Abstract
Apolipoprotein E (ApoE), a component of plasma lipoproteins, also can promote tumor cell proliferation, migration and metastasis. However, the role of host ApoE in regulating anti-tumor immunity is still unknown. Here host ApoE-deficient (ApoE −/−) mice were used to understand the role of ApoE in antitumor immunity. Tumor-infiltrating lymphocytes subsets and exhausted T cells were measured by flow cytometry in B16F10 and MC38 immunogenic tumor-bearing mice. Both B16F10 and MC38 growth were significantly repressed in ApoE −/− mice. Tumor-infiltrating T cells and pro-inflammatory M1 macrophages were significantly increased in ApoE −/− mice. Moreover, decreased PD-1+ cells representing exhausted CD8+ T cells and increased cytotoxic CD8+ T cells function and proliferation indicating as TNF- α+, IFN-γ+, GZMB+ and Ki67+ CD8+ cells in tumor-infiltrating lymphocytes of ApoE −/− mice may contribute to the antitumor function of host ApoE deficiency. Thus, inhibition of ApoE could be a good strategy for cancer immunotherapy.
Introduction
Apolipoprotein E (ApoE) is a component of plasma lipoproteins that could interact with low-density lipoprotein receptor (LDL-R) and mediate the uptake of lipids, fat-soluble vitamins, and cholesterol. ApoE is therefore important for the processing of triglyceride-rich lipoproteins and ApoE-deficient mice could develop extreme hypercholesterolemia (McNeill et al. Citation2010). In tumor cells, increased ApoE expression was found in gastric (Oue et al. Citation2004), lung (Su et al. Citation2011), endometrial cancer (Huvila et al. Citation2009) and it promotes cell proliferation, migration and metastasis. However, there is still unknown of the role of host ApoE in regulating tumor growth.
Antitumor immunity means using the host immune system to fight cancer, which yields long-term clinical benefit for tumor patients. Tumor-associated macrophages and cytotoxic T lymphocytes are very important for antitumor immunity (Munhoz and Postow Citation2016). However, under the tumor stimulation, T cell exhaustion, a state of T cell dysfunction, arises. Cell surface inhibitory receptors such as PD-1, Tim-3 are typical markers of T cell exhaustion, repress the anti-tumor immunity and promote tumor growth (Wherry Citation2011).
ApoE is expressed in multiply immune cells including T cells, macrophages, natural killer T cells (Zhang et al. Citation2010). In vitro study indicated that ApoE could suppress T cell proliferation (Avila et al. Citation1982). ApoE-deficient mice can increase lipopolysaccharide-induced pro-inflammatory response (Van Oosten et al. Citation2001). ApoE was found to modulate nitric oxide expression in human macrophages (Vitek et al. Citation1997). Increased secreted ApoE proteins could suppress the innate antitumor response (Tavazoie et al. Citation2018). Therefore, ApoE plays a key role in modulating inflammation and regulating immune response.
Here the roles of ApoE in anti-tumor immunity and T cell exhaustion were investigated by using host ApoE-deficient (ApoE −/−) mice. Multiply tumor cell lines were injected to ApoE −/− mice, tumor-infiltrating T cells and macrophages were analyzed by flow cytometry (FACS).
Materials and methods
Mice
Congenic ApoE −/− mice in C57BL/6J genetic background were generated by deletion of the exon 3 (Piedrahita et al. Citation1992). Both wild type and ApoE −/− mice were purchased from Gempharmatech (Jiangsu, China). Experiments were conducted in accordance with the guidelines of the Animal Care and Use Committee of Luohe Medical College. Mice were feed with normal diet (Gempharmatech, Jiangsu, China).
Cell culture and tumor injection
B16F10 and MC38 tumor cells were maintained in RPMI 1640 or DMEM high Glucose (Solarbio, Beijing, China) supplemented with 10% FBS (Beyotime, Shanghai, China), and 1% Pen/Strep (Solarbio, Beijing, China), respectively.
wide type (WT) (n = 6) and ApoE −/− (n = 6) male mice (20 ± 2 g) were used for tumor injection at 8 weeks old. Tumor cells (5 × 105) were injected subcutaneously to the flank of WT or ApoE −/− mice. Tumor sizes were measured every 2–3 days by a caliper from day 7 after tumor injection and tumor volumes were calculated by length × width2/2. Mice were scarificed on day 15 after tumor injection.
