Abstract
‘Huabai 1’ is a novel albino tea germplasm that has a stable albino phenotype and prolonged albino period. In this study, widely targeted metabolomics was employed to analyze the metabolic profile of ‘Huabai 1’, and compare metabolites between albino shoots of ‘Huabai 1’ (HBW) and albino shoots of the broadest cultivated cultivar ‘Baiye 1’ (BYW). A total of 359 differential metabolites were identified in ‘Huabai 1’. Comparative analysis of differential metabolites revealed that 83 and 161 differential metabolites were specific for HBW and BYW, respectively. The phenylpropanoid and flavonoids biosynthesis are the key metabolic pathways, contributing to the color change in ‘Huabai 1’, while the purine metabolism and lipid-related metabolites may play important roles in regulating albinism in ‘Baiye 1’. Comparative analysis of amino acids and catechins revealed that the umami taste of albino tea may be due to the internal balance of amino acid components and the decrease in phenol-ammonia ratio. In addition, lipid-related metabolites, the main contributors to the aroma of tea, were significantly enriched in BYW, while, HBW possess higher antioxidative components than that of BYW, such as apigenin and genistein etc., suggesting that the ‘Huabai 1’ may have high prospects in commercialization and future breeding programs.
1. Introduction
Tea plant (Camellia sinensis (L.) O. Ktze.) is an important economic plant in the subtropical and tropical regions as their young leaves are used to produce the world’s second most consumed beverage after water. Recently, many albino tea cultivars with white or yellow leaf color have received increasing attention from tea researchers and manufacturers due to their unique phenotypes, great flavor and good economic value. These cultivars include ‘Huangjinya’ (Song et al. Citation2017a; Wu et al. Citation2019), ‘Baijiguan’ (Li et al. Citation2018), ‘Baiye 1’ (Li et al. Citation2016; Hao et al. Citation2019; Tu et al. Citation2020) and ‘Rougui’ (Wang et al. Citation2020). Based on the environment responsive factor, albino tea cultivars are divided into two main types, namely light-sensitive and temperature-sensitive. For example, ‘Huangjinya’ is a light-sensitive albino tea cultivar with yellow leaves under sunlight and green leaves under shading, while ‘Baiye 1’ is a temperature-sensitive albino tea cultivar with white leaves under low temperature and green leaves under high temperature conditions (Shin et al. Citation2018).
Chlorophyll deficiency is the direct expression of albinism in plants (Butnariu Citation2005). Current trend in tea research focuses largely on elucidating the albino mechanism of these cultivars. Most of these genetic studies have revealed a number of genes including transcription factors, carbohydrate and energy metabolism genes, chloroplast biogenesis genes, signal transduction genes, ubiquitination-related genes, stress-related genes and cell cycle genes related to albino phenotype (Ma et al. Citation2012; Ling et al. Citation2015; Zhu et al. Citation2016). Albinism results in the alteration of the amount and number of plant secondary metabolites. Studies have reported lower levels of carotenoids, catechins and caffeine contents, and higher levels of zeaxanthin contents and many free amino acids in albino leaves (Feng et al. Citation2014; Ling et al. Citation2015; Liu et al. Citation2017). Interestingly, different albino plants always have different amounts of metabolites, resulting from significant differences in quality related metabolites in albino tea leaves and their green counterparts. For example, total catechins content was found to be lower in ‘Baiye 1’ than in ‘Huangjinya’ (Feng et al. Citation2014).
The albino tea cultivar ‘Baiye 1’ is the widely studied and most cultivated albino germplasm due to its large economic value such as albino tender shoots and high amino acids. However, ‘Baiye 1’ is an unstable albino phenotype, with very short albino period of one month, and thus significantly decreases the economic benefits of albino tea cultivation. These limitations have opened doors for the investigation into the development of new albino tea cultivar with more stable albino phenotype and more stable quality related metabolites. ‘Huabai 1’, a more stable albino tea germplasm, was first reported by Qingping et al. (Citation2018). ‘Huabai 1’ has pure white shoots, sepal and pericarp, with more pronounced albinism period at the offspring stage, and economic value of 50% longer albinism period compared with ‘Baiye 1’; In addition, dried tea of ‘Huabai 1’contains high amino acids (84 mg/g), appropriate catechins (17.7%, w/w) and tea polyphenol (177 mg/g) which contribute to the umami taste of tea infusion and high anti-oxidative effect (Caunii and Butnariu Citation2013; Butnariu Citation2014), respectively (Ma et al. Citation2018). Our previous studies reported that the color change in ‘Huabai 1’ tender shoots was mainly regulated by genes related to the biosynthesis of phenylpropanoid as revealed by transcriptome analysis, however, the content changes of the quality metabolites are largely unknown (Ma et al. Citation2018). Widely targeted metabolomics is a classic method that can accurately detect hundreds of target metabolites and has been extensively used in some plant studies (Albinsky et al. Citation2010; Chen et al. Citation2013; Zhu et al. Citation2018; Meng et al. Citation2019; Zhou et al. Citation2019). Owing to the previous account, metabolic profiling using widely targeted metabolomics was performed to reveal the metabolic alteration in ‘Huabai 1’, and also identify the difference of metabolites involved in albinism between ‘Huabai 1’ and ‘Baiye 1’.
