Abstract
Aflatoxin contamination in food commodities is a major food safety concern all over the world. These aflatoxins are a group of carcinogenic secondary metabolites produced by some fungi belonging to Aspergillus section Flavi. To minimize the level of aflatoxins in food commodities, a wide range of pre- and post-harvest procedures have been employed. In this study, aqueous extracts of 13 medicinal herbs were assessed for their ability to detoxify aflatoxin B1 (AFB1), the most potent and commonly occurring carcinogen in foods. Among them, herbal extracts of Hybanthus enneaspermus, Eclipta prostrata and Centella asiatica exhibited over 70% detoxification of AFB1. The degradation of AFB1 upon treatment with these herbal extracts was confirmed by liquid chromatography–mass spectrometry analysis. Two fractions (Rf 0.75 and 0.87) purified from H. enneaspermus by using thin-layer chromatography displayed in vitro AFB1-detoxifying properties. Gas chromatography–mass spectrometry analysis of the active fractions revealed the presence of linalool and bornyl acetate as the major components suggesting possible involvement of volatile compounds of H. enneaspermus in the detoxification of AFB1. To our knowledge, this is the first report on the detoxification of AFB1 by H. enneaspermus, E. prostrata and C. asiatica aqueous extracts.
Introduction
Aflatoxin contamination in agricultural products is of great concern to food safety all over the world (Kumar et al. Citation2017; Mahato et al. Citation2019). Aflatoxins are carcinogenic products predominantly released by Aspergillus flavus Link and A. parasiticus Speare, members of Aspergillus section Flavi, during their invasion on susceptible crops under favorable conditions. Aflatoxin contamination has been reported in several agricultural commodities including corn, peanut, rice, chili, cottonseed, cashew, pistachios, hazelnuts, almonds, cocoa beans and dried figs (Mahato et al. Citation2019; Rushing and Selim Citation2019; Kumar et al. Citation2021). Ingestion of food or feed contaminated with excessive levels of aflatoxin leads to ‘aflatoxicosis’ in humans and animals (Sarma et al. Citation2017).
The aflatoxigenic fungi invade the agricultural commodities in the field before harvest or during post-harvest processes and storage and secrete aflatoxins. More than 20 different types of aflatoxins have been characterized (Kumar et al. Citation2017). The major aflatoxins that are commonly found in agricultural products are aflatoxin B1 (AFB1) and aflatoxin B2 (AFB2), secreted by A. flavus and A. parasiticus and aflatoxin G1 (AFG1) and aflatoxin G2, secreted by A. parasiticus. Aflatoxin M1 (AFM1), which is the main metabolite of AFB1, is often found in milk of animals that have consumed AFB1-contaminated feed (Kumar et al. Citation2017). Among the various types of aflatoxins, AFB1 has been described as the highly carcinogenic mycotoxin to animals and humans (Roze et al. Citation2013; Adam et al. Citation2017). AFB1 is converted into aflatoxin B1-8,9-epoxide (AFBO) by the action of cytochrome P450 present in the liver. The AFBO, which is a highly reactive and unstable molecule, binds to DNA (causing genotoxicity) and proteins (causing cytotoxicity). The AFBO binds to guanine residues in DNA and forms aflatoxin-N7-guanine that results in guanine (G) to thymine (T) transversion mutations. A mutation in the 249th codon of the tumor suppressor gene p53 leads to hepatocellular carcinoma (HCC) or liver cancer (Adam et al. Citation2017). AFB1 is also known to affect kidney, heart, testis, brain, lungs and the entire respiratory system (Denissenko et al. Citation1999). In addition, AFB1 has been shown to cause malnutrition, growth impairment and suppression of immune system in humans and animals (Rushing and Selim Citation2019; Khan et al. Citation2021). Recent studies suggest that long-term exposure to aflatoxins may lead to neurodegenerative diseases (Khan et al. Citation2021). In order to safeguard the consumers, over 100 countries established regulations for aflatoxins in food (van Egmond et al. Citation2007). The USA and European Union set regulatory limits of 20 and 4 ppb for foods for human consumption, respectively (Kaale et al. Citation2021).