Splenocytes purification
Spleens were mechanically disrupted onto a 70 µm cell strainer using a plunger and resuspended in 5 mL of ACK buffer (155 mM NH4Cl, 10 mM KHCO3, 0.1 mM EDTA) for 5 min on ice. Cell suspensions were washed by RPMI 1640.
Tumor-infiltrating cells purification
Tumors were collected, mechanical disrupted and digested in RPMI 1640 with Collagenase D and DNase I (Solarbio, Beijing, China) for 30 min at 37°C under agitation. Then the cells were disrupted onto a 100 µm strainer, lymphocytes from tumors were enriched using Percoll (Solarbio, Beijing, China) density gradient separation (70%/40%), and washed twice with RPMI 1640.
Cell surface makers stanning and flow cytometry analysis
Splenocytes and tumor-infiltrating cells were stanning with FcR blocking reagent (Miltenyibiotec, Bergisch Gladbach, Germany) for 10 min on ice in PBS with 1% BSA. The dead cells were stanning by Fixable viability dye (Thermofisher, CA, USA). Antibodies against BV605-CD4 (100548, Biolegend), BV650-CD8 (100742, Biolegend), APC-CD3 (100236, Biolegend), FITC-CD45 (103107, Biolegend), PerCP/Cyanine5.5-CD11b (101227, Biolegend), PE-CD11c (557401, Bioscience), PerCP/Cyanine5.5-PD-1 (109119, Biolegend), BV605-Tim-3 (119721, Biolegend) were used to staining on ice for 30 min. After washing, the cells were acquired on BD LSR Fortessa (BD, NJ, USA) and analyzed with Flow Jo v.10 software (Tree Star Inc, NJ, USA).
Intracellular cytokine staining
Tumor-infiltrating cells were re-stimulated in vitro for 4 h at 37°C 5% CO2 in RPMI 1640 with cell activation cocktail (with Brefeldin A) (423304, Biolegend). After cell surface makers staining as above, the cells were fixed and permeabilizated by BD Fixation/permeabilization kit (BD, NJ, USA). APC-IFN-γ (17-7311-82, Thermofisher), APC-TNF-α (506308, Biolegend), APC-Ki67 (652406, Biolegend) and APC-GZMB (372204, Biolegend) were used for stanning overnight at 4°C. After washing, the cells were acquired on BD LSR Fortessa and analyzed with FlowJo v.10 software.
Statistics
Flow cytometry data shown as mean ± SD were examined using unpaired two-tailed Student’s t test. Tumor growth curve shown as mean ± SEM was examined by ANOVA.
Results
Host ApoE deficiency reduces the tumor growth
To understand the role of host ApoE in tumor growth, ApoE −/− mice were subcutaneously injected with B16F10 cells, immunogenic melanoma cells. The tumor sizes were measured in the following 15 days. The tumor growths in ApoE −/− mice were significantly slower than in WT mice (Figure (A)). The tumor weights in ApoE −/− mice were significantly reduced by 49.1% compared to WT mice (Figure (B)). Moreover, MC38 cells, an immunogenic colorectal cancer cells, were injected to ApoE −/− mice. The tumor sizes in ApoE −/− mice were much smaller than the tumor in WT mice (Figure (C)). Consistently, MC38 tumor growths were much slower in ApoE −/− mice (Figure (D)). Thus, these suggested that host ApoE deficiency could reduce the tumor growth.
Figure 1. Tumor growths were slower in ApoE −/− mice. (A) Tumor growth curves of B16F10 were measured in WT and ApoE −/− mice. n = 6; ***p < .001. (B) The tumor weights of B16F10 from WT and ApoE −/− mice were measured when the mice were sacrificed. n = 3; **p < .01. (C) Typical tumor photos of MC38 from WT and ApoE −/− mice were shown. (D) Tumor growth curves of MC38 cells were measured in WT (n = 4) and ApoE −/− (n = 5) mice. ***p < .001.
