly reduced. Additionally, the early analysis of the CSF sample allows for risk assessment (i.e. meningococcal meningitis) in regard to the subsequent autopsy. Finally, this robotic, CT-guided needle placement is widely independent of the examiners’ experience in CSF sampling. Only the sampling position of the needle has to be chosen correctly, while the calculation of the needle trajectory and the placement of the needle is performed automatically. As shown in prior studies, Virtobot may also be operated by a technician via remote access if necessary (Ebert et al. Citation2012). The minimally invasive intervention leaves only the puncture wounds, which are similar to a blood collection; thus, the integrity of the body is maintained.
Limitations
The application has some limitations that are similar to a routine clinical procedure, such as a dry tap or accidental injury of a blood vessel, which may result in a false-positive subarachnoid hemorrhage. Furthermore, anatomical variants such as advanced degenerative spinal changes may hinder CSF puncture. Also, in badly damaged bodies (trauma, etc.) or in advanced stages of decomposition, sampling of CSF might be impossible. In addition, there are also system-related limitations, one of which is a limited range of motion of the robot arm. Furthermore, the robot is not yet able to fully aspirate the sample automatically. However, the current development aims to implement additional tools with the Virtobot for fully automated liquid sampling so that the risk of infection of technicians and forensic pathologists can be further reduced.
Conclusion
Our application allows for minimally invasive, fast, efficient and safe CSF sampling prior to autopsy, enabling microbiological testing and subsequent application of adequate precautionary measures for both autopsy technicians and forensic pathologists at autopsy.
Key points
PMCT data can be used along with a multifunctional robotic system for semiautomated postmortem needle placement.
The sampling of cerebrospinal fluid (CSF) is usually performed freehand and without prior imaging.
We are introducing a new application of semiautomated robotic CT-guided needle placement for postmortem CSF sampling.
This application may significantly improve postmortem diagnosis, especially in terms of safety issues (risk of infection), efficiency (time and costs) and accuracy (independence of the operators’ experience).
Ethical approval
A nonresponsibility declaration of the ethics committee has been received (BASEC-Nr. Req-2020-00227).
Data availability statement
The data availability is restricted due to legal restrictions applying to all cases at the Institute of Forensic Medicine. Data sharing is not allowed without permission from the public prosecutor.
Disclosure statement
No potential conflict of interest was reported by the authors.
References
- Ampanozi G, Halbheer D, Ebert LC, Thali MJ, Held U. 2019. Postmortem imaging findings and cause of death determination compared with autopsy: a systematic review of diagnostic test accuracy and meta-analysis. Int J Legal Med. doi:10.1007/s00414-019-02140-y.
- Brockbals L, Staeheli SN, Gascho D, Ebert LC, Kraemer T, Steuer AE. 2018. Time-dependent postmortem redistribution of opioids in blood and alternative matrices. J Anal Toxicol. 42(6):365–374. doi:10.1093/jat/bky017.
- Dirnhofer R, Jackowski C, Vock P, Potter K, Thali MJ. 2006. VIRTOPSY: minimally invasive, imaging-guided virtual autopsy. Radiographics. 26(5):1305–1333. doi:10.1148/rg.265065001.
- Ebert LC, Ptacek W, Breitbeck R, Furst M, Kronreif G, Martinez RM, Thali M, Flach PM. 2014. Virtobot 2.0: the future of automated surface documentation and CT-guided needle placement in forensic medicine. Forensic Sci Med Pathol. 10(2):179–186. doi:10.1007/s12024-013-9520-9. Epub 2014 Jan 29.
- Ebert LC, Ptacek W, Fürst M, Ross S, Thali MJ, Hatch G. 2012. Minimally invasive postmortem telebiopsy. J Forensic Sci. 57(2):528–530. doi:10.1111/j.1556-4029.2011.01990.x.
- Ebert LC, Ptacek W, Naether S, Fürst M, Ross S, Buck U, Weber S, Thali M. 2010. Virtobot – a multi-functional robotic system for 3D surface scanning and automatic post mortem biopsy. Int J Med Robot. 6(1):18–27. doi:10.1002/rcs.285.
- Flach PM, Gascho D, Schweitzer W, Ruder TD, Berger N, Ross SG, Thali MJ, Ampanozi G. 2014. Imaging in forensic radiology: an illustrated guide for postmortem computed tomography technique and protocols. Forensic Sci Med Pathol. 10(4):583–606. doi:10.1007/s12024-014-9555-6.
- Staeheli SN, Gascho D, Ebert LC, Kraemer T, Steuer AE. 2017. Time-dependent postmortem redistribution of morphine and its metabolites in blood and alternative matrices-application of CT-guided biopsy sampling. Int J Legal Med. 131(2):379–389. doi:10.1007/s00414-016-1485-2.
- Thali M. 2011. [Virtual autopsy (virtopsy) in forensic science: from the scalpel to the scanner]. Pathologe. 32(Suppl 2):292–295. doi:10.1007/s00292-011-1520-5.
- Thali MJ, Jackowski C, Oesterhelweg L, Ross SG, Dirnhofer R. 2007. VIRTOPSY – The Swiss virtual autopsy approach. Leg Med. 9(2):100–104. doi:10.1016/j.legalmed.2006.11.011.
- Thali MJ, Yen K, Schweitzer W, Vock P, Boesch C, Ozdoba C, Schroth G, Ith M, Sonnenschein M, Doernhoefer T, Scheurer E, Plattner T, Dirnhofer R. 2003. Virtopsy, a new imaging horizon in forensic pathology: virtual autopsy by postmortem multislice computed tomography (MSCT) and magnetic resonance imaging (MRI) – a feasibility study. J Forensic Sci. 48(2):386–403.