Aflatoxins are highly stable under different storage and processing conditions of foods or feeds. Aflatoxin contamination in the agricultural commodities at the pre-harvest stage can be prevented by planting resistant crop cultivars, crop rotation, insect management, proper irrigation and fertilization and early harvesting (Adeyeye Citation2016). At the post-harvest stage, strategies such as reducing moisture content of the agricultural products, proper storage, treatment with electromagnetic radiation, ozone fumigation, adoption of improved sorting technologies and detoxification can minimize aflatoxins in agricultural commodities to acceptable levels (Udomkun et al. Citation2017). Various biological, physical and chemical detoxification methods have been used to minimize aflatoxins content in foods and feeds (Nazhand et al. Citation2020). These methods are based mainly on degradation, modification and adsorption of aflatoxins. However, each method has its limitations. The use of plant products for the detoxification of aflatoxins has been suggested as a biologically safe and cost-effective method (Velazhahan Citation2017). Aflatoxin detoxification potential of medicinal plants such as Trachyspermum ammi (Hajare et al. Citation2005; Velazhahan et al. Citation2010; Iram et al. Citation2016a), Zimmu (Allium sativum x A. cepa) (Sandosskumar et al. Citation2007), Thymus daenensis (Goran et al. Citation2013), Adhatoda vasica (Vijayanandraj et al. Citation2014), Barleria lupulina (Kannan and Velazhahan Citation2014), Corymbia citriodora (Iram et al. Citation2015), Ocimum basilicum (Iram et al. Citation2016b), Rosmarinus officinalis, Origanum vulgare and Psidium cattleianum (Ponzilacqua et al. Citation2019) has been documented. The objective of this study was to evaluate the AFB1-detoxifying potential of 13 herbal medicinal products that are widely used in the traditional medicine for the treatment of various ailments including cough, cold and asthma.
Materials and methods
Medicinal herbs
Dry powders of 13 medicinal herbs viz., Alpinia officinarum Hance. (Zingiberaceae), Cardiospermum halicacabum Linn. (Sapindaceae), Centella asiatica (L.) Urban (Apiaceae), Eclipta prostrata (L.) L. (Asteraceae), Glycyrrhiza glabra L. (Fabaceae), Hybanthus enneaspermus (L.) F. Muell (Violaceae), Myristica fragrans Houtt. (Myristicaceae), Phyllanthus niruri L. (Phyllanthaceae), Piper longum L. (Piperaceae), Quercus infectoria Oliv. (Fagaceae), Solanum trilobatum L. (Solanaceae), Syzygium cumini (L.) Skeels (Myrtaceae) and Terminalia chebula Retz. (Combretaceae) were obtained from herbal medical stores. The samples were stored in airtight containers at 4°C.
Aflatoxin
The AFB1 standard (purified from Aspergillus flavus) was purchased from Sigma-Aldrich, St. Louis, MO, USA. The stock solution of AFB1 (1000 μg ml−1) was prepared in methanol, wrapped in aluminum foil and stored at 4°C.
Preparation of extracts
One gram of herbal powder was mixed with 5 ml of sterile distilled water (SDW) and allowed to stand overnight at 4°C. After incubation, the mixture was centrifuged at 12,000×g for 15 min at 4°C and the supernatant was collected. The volume of the extract was made up to 5 ml with SDW.