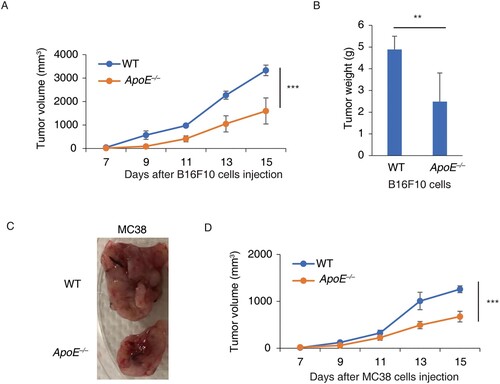
Host ApoE deficiency increase the T cell and macrophage infiltration
To understand the role of ApoE in antitumor immune response, tumor-infiltrating cells and splenocytes were purified. In spleen, the CD3+ cell percentages, indicating as T cells, were similar between WT and ApoE −/− mice. In tumor-infiltrating cells, the T cells amounts were significantly increased in ApoE −/− mice, compared to WT mice (Figure (A,B)). This result suggested that host ApoE deficiency specifically increased T cell numbers in tumor microenvironment but not in spleen. Moreover, the total macrophages indicating as CD11b+ CD3−CD45+ cells in ApoE −/− mice were significantly increased in tumor microenvironment but not in spleen. In tumor-associated macrophage, the pro-inframammary M1 macrophages (CD11b+ CD11c+ cells) were significantly increased in ApoE −/− mice. (Figure (C,D)) These results suggested that host ApoE deficiency could increase antitumor immune response via recruiting more amounts of T cells and macrophages.
Figure 2. Tumor-infiltrating T cells and macrophages were increased in ApoE −/− mice. CD3+ cell percentages were measured in splenocytes and tumor-infiltrating lymphocytes (TIL) from WT and ApoE −/− mice. (A) Typical FACS photos were shown. (B) The statistic results were shown. n = 3; *p < .05; NS, not significant. Macrophage percentages were measured in splenocytes and TIL from WT and ApoE −/− mice. (C) Typical bivariant plots were shown (D) CD11b+ cell percentages in CD3− CD45 + cell were shown. (E) CD11C+ cell percentages in CD11b+ CD3− CD45 + cells were shown. n = 3; *p < .05; NS, not significant.
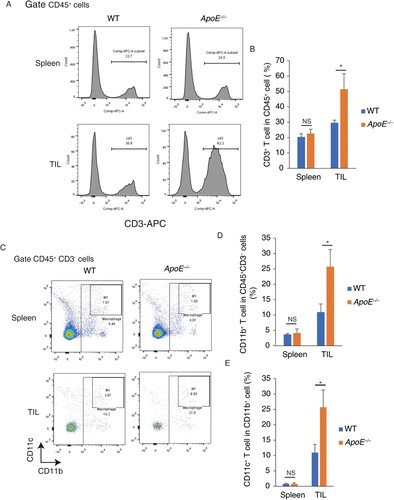
Host ApoE deficiency increases the cytotoxic CD8+ T cell infiltration
Cytotoxic CD8+ T cells expressed IFN-γ or tumor necrosis factor (TNF)-α are direct tumor killers in tumor microenvironment (Maimela et al. Citation2019), the level of cytotoxic CD8+ T cells were therefore measured in both WT and ApoE −/− mice. In ApoE −/− mice, tumor- infiltrating CD4+ (Figure (A,B)) and CD8+ T cells (Figure (A, C)) amounts were significantly increased by 55.9% and 98.65%, respectively. There is no significant difference between WT and ApoE −/− CD8+ T cells in spleen, and the CD4+ T cells were slightly increased in ApoE −/− splenocytes.