2. Materials and methods
2.1. Plant materials
Metabolic analysis was performed using two-year-old albino tea cultivars ‘Huabai 1’ and ‘Baiye 1’, as well as normal green tea cultivars ‘Longjing 43’ (LJ) and ‘Fudingdabai’ (FD), all of which were cultivated in the greenhouse of Nanjing Agricultural University. First, the albino shoots of ‘Huabai 1’ and ‘Baiye 1’ were compared with green shoots (green shoots of ‘Huabai 1’ (HBG) or green shoots of ‘Baiye 1’ (BYG), LJ and FD) in order to eliminate the error between albino shoots and normal green shoots. Then, Metabolomes between albino shoots of ‘Huabai 1’ (HBW) and albino shoots of ‘Baiye 1’ (BYW) were detected. 1 g tender shoots (a bud and a leaf) of each sample were harvested, frozen in liquid nitrogen and stored at -80°C prior to metabolomic analysis, each sample set 6 biological replicates.
2.2. Metabolomic analysis by liquid chromatography–electrospray ionization–tandem mass spectrometry (LC–ESI–MS/MS)
The freeze-dried samples were extracted according to the method described by Chen et al. (Citation2013). The extracts were analyzed using LC-ESI-MS/MS system (UPLC, Shim-pack UFLC SHIMADZU CBM20A; MS/MS, Applied Biosystems 4500 QTRAP). 5 μl of samples were injected onto a Waters ACQUITY UPLC HSS T3 C18 column (1.8 µm, 2.1 mm*100 mm) operating at 40°C and a flow rate of 0.4 mL/min. The mobile phases used were acidified water (0.04% acetic acid) (Phase A) and acidified acetonitrile (0.04% acetic acid) (Phase B). Compounds were separated using the following gradient: 95:5 Phase A/Phase B at 0 min; 5:95 Phase A/Phase B at 11.0 min; 5:95 Phase A/Phase B at 12.0 min; 95:5 Phase A/Phase B at 12.1 min and 95:5 Phase A/Phase B at 15.0 min. The effluent was connected to an ESI-triple quadrupole-linear ion trap (Q TRAP) MS (Butu et al. Citation2014).
LIT and triple quadrupole (QQQ) scans were acquired on a triple quadrupole-linear ion trap mass spectrometer (Q TRAP), AB Sciex QTRAP4500 System, equipped with an ESI-Turbo Ion-Spray interface, operating in a positive ion mode and controlled by Analyst 1.6.1 software (AB Sciex). The operation parameters were as follows: ESI source temperature 550°C; ion spray voltage (IS) 5500 V; curtain gas (CUR) 25psi and the collision-activated dissociation (CAD) was set at the highest level. QQQ scans were acquired as MRM experiments with optimized declustering potential (DP) and collision energy (CE) for each individual MRM transitions. The m/z range was set between 50 and 1000.
2.3. Qualitative and quantitative analysis
Metabolites were identified by comparing the fragmentation patterns, the retention time, and the accurate m/z value to metabolites in the database established by standards (Chen et al. Citation2013). Principle component analysis (PCA) and orthogonal partial least squares discrimination analysis (OPLS-DA) of identified metabolites were performed using the R package (https://www.r-project.org/). Differences in the metabolites were determined using Welch’s t-test (P < 0.01) in ZS97 and IRAT10. The significantly changed (P < 0.01) metabolites were used for subsequent PCA.
2.4. Quantitative analysis of chlorophylls
The total chlorophyll content was measured according to the method described by Song et al. (Citation2017b). Extraction was performed with 100 mg of fresh tea leaves using 15 ml of 95% ethanol. The extracts were filtered and analyzed with a UV-5800PC spectrophotometer (Metash, China). The absorbance values at 665 and 649 nm were record. Total chlorophyll (Chl mg/g) was calculated according to the formula 6.63A665 + 18.08A649 (Song et al. Citation2017a). Three biological replicates were conducted.
2.5. Statistical analysis
All statistical analyses were performed using Excel 2016 and GraphPad Prism 5. One-way ANOVA was used to evaluate the significance of differences.
3. Results
3.1. Phenotype and chlorophyll content of ‘Huabai 1’
New shoots of ‘Huabai 1’ showed white jade coloration, with the sepal and pericarp are pure white, and turned green as they developed. This phenotypic expression was, however, significantly different from temperature sensitive ‘Baiye 1’ plants which showed green leaf vein coloration even at albino stage (Figure (A)). New shoots of LJ and FD were full green at all developmental stages.