Determination of AFB1 detoxification
AFB1 detoxification potential of herbal extracts was determined as described by Velazhahan et al. (Citation2010) with some modifications. Briefly, 250 μl of plant extract in a 1.5-ml centrifuge tube was mixed with 50 μl of AFB1 (50 μg/l) and incubated at 37°C in a water bath. After 48 h, 250 μl of chloroform was added, vortexed and then centrifuged at 12,000×g for 15 min. The chloroform layer was collected in a new tube and evaporated at 60°C in a water bath. The residue was suspended in 50 μl of 70% methanol and AFB1 content was determined by ELISA (RIDASCREEN Aflatoxin B1 30/15, Lot No. 21240; R-Biopharm AG, Darmstadt, Germany) by following the manufacturer’s instructions. SDW (250 μl) mixed with 50 μl of AFB1 (50 μg/l) and processed under the same conditions served as control. Three replicates were used for each treatment.
Analysis of the degraded products of AFB1
The degraded products of AFB1 after treatment with herbal extracts were analyzed by Agilent LC/MS/MS, equipped with high-performance autosampler (G4226A), quaternary pump (G4204A), thermostated column compartment (G1316C) and 6460 Triple Quad MS detector. Chromatographic separations were performed with Symmetry C8 5 µm, 3 mm × 150 mm column (Waters). The column was maintained at 45°C. The injection volume was 5 µl. The mobile phase used was acetonitrile (eluent A) and water (eluent B), both with 0.1% formic acid under gradient condition (eluent A 10–70% in 0–1 min, 70–95% in 1–2 min, held at 95% for 4 min, 95–70% in 6–7.5 min, 70–10% in 7.5–8 min and hold at 10% for 1 min) with a flow rate of 0.3 ml per min. The following MS detector parameters were used for the analysis: gas temperature 300°C, gas flow 3 L min−1, nebulizer pressure 50 PSI, sheath gas heater 375°C, sheath gas flow 10 L min−1, capillary voltage 3500 V, scan range 100–3000 m/z and positive polarity. Mass spectra data were acquired and processed by Agilent MassHunter workstation and Agilent MassHunter qualitative analysis software, respectively.
Analysis of AFB1-detoxifying compounds
Thin-layer chromatography (TLC)
One gram of herbal powder was mixed with 5 ml of methanol, vortexed and centrifuged at 12,000×g for 15 min at 4°C. Aliquots (10 µl) of the supernatant were applied on silica gel 60 F254 TLC plate (Merck KGaA, Darmstadt, Germany) and the plates were developed in chloroform: ethyl acetate: formic acid (10:8:2, v/v/v). After developing the plates, they were dried, observed under UV light (254 nm) and Rf values of each spot were calculated. Silica gel at each spot was collected separately into a 1.5-ml tube by gently scrapping the preparative TLC plate. One ml spectrophotometric grade methanol was added to each tube, mixed well by vortexing and centrifuged at 12,000×g for 10 min. The supernatant was collected and analyzed for detoxification of AFB1 by ELISA as described before. Methanol (250 μl) mixed with 50 μl of AFB1 (50 μg/l) and processed under the same conditions served as control. Three replicates were used for each treatment.
Gas chromatography–mass spectrometry (GC–MS) analysis
The TLC fractions with Rf values of 0.75 and 0.87 obtained from H. enneaspermus extract that showed high aflatoxin detoxification ability were analyzed using a Shimadzu GC-2010 Plus, equipped with an Rtx-5MS capillary column (30 m length, 0.25 mm i.d., 0.25 μm film thickness) and GCMS-QP2010 ULTRA MS. One μl of the sample with a split ratio of 100:1 was injected into the column. The flow rate of helium gas was set at 1.0 ml min−1. The temperatures of injection port, transfer line and ion source were 280°C, 270°C and 270°C, respectively. The ionization electron energy was set to 70 eV. All data were obtained by collecting the full-scan mass spectra with the scan range 40–550 amu. The oven temperature settings were programmed to begin with 42°C, followed by an increase at a rate of 5.5°C min−1 till 330°C and then held for 10 min. The total run time was 63.5 min. The NIST 2011 v.2.3 and Wiley 9th edition mass spectrum libraries were used to identify the compounds.