Figure 3. Tumor-infiltrating cytotoxic CD8+ T cell were increased in ApoE −/− mice. CD4+ and CD8+ T cell percentages were measured in splenocytes and TIL from WT and ApoE −/− mice. (A) Typical FACS bivariant plots were shown. (B) CD4+ T cell percentages in CD45 + cells were shown. (C) CD8+ T cell percentages in CD45 + cells were shown. n = 3; *p < .05; NS, not significant. TNF-α+ (D), IFN-γ+ (E), GZMB+ (F) and Ki67+ (G) CD8+ T cells percentage were measured in tumor-infiltrating cytotoxic CD8+ T cells from WT and ApoE −/− mice. n = 3; **p < .01.
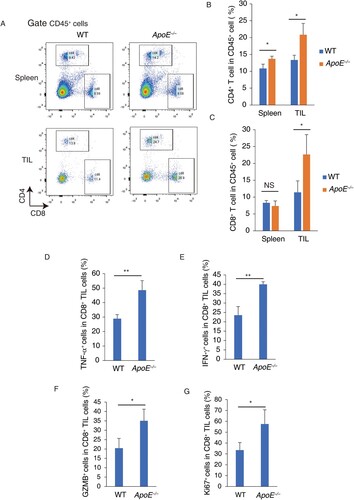
Moreover, the cytotoxic functions of tumor- infiltrating CD8+ T cells were analyzed in ApoE −/− mice. The pro-inflammatory cytokine TNF-α+ (Figure (D)) and IFN-γ+ (Figure (E)) expressed intertumoral CD8+ T cells were increased in ApoE −/− mice. Granzyme B (GZMB), a cytotoxic protein, usually expressed by cytotoxic CD8+ T cells and induce target cell death. In ApoE −/− mice, GZMB+ intertumoral CD8+ T cells were increased (Figure (F)). Ki67 was used to understand the cell proliferation and growth of CD8+ T cells. Ki67+ intertumoral CD8+ T cells were significantly increased in ApoE −/− mice (Figure (G)). These results indicate that host ApoE deficiency increases the cytotoxic CD8+ T cell infiltration, and increases the cytotoxic functions of intertumoral CD8+ T cells. This may be associated the anti-tumor functions of host ApoE deficiency.
Host ApoE deficiency suppresses tumor-induced T cell exhaustion
T cell exhaustion, one of blockages of antitumor immunity, could repress the cytotoxic CD8+ T cells function. PD-1 and Tim-3, inhibitory receptors, were therefore measured in tumor-infiltrating CD8+ T cells (Figure (A)). In ApoE −/− mice, the PD-1+ cell population was significantly decreased. PD-1+ Tim-3+ cells indicating as the terminal exhausted T cells were also significantly reduced in ApoE −/− mice. (Figure (B)) These results suggested that host ApoE deficiency suppresses tumor-induced T cell exhaustion.
Discussion
T cell exhaustion, a major challenge of cancer immunotherapy, is characterized by increased inhibitory receptors (such as PD-1 and Tim-3) and reduced the cytotoxic cytokines secretion in tumor-infiltrating cells. Overcoming the problem of exhausted T cells via blocking PD-1 has been significantly increased the tumor therapy (Patil et al. Citation2015). Liver-X nuclear receptor (LXR) could regulate the expression of ApoE. In LXR-deficient mice, reduced ApoE could increase the anti-PD-1 therapy (Tavazoie et al. Citation2018). In this work, the PD-1+ and Tim-3+ tumor-infiltrating cells were reduced and increased TNF-α, IFN-γ and Granzyme B expressions were found in ApoE-deficient mice. This suggested that host ApoE deficiency could overcome T cell exhaustion, enhance cancer immunotherapy, and reduce tumor growth. Inhibition of ApoE may be a good strategy for tumor therapy.