Figure 1. Leaf color phenotype and chlorophyll content of different tea cultivars. (A) Phenotypic expression and (B) total chlorophyll content of albino shoots of ‘Huabai 1’ (Huabai 1-W), albino shoots of ‘Baiye 1’ (Baiye 1-W), green shoots of ‘Huabai 1’ (Huabai 1-G), green shoots of ‘Baiye 1’ (Baiye 1-G), ‘Longjing 43’ (LJ) and ‘Fudingdabai’ (FD).
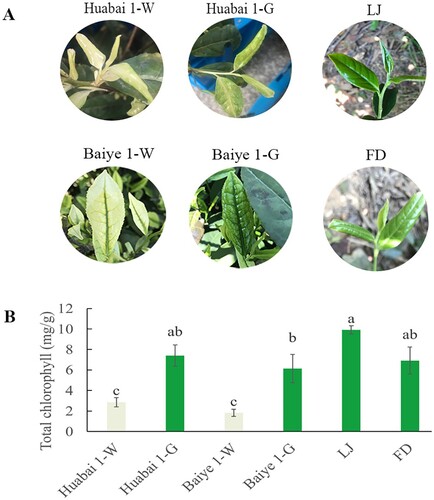
LJ, FD, green shoots of ‘Baiye 1’ and ‘Huabai 1’ revealed significantly higher chlorophyll content than white shoots of ‘Baiye 1’ and ‘Huabai 1’ (P < 0.05) (Figure (B)). However, no significant difference was observed between albino shoots of ‘Huabai 1’ and ‘Baiye 1’.
3.2. Differential metabolite identification between the white and green shoots of ‘Huabai 1’
Relative changes in metabolites between HBW and HBG were compared by widely targeted metabolomics. A total of 359 differential metabolites were identified, among which flavones were the most abundant, followed by ‘organic acid and its derivatives’ and ‘nucleotides and its derivatives’ (Figure ). This indicates that flavone related metabolites, the main contributors to the flavor quality of tea, are the largest group of metabolites that may regulate albinism in tea plants. The phenylpropanoid biosynthesis pathway enriched for the most differential metabolites, among which, the metabolites downstream of phenylalanine biosynthesis including sinapaldehyde and sinapyl alcohol were significantly increased in HBW (Table S1).
3.3. Classification and annotation of differential metabolites of white shoots from ‘Huabai 1’ and ‘Baiye 1’
HBW and BYW were compared with green shoots (HBG or BYG, LJ and FD) respectively. A total of 138 differential metabolites were identified in HBW, with flavone (22), flavonoid C-glycosides (14), hydroxycinnamoyl derivatives (12), amino acid derivatives (11) and nucleotides and its derivatives (11) as the most abundant classes of metabolites (Figure ). In additionally, a total of 216 differential metabolites were identified in BYW, with lipids (30), nucleotide and its derivatives (20), flavone (20), others (20) and amino acid derivatives (16) as the top five classes in this group (Figure ).
Figure 3. Venn diagram and classification of differential metabolites in (A) HBW compared with green shoots; (B) BYW compared with green shoots. HBW: albino shoots of ‘Huabai 1’; HBG: green shoots of ‘Huabai 1’; BYW: albino shoots of ‘Baiye 1’; BYG: green shoots of ‘Baiye 1’; FD: ‘Fudingdabai’ and LJ: ‘Longjing 43’.
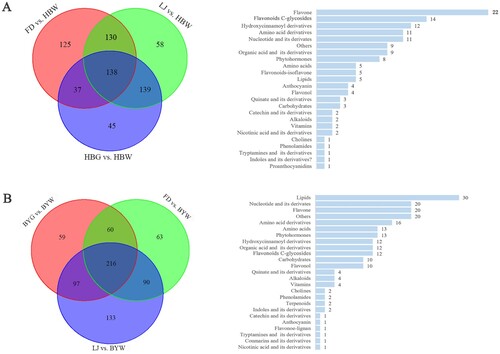
Further analysis on the albino germplasm, relative to green shoots (HBG or BYG, FD and LJ) revealed no obvious changes in the content of amino acids that regulate the umami and sweet taste in albino shoots of ‘Huabai 1’. Interestingly, the content of amino acids with bitter taste including L-Histidine and L-Methionine increased significantly, while DL-Methionine decreased significantly (Table S2). Moreover, the contents of amino acids with bitter, sweet and umami taste changed significantly in BYW (Table S3). Comparative analysis of catechins revealed that compared with green shoots (HBG or BYG, FD and LJ), only the catechin and its derivatives content changed in the HBW and BYW. Specifically, epigallate catechin O-hexside increased slightly while the protocatechuic acid O-hexoside decreased significantly in the HBW; protocatechuic acid O-hexoside decreased significantly in BYW (Tables S2 and S3).