Statistical analysis
The data were analyzed using general linear model ANOVA and the differences between the means were determined using Tukey’s test at P ≤ 0.05 (Minitab v17; Minitab Inc., State College, PA, USA).
Results and discussion
The aqueous extracts of 13 herbal powders were assessed for their ability to degrade AFB1 under in vitro conditions. Among them, the extracts of H. enneaspermus exhibited the maximum degradation of AFB1 (77%) after 48 h of incubation at 37°C, followed by E. prostrata (76%) and C. asiatica (72%). The rest of the herbal extracts showed less than 70% detoxification of AFB1. The extract of P. longum was the least effective (1.5%) in AFB1 detoxification (Table ). Vijayanandraj et al. (Citation2014) reported that aqueous extract from the leaves of Adhatoda vasica showed degradation of above 98% of AFB1 after 24 h of incubation at 37°C. Iram et al. (Citation2015) demonstrated that aqueous extract from the leaves of Corymbia citriodora showed 95.21% degradation of AFB1. Negera and Washe (Citation2019) while studying the AFB1 degradation efficacy of different dietary spices showed that the aqueous extracts of Allium sativum and Citrus limon caused 61.7% and 56% degradation of AFB1, respectively after 1 h of treatment at 37°C. Ponzilacqua et al. (Citation2019) demonstrated that the aqueous extracts of Rosmarinus officinalis, Origanum vulgare and Psidium cattleianum exhibited AFB1 degradation of 60.3%, 38.3% and 30.0%, respectively after 48 h of incubation at 37°C. However, the detoxification of AFB1 by H. enneaspermus, E. prostrata and C. asiatica has not been reported so far. The degradation of AFB1 in this study might be due to water-soluble bioactive compounds present in the herbal extracts. The three herbal extracts viz., H. enneaspermus, E. prostrata and C. asiatica that showed high levels of detoxification of AFB1 were selected for further studies. To confirm the degradation of AFB1 by herbal extracts, AFB1 treated with herbal extracts was analyzed by liquid chromatography–mass spectrometry. Mass spectrum of untreated AFB1 (control) showed the molecular ion m/z 313 that is specific to AFB1 (Ventura et al. Citation2006) (Figure A, Figure A and Figure A). The molecular ion m/z 313 was not detected in the herbal extracts (Figure B, Figure B and Figure B) confirming the absence of AFB1. However, after treatment with herbal extracts, the level of AFB1 was reduced to undetectable levels and a fragment ion (m/z 189) appeared confirming degradation of AFB1 (Figure C, Figure C and Figure C). These results are in agreement with the findings of Vijayanandraj et al. (Citation2014) who reported the disappearance of molecular base ion at m/z 313 and appearance of a product ion at m/z 189.59 after incubation with A. vasica extract. The expected fragmentation pathway of AFB1 after treatment with the herbal extracts is depicted in Figure . It shows two sequential losses of carbon monoxide (CO) as the main fragmentation pathway. The latter fragment might lose the methoxy moiety, on the aromatic