Additionally, increased CD11c+ CD11b+ macrophages were increased in ApoE-deficient mice. Various reports indicated that in CD11c+ tumor-associated macrophages are pro-inflammatory M1 macrophages (Jeong et al. Citation2019; Suárez-Sánchez et al. Citation2020), which exert anti-tumor activity (Parisi et al. Citation2018). M1 macrophages have been reported to express cytotoxic cytokines including IFN-γ, GM-CSF, or TNF-α (Mantovani et al. Citation2005). The switch of M2-to-M1 macrophage polarization could repress tumor growth (Domínguez-Soto et al. Citation2014). Thus, host ApoE deficiency-induced increased M1 macrophage polarization may exert antitumor function and reduce the tumor growth. In addition, ApoE-deficient NK cells were reported to express higher levels of Granzyme B, Fas Ligand, IFN-γ, TNF-α, NKG2D, NKp46, and DNAM-1, which inhibited tumor growth (Lee et al. Citation2019). This suggested that the anti-tumor function of host ApoE deficiency possesses multiple mechanisms: (1) inhibition of T cell exhaustion, (2) enhancement of M1 macrophage polarization, and (3) upregulation of anti-tumor function of NK cells.
Interestingly, increased cholesterol and LDL levels were found in the blood of ApoE-deficient mice (Martins et al. Citation2006). The metabolomic profiles of ApoE −/− mice are quite similar to high fat diet-treated mice. However, high fat diet and obesity have been reported to repress the immune cell function and promote the tumor growth (Drijvers et al. Citation2020; Ringel et al. Citation2020). Obesity-induced abnormal metabolomics profiles could suppress anti-tumor immunity (Ringel et al. Citation2020). Therefore, the abnormal metabolomic profiles may not play an important role in host ApoE deficiency-induced anti-tumor function.
Leukocyte immunoglobulin-like receptor B4 (LILRB4), an immune inhibitory receptor, was expressed in variety of intratumoral immune cell types in murine tumor models and human cancers (Sharma et al. Citation2021). ApoE is one extracellular binding protein of LILRB4 (Deng et al. Citation2018). The disruption of LILRB4 and ApoE could block tumor development via (1) upregulation of T cell function, (2) enhancement of cellular cytotoxicity, and (3) increase of the cellular phagocytosis (Gui et al. Citation2019). Thus, the remodeling of immune landscape in ApoE −/− tumor microenvironment may be associated with the disassociation of ApoE and LILRB4. However, the relationship between LILRB4/ApoE and T cell exhaustion is still unknown. How LILRB4/ApoE-mediated T cell exhaustion is still needed to clarify.
In contrast to murine ApoE, human ApoE has one parent ApoE and three functional different isoforms: ApoE2, ApoE3, and ApoE4 (Phillips Citation2014). ApoE suppresses the production of pro-inflammatory cytokines by macrophages in an isoform-specific manner (E2 > E3 > E4) (Maezawa et al. Citation2006). And mice expressed humans ApoE4 isoform have increased circulating levels of activated T cells compared to those expressing ApoE2 or ApoE3 (Bonacina et al. Citation2018). Besides, ApoE derived peptides exhibit antibacterial, anti-biofilm, antiviral, anti-inflammatory, anti-atherogenic, antioxidant, or anticancer activities (Gaglione et al. Citation2020). An ApoE peptide comprising residues 133–162 exerts antimicrobial activity and anti-tumor functions (Azuma et al. Citation2000; Kojima et al. Citation2005). Another ApoE peptide, ApoE (133–167) can reduce lipopolysaccharide-induced cytokine release (Zanfardino et al. Citation2018). However, which ApoE isoform and ApoE derived peptides-mediated anti-tumor immunity function needs further clarify.
Conclusion
This work suggested that host ApoE deficiency could suppress tumor growth via inhibition of T cell exhaustion and enhancement of cytotoxic T cell function. Thus, inhibition of ApoE may be a good strategy for cancer immunotherapy.
Funding
This work was supported by the Science and Technology Project of Henan Province, P.R.C (grant number: 192102310090).
Data availability statement
The data that support the findings of this study are available in ‘figshare’ at https://doi.org/10.6084/m9.figshare.15260400.
Disclosure statement
No potential conflict of interest was reported by the author(s).
References
- Avila EM, Holdsworth G, Sasaki N, Jackson RL, Harmony JA. 1982. Apoprotein E suppresses phytohemagglutinin-activated phospholipid turnover in peripheral blood mononuclear cells. J Biol Chem. 257(10):5900–5909.