3.4. Comparative analysis of the differential metabolites of the white shoots between ‘Huabai 1’ and ‘Baiye 1’
We finally identified the differential metabolites between HBW and BYW by comparing the 138 and 216 metabolites from HBW and BYW respectively. Then, the relative changes in metabolites between HBW and BYW were compared and annotated. The results showed that among these differential metabolites, only 55 metabolites were identified as common to HBW and BYW, while 83 and 161 differential metabolites were specific for HBW and BYW, respectively (Figure ). Notably, among the 83 metabolites annotated for HBW, flavone and flavonoid C-glycosides were the most enriched metabolites. However, lipids-glycerophospholipids, others and nucleotides and its derivatives were the most enriched metabolites among the 161 specific identified metabolites of BYW. The results also show clearly that at albino stage, the lipids related metabolites were more abundant in ‘Baiye 1’, while flavonoids were the most abundant in ‘Huabai 1’. These results suggest that there may be different metabolic mechanisms associated with albinism in ‘Huabai 1’ and ‘Baiye 1’.
Figure 4. Common and specific differential metabolites between the albino shoots of ‘Huabai 1’ and ‘Baiye 1’. The upper left and lower right part represent specific differential metabolites of albino ‘Huabai 1’ and ‘Baiye 1’, respectively; the middle part represents common differential metabolites of albino ‘Huabai 1’ and ‘Baiye 1’.
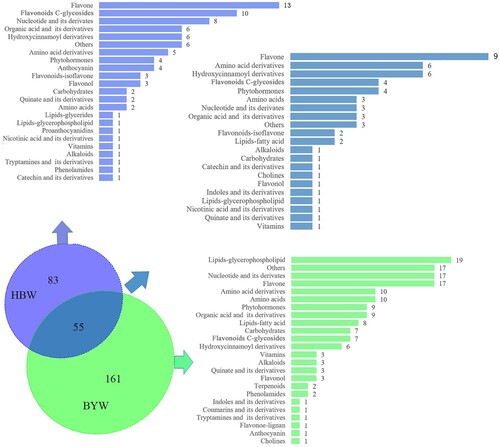
In addition, differential analysis of the 55 common differential metabolites between HBW and BYW (Figure ) revealed significantly lower levels of apigenin, astragalin, dihydromyricetin and genistein in BYW than in controls (BYG, LJ and FD); however, in HBW, these flavonoids showed significantly higher levels compared with controls (HBG, LJ and FD). This indicated that these specific flavonoids were significantly higher in HBW than in BYW. Moreover, phytohormones, including (+)-jasmonic acid (JA), gibberellin A4 (GA4) and N-[(-)-jasmonoyl]-(L)-isoleucine (Ja-L-Ile) were significantly higher in HBW, compared with BYW (Table S4). This indicates that phytohormones transduction may play an important role in albinism.
3.5. KEGG enrichment analysis of the differential metabolites between white and green shoots in ‘Huabai 1’ and ‘Baiye 1’
Comparative analysis of the green shoots and albino shoots of ‘Huabai 1’ and ‘Baiye 1’ respectively, revealed that the phenylpropanoid biosynthesis was the highest enrichment factor in ‘Huabai 1’, followed by flavone and flavonol biosynthesis as well as isoflavonoid biosynthesis; while purine metabolism was significantly enriched in differential metabolites in ‘Baiye 1’ (Figure ). This suggests that during the albescent stage, the phenylpropanoid is a major contributing class of metabolites in ‘Huabai 1’, while purine metabolism-associated metabolites are more pronounced during the color change of ‘Baiye 1’.
4. Discussion
Albino tea plants have gained increasing attention in recent physiological and molecular studies due to their unique phenotypes, essential metabolites, and special flavor. Research has shown that the albino phenotype causes metabolite changes in tea leaves, thereby influencing their flavor (Feng et al. Citation2014). A new and less studied germplasm, ‘Huabai 1’, which has been speculated to be superior to existing albino tea cultivars, was studied in this research. ‘Huabai 1’ shows pure white shoots, sepals and pericarp at the tender age, and turns green as the plant matures. Its phenotypic expression is significantly different from that of other light-sensitive and temperature-sensitive albino tea cultivars; however, the content changes of its essential metabolites are largely unknown. In this study, widely targeted metabolomics was employed to analyze the metabolic profile of ‘Huabai 1’, Additionally, the metabolites of HBW were compared to BYW. The results revealed a different metabolic mechanism associated with albinism between ‘Huabai 1’ and ‘Baiye 1’.