system, and a carbon monoxide and a formaldehyde moiety. This suggested pathway of degradation is based on the major fragment peaks that appear in the mass spectrum and following fragmentation pathways of similar structures. Similar results were reported by Iram et al. (Citation2016b) while analyzing the degraded products of AFB1 by Ocimum basilicum extract. LC–MS/MS analysis of AFB1 after treatment with O. basilicum extract showed that continuous loss of carbon monoxide (CO) was the principal fragmentation pathway. Further, methyl and methanol losses occurred on the methoxy group located on the side chain of benzene. Several studies have described the molecular structures of degradation products of aflatoxins (Cucullu et al. Citation1976; Mendez-Albores et al. Citation2008; Velazhahan et al. Citation2010; Iram et al. Citation2016a). The production of hydroxydihydro-aflatoxin B1, a fluorescing compound, from AFB1 after treatment with acid-producing fungi has been reported (Ciegler and Peterson Citation1968). The formation of dihydro-4-hydroxy-6- methoxyfuro [2,3-b] benzofuran with a molecular weight of 206 from AFB1 upon ammoniation has been documented (Cucullu et al. Citation1976). Lee et al. (Citation1974) reported the formation of a product with a molecular mass of 286 from AFB1 after treatment with NH4OH. Mendez-Albores et al. (Citation2008) reported the production of a compound lacking the lactone group (m/z 286) and a compound holding the difurane moiety but deficient in the lactone carbonyl and the cyclopentenone ring (m/z 206) as degradation products of AFB1 following treatment with citric acid. Alterations in the lactone ring structure of AFG1 have been suggested as the mechanism in the AFG1 detoxification by T. ammi seed extracts (Velazhahan et al. Citation2010). Iram et al. (Citation2016a) analyzed the degraded products of AFB1 and AFB2 after treatment with seed extract of T. ammi and suggested that most of these products were produced by the elimination of the double bond in the furan ring and alteration of the lactone group. The disappearance of ion peak at m/z 313 (specific to AFB1) in response to treatment with aqueous extracts of medicinal herbs in this study suggests the degradation of AFB1.
Figure 1. Mass spectra of AFB1 after treatment with aqueous extract of C. asiatica. (A) untreated AFB1 (control); (B) C. asiatica extract alone; (C) AFB1 after treatment with C. asiatica extract.
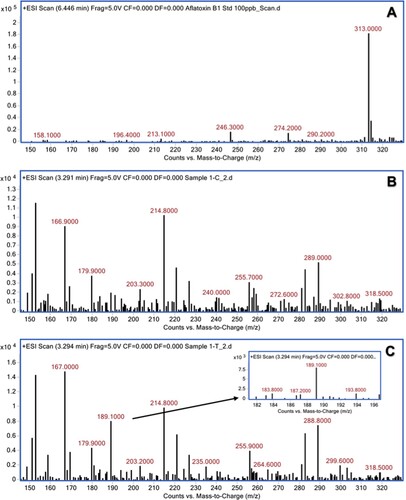
Figure 2. Mass spectra of AFB1 after treatment with aqueous extract of H. enneaspermus. (A) untreated AFB1 (control); (B) H. enneaspermus extract alone; (C) AFB1 after treatment with H. enneaspermus extract.
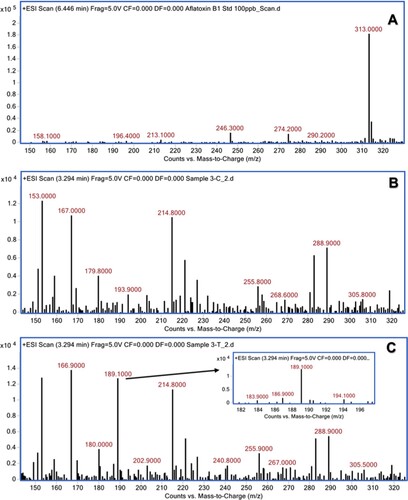
Figure 3. Mass spectra of AFB1 after treatment with aqueous extract of E. prostrata. (A) untreated AFB1 (control); (B) E. prostrata extract alone; (C) AFB1 after treatment with E. prostrata extract.
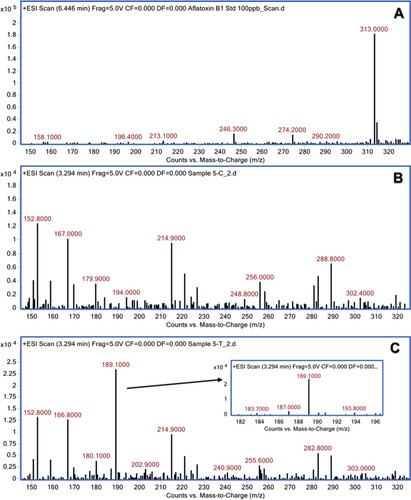
Table 1. Detoxification of aflatoxin B1 by aqueous extracts of medicinal herbs.