- Azuma M, Kojimab T, Yokoyama I, et al. 2000. A synthetic peptide of human apoprotein E with antibacterial activity. Peptides. 21(3):327–330.
- Bonacina F, Coe D, Wang G, Longhi MP, Baragetti A, Moregola A, Garlaschelli K, Uboldi P, Pellegatta F, Grigore L, et al. 2018. Myeloid apolipoprotein E controls dendritic cell antigen presentation and T cell activation. Nat Commun. 9(1):3083.
- Deng M, Gui X, Kim J, Xie L, Chen W, Li Z, He L, Chen Y, Chen H, Luo W, et al. 2018. LILRB4 signalling in leukaemia cells mediates T cell suppression and tumour infiltration. Nature. 562(7728):605–609.
- Domínguez-Soto A, de las Casas-Engel M, Bragado R, Medina-Echeverz J, Aragoneses-Fenoll L, Martín-Gayo E, van Rooijen N, Berraondo P, Toribio ML, Moro MA, et al. 2014. Intravenous immunoglobulin promotes antitumor responses by modulating macrophage polarization. J Immunol. 193(10):5181–5189.
- Drijvers JM, Sharpe AH, Haigis MC. 2020. The effects of age and systemic metabolism on anti-tumor T cell responses. Elife. 9:e62420.
- Gaglione R, Pizzo E, Notomista E, de la Fuente-Nunez C, Arciello A. 2020. Host defence cryptides from human apolipoproteins: applications in medicinal chemistry. Curr Top Med Chem. 20(14):1324–1337.
- Gui X, Deng M, Song H, Chen Y, Xie J, Li Z, He L, Huang F, Xu Y, Anami Y, et al. 2019. Disrupting LILRB4/APOE interaction by an efficacious humanized antibody reverses T-cell suppression and blocks AML development. Cancer Immunol Res. 7(8):1244–1257.
- Huvila J, Brandt A, Rojas CR, Pasanen S, Talve L, Hirsimäki P, Fey V, Kytömäki L, Saukko P, Carpén O, et al. 2009. Gene expression profiling of endometrial adenocarcinomas reveals increased apolipoprotein E expression In poorly differentiated tumors. Int J Gynecol Cancer. 19(7):1226–1231.
- Jeong H, Hwang I, Kang SH, Shin HC, Kwon SY. 2019. Tumor-associated macrophages as potential prognostic biomarkers of invasive breast cancer. J Breast Cancer. 22(1):38–51.
- Kojima T, Fujimitsu Y, Kojima H. 2005. Anti-tumor activity of an antibiotic peptide derived from apoprotein E. In Vivo. 19(1):261–264.
- Lee YS, Yeo IJ, Kim KC, Han SB, Hong JT. 2019. Inhibition of lung tumor development in ApoE knockout mice via enhancement of TREM-1 dependent NK cell cytotoxicity. Front Immunol. 10:1379.
- Maezawa I, Nivison M, Montine KS, Maeda N, Montine TJ. 2006. Neurotoxicity from innate immune response is greatest with targeted replacement of E4 allele of apolipoprotein E gene and is mediated by microglial p38MAPK. FASEB J. 20(6):797–799.
- Maimela NR, Liu S, Zhang Y. 2019. Fates of CD8+ T cells in tumor microenvironment. Comput Struct Biotechnol J. 17:1–13.
- Mantovani A, Sica A, Locati M. 2005. Macrophage polarization comes of age. Immunity. 23(4):344–346.
- Martins IJ, Hone E, Foster JK, Sünram-Lea SI, Gnjec A, Fuller SJ, Nolan D, Gandy SE, Martins RN. 2006. Apolipoprotein e, cholesterol metabolism, diabetes, and the convergence of risk factors for Alzheimer’s disease and cardiovascular disease. Mol Psychiatry. 11(8):721–736.
- McNeill E, Channon KM, Greaves DR. 2010. Inflammatory cell recruitment in cardiovascular disease: murine models and potential clinical applications. Clin Sci (Lond). 118(11):641–655.