Metabolite composition not only contributes to tea flavor but also affects the quality and health benefits of tea (Ji et al. Citation2018; Leal et al. Citation2018). In our previous studies, transcriptome analysis of ‘Huabai 1’ showed that the phenylpropanoid biosynthesis pathway was enriched for the most differentially expressed genes (DEGs) in albinism, which concluded that albinism in ‘Huabai 1’ was regulated by genes in the phenylpropanoid biosynthesis pathway (Ma et al. Citation2018). Interestingly, the result in the present study corroborates that of our previous study. In this study, KEGG enrichment analysis of differential metabolites between HBW and HBG revealed that the most differential metabolites were involved in phenylpropanoid biosynthesis. As shown in Table S1, the contents of metabolites downstream of phenylprponoid biosynthesis including sinapaldehyde and sinapyl alcohol increased in HBW, while, transcriptome analysis found that the expression of cinnamyl-alcohol dehydrogenase (CAD) expressing on this metabolic pathway was also up-regulated in HBW (Qingping et al. Citation2018). The up-regulation of CAD and the increase of Sinapaldehyde, the catalytic substrate of CAD, are the direct causes of Sinapyl alcohol accumulation. Lignin biosynthesis is a major branch of phenylpropanoid biosynthesis and produces lignin polymers (Boerjan and Ralph Citation2003). CAD is one of the key enzymes in lignin synthesis, catalyzing the conversion of the–aldehydes to–alcohols (Chen et al. Citation2021). Studies have shown that CAD belongs to the family of defense-related CADs and can combine with signaling molecules such as ABA, salicylic acid and auxin to produce stress response mechanisms (Goicoechea et al. Citation2010; Kim and Bae Citation2010). In this study, 18 phytohormones were identified as differential metabolites, as shown in Figure , moreover, the expression level of CAD in the albino shoots of ‘Huabai 1’ was significantly up-regulated. This is mainly because the biotic or abiotic stress from the environment triggers the transduction of phytohormones in tea plants, thereby initiating the stress response mechanism caused by the combination of CAD and phytohormones. CAD can change the lignin structure, additionally, the loss of CAD function generally leads to reduction of lignin content and red coloration of xylem tissue (Sibout et al. Citation2005; Ralph et al. Citation2010; Zhao et al. Citation2013). The expression of CAD in HBW was significantly higher than in green shoots, so it can be speculated that the expression of CAD in green leaves is inhibited, which triggers the changes in the structure of lignin and leads to variations in leaf color. This, therefore, suggests that phenylpropanoid biosynthesis is an important metabolic pathway that regulates albinism in ‘Huabai 1’.
Flavonoids are the main flavor substances and functional components in tea (Danlele et al. Citation2014; Kuhn et al. Citation2016), while the shikimic acid pathway is one of the important pathways of flavonoid biosynthesis. Zhu et al. found that the expression of four shikimic acid pathway related genes was related to the albino phenotype of ‘Baiye 1’ (Zhu et al. Citation2016). As shown in Figures and , flavonoids are abundant in HBW, it can be seen that the flavonoid biosynthesis pathway, which is associated with phenylpropanoid biosynthesis, could also contribute to the color change in ‘Huabai 1’ tender shoots. In addition, differential analysis of the common differential metabolites revealed that special flavonoids including apigenin (Liang et al. Citation2017), astragalin (Ma et al. Citation2015), dihydromyricetin (Hou et al. Citation2015) and genistein (Russo et al. Citation2016) were significantly higher in HBW than in BYW, these flavonoid components have anticancer, anti-inflammatory and neuroprotective effects (Butu and Rodino Citation2014). This indicates that HBW may have a higher antioxidative function than BYW.
Unlike ‘Huabai 1’, the purine metabolism was significantly enriched for differential metabolites in ‘Baiye 1’, and lipid-related metabolites were the most enriched in BYW. This contradicts the result in the previous study, in which the albinism in ‘Baiye 1’ was also associated with metabolites related to phenylpropanoid biosynthetic pathway (Ling et al. Citation2015; Zeng et al. Citation2019). This may be due to the use of a variety of green leaves as controls to eliminate the error between cultivars to a large extent, resulting in differences in research results. Moreover, the phenylpropanoid biosynthetic pathway may affect HBW more than BYW. Lipids is the main contributor to the aroma of tea, so it is speculated that tea made from BYW has a more significant aroma than HBW. To sum up, this reveals the purine metabolism and lipid-related metabolites may play important roles during the color change of ‘Baiye 1’.
Amino acids are the major compounds in tea plant, and are known to be the key contributor to tea flavor (Liu et al. Citation2016). Both ‘Huabai 1’ and ‘Baiye 1’ are rich in amino acids (Ling et al. Citation2015; Ma et al. Citation2018). Arginine, histidine, tyrosine, phenylalanine, methionine, and branched chain amino acids, including valine, isoleucine and leucine, have a bitter flavor, whereas serine, alanine, threonine and glycine have a sweet flavor (Yuasa et al. Citation2017). Aspartic and glutamic acids contribute to the umami taste (Ninomiya Citation2015; Phat and Moon Citation2016). In the present study, the contents of amino acids that give tea its umami and sweet taste did not show change significantly in albino shoots of ‘Huabai 1’, while the contents of amino acids that contribute to the bitter taste of tea changed significantly. L-Histidine and L-Methionine which contribute to the bitter taste of tea were significantly higher in HBW than that of controls (HBG, LJ and FD) (Table S2). However, the reason for the high umami taste of dried tea made from white shoots of ‘Huabai 1’ needs further exploration. The content of amino acids with bitter, sweet and umami tastes in BYW was significantly changed compared with that of controls (BYG, FD and LJ) (Table S3). Among them, L-glutamic acid, the main contributor to the umami taste of tea, increased its content significantly, which is consistent with previous research results (Zeng et al. Citation2019). This could possibly explain the reason for the high umami taste of ‘Baiye 1’. Putting together, the umami taste of albino tea cultivars may be due to the internal balance of amino acid components. Moreover, as shown in Figure , the comparative analysis of specific differential metabolites between HBW and BYW showed that ten amino acidassociated metabolites were enriched in tender shoots of ‘Baiye 1’ during the albescent stage, which indicated prominent changes in amino acid metabolism. However, only two amino acids in ‘Huabai 1’ showed significant changes, which suggests that different albino tea varieties have different metabolic mechanisms associated with albinism.