To identify the aflatoxin-detoxifying principle(s), methanolic extracts of the herbal powders were analyzed by TLC. Under UV light (254 nm), 3 prominent bands (Rf 0.75, Rf 0.80 and Rf 0.87) in C. asiatica, 2 bands (Rf 0.75 and Rf 0.87) in H. enneaspermus and 5 bands (Rf 0.62, Rf 0.74, Rf 0.80, Rf 0.87, Rf 0.90) in E. prostrata were identified (Figure ). Analysis of these compounds for their AFB1-detoxifying ability revealed that the fractions with Rf values of 0.87 and 0.75 obtained from H. enneaspermus were efficacious and caused 60.4 and 90.8% detoxification of AFB1, respectively (Table ). GC–MS analysis showed the presence of linalool (46.5%) and bornyl acetate (53.4%) in fraction 1 with an Rf of 0.75; Fraction 2 with an Rf of 0.87 contained linalool (63.5%), bornyl acetate (33.68%) and methyl oleate (2.78%) (Figure ; Table ). Linalool, an acyclic monoterpene, has been reported to be present in the essential oils of over 200 plant species (Stashenko and Martinez Citation2008). Antimicrobial effect of linalool (Park et al. Citation2012) and anti-inflammatory activity of linalool-containing essential oils have been reported earlier (Peana et al. Citation2002). Similarly, bornyl acetate has been identified as a major constituent of essential oil isolated from Tetraclinis articulate that exhibited antioxidant and antibacterial activities (Rabib et al. Citation2020). However, the role of linalool and bornyl acetate in the detoxification of AFB1 has not been reported so far.
Figure 5. TLC analysis of methanolic extracts of C. asiatica, H. enneaspermus and E. prostrata. Herbal powder (1 g) was extracted with methanol (5 ml) and 10 µl of the extract was analyzed by TLC using chloroform: ethyl acetate: formic acid (10:8:2) as mobile phase and viewed under UV light: (A) C. asiatica extract; (B) H. enneaspermus extract; (C) E. prostrata extract.
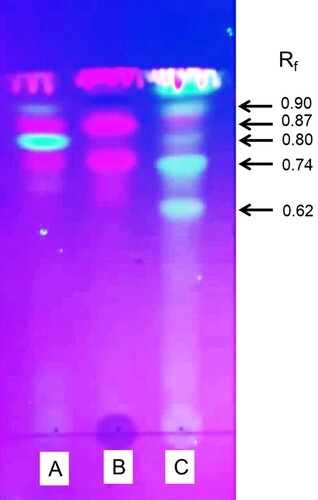
Table 2. Effect of purified compounds from the methanolic extracts of medicinal herbs on detoxification of AFB1.
Table 3. Characterization of the purified fractions from H. enneaspermus by GC–MS analysis.
C. asiatica, E. prostrata and H. enneaspermus have been used in traditional medicine to treat various kinds of illnesses in humans. C. asiatica has been used for wound healing, memory enhancement and to treat various diseases including cardiovascular diseases, endocrine diseases, gastrointestinal diseases, gynecological diseases, neurological diseases and skin diseases (Sun et al. Citation2020). Saponins are the major constituents in C. asiatica (Gohil et al. Citation2010). H. enneaspermus has been used as aphrodisiac, anticonvulsant, antitussive and demulcent and for treating gonorrhoea, urinary infections, strangury, epilepsy, urethral discharges, asthma and cough (Hemalatha et al. Citation2003; Boominathan et al. Citation2004; Sahoo et al. Citation2006). H. enneaspermus is known to contain alkaloids, anthraquinones, L-DOPA, diosgenin, flavones, flavonoids, phenols and triterpenes (Patel et al. Citation2013; Sivanandhan et al. Citation2015). E. prostrata has been used to treat abnormal uterine bleeding, aching of the knees and loins, liver cirrhosis, jaundice, hematuria, diarrhea and epistaxis (Ying et al. Citation2008). To our knowledge, this is the first study to demonstrate the AFB1-detoxifying properties of C. asiatica, E. prostrata and H. enneaspermus.