- Munhoz RR, Postow MA. 2016. Recent advances in understanding antitumor immunity. F1000Res. 5:2545.
- Oue N, Hamai Y, Mitani Y, Matsumura S, Oshimo Y, Aung PP, Kuraoka K, Nakayama H, Yasui W. 2004. Gene expression profile of gastric carcinoma: identification of genes and tags potentially involved in invasion, metastasis, and carcinogenesis by serial analysis of gene expression. Cancer Res. 64(7):2397–2405.
- Parisi L, Gini E, Baci D, Tremolati M, Fanuli M, Bassani B, Farronato G, Bruno A, Mortara L. 2018. Macrophage polarization in chronic inflammatory diseases: killers or builders? J Immunol Res. 2018:1–25.
- Patil S, Rao RS, Majumdar B. 2015. T-cell exhaustion and cancer immunotherapy. J Int Oral Health. 7(8):i–ii.
- Phillips MC. 2014. Apolipoprotein E isoforms and lipoprotein metabolism. IUBMB Life. 66(9):616–623.
- Piedrahita JA, Zhang SH, Hagaman JR, Oliver PM, Maeda N. 1992. Generation of mice carrying a mutant apolipoprotein E gene inactivated by gene targeting In embryonic stem cells. Proc Natl Acad Sci U S A. 89(10):4471–4475.
- Ringel AE, Drijvers JM, Baker GJ, Catozzi A, García-Cañaveras JC, Gassaway BM, Miller BC, Juneja VR, Nguyen TH, Joshi S, et al. 2020. Obesity shapes metabolism in the tumor microenvironment to suppress anti-tumor immunity. Cell. 183(7):1848–1866.e26.
- Sharma N, Atolagbe OT, Ge Z, Allison JP. 2021. LILRB4 suppresses immunity in solid tumors and is a potential target for immunotherapy. J Exp Med. 218(7):e20201811.
- Su WP, Chen YT, Lai WW, Lin CC, Yan JJ, Su WC. 2011. Apolipoprotein E expression promotes lung adenocarcinoma proliferation and migration and as a potential survival marker in lung cancer. Lung Cancer. 71(1):28–33.
- Suárez-Sánchez FJ, Lequerica-Fernández P, Suárez-Canto J, Rodrigo JP, Rodriguez-Santamarta T, Domínguez-Iglesias F, García-Pedrero JM, de Vicente JC. 2020. Macrophages in oral carcinomas: relationship with cancer stem cell markers and PD-L1 expression. Cancers (Basel). 12(7):1764.
- Tavazoie MF, Pollack I, Tanqueco R, Ostendorf BN, Reis BS, Gonsalves FC, Kurth I, Andreu-Agullo C, Derbyshire ML, Posada J, et al. 2018. LXR/ApoE activation restricts innate immune suppression in cancer. Cell. 172(4):825–840.e18.
- Van Oosten M, Rensen PC, Van Amersfoort ES, Van Eck M, Van Dam AM, Brevé JJ, Vogel T, Panet A, Van Berkel TJ, Kuiper J. 2001. Apolipoprotein E protects against bacterial lipopolysaccharide-induced lethality: a new therapeutic approach to treat gram-negative sepsis. J Biol Chem. 276(12):8820–8824.
- Vitek MP, Snell J, Dawson H, Colton CA. 1997. Modulation of nitric oxide production in human macrophages by apolipoprotein-E and amyloid-beta peptide. Biochem Biophys Res Commun. 240(2):391–394.
- Wherry EJ. 2011. T cell exhaustion. Nat Immunol. 12(6):492–499.
- Zanfardino A, Bosso A, Gallo G, et al. 2018. Human apolipoprotein E as a reservoir of cryptic bioactive peptides: the case of ApoE 133-167. J Pept Sci. 24(7):e3095.
- Zhang HL, Wu J, Zhu J. 2010. The immune-modulatory role of apolipoprotein E with emphasis on multiple sclerosis and experimental autoimmune encephalomyelitis. Clin Dev Immunol. 2010:186813.