Catechins are the major class of flavonoids in tea and play a significant role in tea flavor quality. In this study, comparative analysis of catechins revealed that compared with that of green shoots, only the content of catechin and its derivatives changed in HBW or BYW, while there was no significant change in the components of catechin (Tables S2 and S3). It can be deduced from this result that albinism in tea shoots does not reduce the functional components of catechins. According to Wong, catechin confers bitterness and astringency to green tea infusion (Wong Citation2018). Therefore, the bitter taste of albino tea is lighter, which may be caused by the decrease in phenol-ammonia ratio due to the accumulation of amino acids. Further studies, however, need to be conducted to ascertain this assertion.
5. Conclusions
In conclusion, the widely targeted metabolomic analyses revealed a different metabolite accumulation pattern between the new tea germplasm ‘Huabai 1’ and the broadest cultivated cultivar ‘Baiye 1’. The phenylpropanoid and flavonoids biosynthesis are the key metabolic pathways, contributing to the color change in ‘Huabai 1’, while the purine metabolism and lipid-related metabolites may play important roles in regulating albinism in ‘Baiye 1’, which suggests that there are different metabolic mechanisms associated with albinism in ‘Huabai 1’ and ‘Baiye 1’. The umami taste of albino tea may be due to the internal balance of amino acid components and the decrease in phenol-ammonia ratio, rather than Simply accumulation of amino acids that give tea its umami or sweet taste and the reduce of catechins. In addition, the tea made from BYW may have a more significant aroma than HBW, while, compared with BYW, albino shoots of ‘Huabai 1’ possess higher antioxidative components, such as apigenin and genistein etc., suggesting that the ‘Huabai 1’ may have high prospects in commercialization and future breeding programs.
Supplemental Material
Download Zip (157.2 KB)Acknowledgements
We thank Dr. Yuehua Ma (Central Laboratory of College of Horticulture, Nanjing Agricultural University) for assistance in using the UPLC system (Acquity, Waters) and the National Program for Student Innovation through research and training.
Disclosure statement
No potential conflict of interest was reported by the author(s).
Data availability statement
The data that support the findings of this study are available in the OMIX in the BIG Data Center at https://bigd.big.ac.cn/omix/, reference number OMIX246.
Additional information
Funding
References
- Albinsky D, Sawada Y, Kuwahara A, Nagano M, Hirai A, Saito K. 2010. Widely targeted metabolomics and coexpression analysis as tools to identify genes involved in the side-chain elongation steps of aliphatic glucosinolate biosynthesis. Amino Acids. 39(4):1067–1075.
- Boerjan W, Ralph J. 2003. Lignin biosynthesis. Annu Rev Plant Biol. 54(1):519–546.
- Butnariu M. 2005. Achieving the crystalline state of chlorophyll of the Fir–tree (Abies alba) and the pine (Pinus sylvestris). Rev Chim Bucharest. 56(4):441–443.
- Butnariu M. 2014. Detection of the polyphenolic components in Ribes nigrum L. Ann Agric Environ Med. 21(1):11–14.
- Butu M, Butnariu M, Rodino S. 2014. Study of zingiberene from Lycopersicon esculentum fruit by mass spectometry. Digest J Nanomater Biostruct. 9(3):935–941.
- Butu M, Rodino S. 2014. Screening of bioflavonoid and antioxidant activity of Lens culinaris medikus. Digest J Nanomater Biostruct (DJNB. 9(2):519–529.
- Caunii A, Butnariu M. 2013. Design management of functional foods for quality of life improvement. Ann Agric Environ Med. 20(4):736–741.
- Chen C, Chang J, Wang S, Lu J. 2021. Cloning, expression analysis and molecular marker development of cinnamyl alcohol dehydrogenase gene in common wheat. Protoplasma. 17.
- Chen W, Gong L, Guo Z, Wang W, Zhang H, Liu X, Yu S, Xiong L, Luo J. 2013. A novel integrated method for large-scale detection, identification, and quantification of widely targeted metabolites: application in the study of rice metabolomics. Mol Plant. 6(6):1769–1780.
- Danlele D, Rio D, Vauzour P, Mena J. 2014. Bibavsttlability, bioactivity and impact-on health of dietary,iavonoids and related compounds: an update. Arch Toxicol. 88(10):1803–1853.
- Feng L, Gao M-J, Hou R-Y, Hu X-Y, Zhang L, Wan X-C. 2014. Determination of quality constituents in the young leaves of albino tea cultivars. Food Chem. 155:98–104.
- Goicoechea M, Lacombe E, Legay S, Mihaljevic S. 2010. EgMYB2, a new transcriptional activator from Eucalyptus xylem, regulates secondary cell wall formation and lignin biosynthesis. Plant J. 43(4):553–567.
- Hao WJ, Wang SL, Yao MZ, Ma JQ, Xu YX. 2019. The complete chloroplast genome of an albino tea, Camellia sinensis cultivar ‘Baiye 1’. Mitochond DNA Part B Resour. 4(2):2.
- Hou XL, Tong Q, Wang WQ, Shi CY. 2015. Suppression of inflammatory responses by dihydromyricetin, a flavonoid from Ampelopsis grossedentata, via inhibiting the activation of NF-κB and MAPK signaling pathways. J Nat Prod. 78:7.
- Ji H-G, Lee Y-R, Lee M-S, Hwang KH, Park CY, Kim E-H, Park JS, Hong Y-S. 2018. Diverse metabolite variations in Tea (Camellia sinensis L.) leaves grown under various shade conditions revisited: a metabolomics study. J Agric Food Chem. 66(8):1889–1897.
- Kim YH, Bae JM. 2010. Transcriptional regulation of the cinnamyl alcohol dehydrogenase gene from sweetpotato in response to plant developmental stage and environmental stress. Plant Cell Rep. 29(7):779–791.
- Kuhn BM, Errafi S, Bucher R, Dobrev P, Geisler M, Bigler L. 2016. 7-Rhamnosylated flavonols modulate homeostasis of the plant hormone auxin and affect plant development. J Biol Chem. 291(10):5385-5395.
- Leal J, Suarez L, Jayabalan R, Huerta Oros J. 2018. A review on health benefits of kombucha nutritional compounds and metabolites. Cyta J Food. 16(1):390–399.
- Li C-F, Ma J-Q, Huang D-J, Ma C-L, Jin J-Q, Yao M-Z. 2018. Comprehensive dissection of metabolic changes in albino and green tea cultivars. J Agric Food Chem. 66(8):2040–2048.
- Li C-F, Xu Y-X, Ma J-Q, Jin J-Q, Huang D-J, Yao M-Z, Ma C-L. 2016. Biochemical and transcriptomic analyses reveal different metabolite biosynthesis profiles among three color and developmental stages in ‘Anji Baicha’ (Camellia sinensis). BMC Plant Biol. 16(1):195.
- Liang H, Sonego S, Gyengesi E, Rangel A, Niedermayer G, Karl T, Münch G. 2017. Anti-inflammatory and neuroprotective effect of apigenin: studies in the GFAP-IL6 mouse model of chronic neuroinflammation. Free Radical Biol Med. 108:S10.
- Ling Y, Li-gui X, Ting-ting D, Yang W, Juan L. 2015. Comparative profiling of gene expression in Camellia sinensis L. cultivar AnJiBaiCha leaves during periodic albinism. Gene. 561(1):23–29.
- Liu GF, Han ZX, Feng L, Gao LP, Gao MJ, Gruber MY, Zhang ZL, Xia T, Wan XC. 2017. Metabolic flux redirection and transcriptomic reprogramming in the albino tea cultivar ‘Yu-Jin-xiang’ with an emphasis on catechin production. Sci Rep. 7:15.
- Liu J, Zhang Q, Liu M, Ma L, Shi Y. 2016. Metabolomic Analyses reveal distinct change of metabolites and quality of green tea during the short duration of a single Spring season. J Agric Food Chem. 64(16):3302–3309.
- Ma CL, Chen L, Wang X-C, Jin J-Q, Ma J-Q, Yao M-Z. 2012. Differential expression analysis of different albescent stages of ‘Anji Baicha’ (Camellia sinensis (L.) O. Kuntze) using cDNA microarray. 2012, 148(none).
- Ma QP, Li H, Zou ZW, Arkorful E, Lv QR, Zhou QQ, Chen X, Sun K. 2018. Transcriptomic analyses identify albino-associated genes of a novel albino tea germplasm ‘Huabai 1’. Hortic Res. 5:8.
- Ma Z, Piao T, Wang Y. 2015. Astragalin inhibits IL-1β-induced inflammatory mediators production in human osteoarthritis chondrocyte by inhibiting NF-κB and MAPK activation. Int Immunopharmacol. 25(1):83–87.
- Meng J, Wang B, He G, Wang Y, Tang X, Wang S, Ma Y, Fu C, Chai G. 2019. Metabolomics integrated with transcriptomics reveals redirection of the phenylpropanoids metabolic flux in ginkgo biloba. J Agric Food Chem. 67(11):3284–3291.
- Ninomiya K. 2015. Science of umami taste: adaptation to gastronomic culture. Flavour. 4(1):1–5.
- Phat C, Moon B. 2016. Evaluation of umami taste in mushroom extracts by chemical analysis, sensory evaluation, and an electronic tongue system. Food Chem. 192:1068–1077.
- Qingping M, Huan L, Zhongwei Z, Emmanuel A. 2018. Transcriptomic analyses identify albino-associated genes of a novel albino tea germplasm ‘Huabai 1’. Hortic Res. 5(1):2–8.
- Ralph J, Kim H, Lu F. 2010. Identification of the structure and origin of a thioacidolysis marker compound for ferulic acid incorporation into angiosperm lignins (and an indicator for cinnamoyl CoA reductase deficiency). Plant J. 53(2):368–379.
- Russo M, Russo GL, Daglia M, Kasi PD, Ravi S, Nabavi SF, Nabavi SM. 2016. Understanding genistein in cancer: The “good” and the “bad” effects: A review. Food Chem. 196:589–600.
- Shin Y-H, Yang R, Shi Y-L, Li X-M, Fu Q-Y, Lu J-L, Ye J-H, Wang K-R, Ma S-C. 2018. Light-sensitive albino tea plants and their characterization. Hortscience. 53(2):144–147.
- Sibout R, Eudes A, Mouille G, Pollet B. 2005. Cinnamyl alcohol dehydrogenase-C and -D are the primary genes involved in lignin biosynthesis in the floral stem. Plant Cell. 17(7):2059–2076.
- Song L, Ma Q, Zou Z, Sun K, Yao Y, Tao J, Kaleri NA. 2017a. Molecular link between leaf coloration and gene expression of flavonoid and carotenoid biosynthesis in Camellia sinensis cultivar ‘Huangjinya’. Front Plant Sci. 8:803.
- Song L, Qingping M, Zhongwei Z, Kang S, Yuantao Y, Jihan T AKN. 2017b. Molecular link between leaf coloration and gene expression of flavonoid and carotenoid biosynthesis in Camellia sinensis cultivar ‘Huangjinya’. Front Plant Sci. 8:00803.
- Tu YY, Chen LF, Ren N, Li B, Wu YY, Rankin GO, Rojanasakul Y. 2020. Standardized saponin extract from Baiye No.1 tea (Camellia sinensis) flowers induced S phase cell cycle arrest and apoptosis via AKT-MDM2-p53 signaling pathway in ovarian cancer Cells. Molecules. 25(15):17.
- Wang PJ, Zheng YC, Guo YC, Liu BS, Jin S, Liu SZ, Zhao F, Chen XJ, Sun Y. 2020. Widely targeted metabolomic and transcriptomic analyses of a novel albino tea mutant of “Rougui”. Forests. 11(2):15.
- Wong DW. 2018. In mechanism and theory in food chemistry. Cham: Springer International Publishing.
- Wu HY, Ke JP, Wang W, Kong YS, Zhang P, Ling TJ. 2019. Discovery of neolignan glycosides with acetylcolinesterase inhibitory activity from Huangjinya green tea guided by ultra performance liquid chromatography-tandem mass spectrometry data and global natural product social molecular networking. J Agric Food Chem. 67(43):11986–11993.
- Yuasa M, Koe M, Maeda A, Eguchi A, Abe H. 2017. Characterization of flavor component in Japanese instant soup stocks ‘dashi’. Int J Gastron Food Sci. 9:55–61.
- Zeng C, Lin H, Liu Z. 2019. Analysis of young shoots of “Anji Baicha’ (Camellia sinensis) at three developmental stages using nontargeted LC-MS-based metabolomics. J Food Sci. 84(7):1746–1757.
- Zhao Q, Tobimatsu Y, Zhou R, Pattathil S, Gallego-Giraldo L, Fu C, Jackson LA, Hahn MG, Kim H. 2013. Loss of function of cinnamyl alcohol dehydrogenase 1 leads to unconventional lignin and a temperature-sensitive growth defect in Medicago truncatula. Pnas. 110(33):13660–13665.
- Zhou K, Hu L, Li Y, Chen X, Zhang Z, Liu B, Li P, Gong X. 2019. MdUGT88F1-mediated phloridzin biosynthesis regulates apple development and valsa canker resistance. Plant Physiol. 180(4):2290–2305.
- Zhu G, Wang S, Huang Z, Zhang S, Liao Q, Zhang C, Lin T, Qin M, Peng M. 2018. Rewiring of the fruit metabolome in tomato breeding. Cell. 172(1-2):249.
- Zhu XJ, Zhao Z, Xin HH, Wang ML, Wang WD, Chen X. 2016. Li XH: isolation and dynamic expression of four genes involving in shikimic acid pathway in Camellia sinensis ‘Baicha 1’ during periodic albinism. Mol Biol Rep. 43(10):1119–1127.