Conclusion
Our data showed that the aqueous extracts of C. asiatica, E. prostrata and H. enneaspermus were highly efficient in detoxifying AFB1 under laboratory conditions. GC–MS analysis of the fractions purified from the methanolic extract of H. enneaspermus that showed high AFB1-detoxifying ability, revealed the presence of linalool and bornyl acetate as the major constituents. Plant-derived bioactive compounds are generally recognized as safe (GRAS). Hence, these herbal products may be highly useful for the development of functional foods or biologically safe herbal feed additives to reduce the toxic effects of AFB1 in livestock. Currently, we are studying the preventive effect of these herbal products on aflatoxin B1-induced toxicity in animal models. Further studies are required to evaluate the efficacy of these herbal extracts in detoxification of other major aflatoxins viz., AFB2, AFG1 and AFG2, to determine the biological toxicity of the degraded products of aflatoxins and to assess the role of linalool and bornyl acetate in the detoxification of aflatoxins.
Author contributions
RV, AMA, JNA designed the study, AA, SPS, MMAA, IHA conducted lab experiments, RV, AMA, JNA supervised the research project, RV, AMA, JNA, SPS, RAJ wrote the manuscript.
Ethical approval
The authors confirm that there are no ethical issues in the publication of the manuscript.
Acknowledgment
We thank Ms. Huda Khalfan Al-Ruqaishi, Central Instrument Laboratory, College of Agricultural and Marine Sciences, Sultan Qaboos University for helping in GC-MS analysis. The authors thank Dr. S. Muthukrishnan, Kansas State University, USA for critically reading the manuscript.
Disclosure statement
No potential conflict of interest was reported by the author(s).
Data availability
All data generated or analyzed during this study are included in this article. The data supporting the findings of this study are available in Mendeley data, V3, DOI: 10.17632/4ysv5stvps.3 (https://data.mendeley.com/datasets/4ysv5stvps/3).
Additional information
Funding
References
- Adam MAA, Tabana YM, Musa KB, Sandai DA. 2017. Effects of different mycotoxins on humans, cell genome and their involvement in cancer. Oncol Rep. 37:1321–1336.
- Adeyeye SA. 2016. Fungal mycotoxins in foods: A review. Cogent Food Agric. 2:1213127.
- Boominathan R, Parimaladevi B, Mandal SC, Ghoshal SK. 2004. Anti-inflammatory evaluation of Ionidium suffruticosam ging. in rats. J Ethnopharmacol. 91:367–370.
- Ciegler A, Peterson RE. 1968. Aflatoxin detoxification:hydroxydihydro-aflatoxin B. Appl Microbiol. 16:665–666.
- Cucullu AF, Lee LS, Pons Jr WA, Stanley JB. 1976. Ammoniation of aflatoxin B1. Isolation and characterization of a product with molecular weight 206. J Agric Food Chem. 24:408–410.
- Denissenko MF, Cahill J, Koudriakova TB, Gerber N, Pfeifer GP. 1999. Quantitation and mapping of aflatoxin B1-induced DNA damage in genomic DNA using aflatoxin B1-8, 9-epoxide and microsomal activation systems. Mutat Res Fundam Mol Mech Mutagen. 425:205–211.
- Gohil KJ, Patel JA, Gajjar AK. 2010. Pharmacological review on Centella asiatica: a potential herbal cure-all. Indian J Pharm Sci. 72:546.
- Gorran A, Farzaneh M, Shivazad M, Rezaeian M, Ghassempour A. 2013. Aflatoxin B1-reduction of Aspergillus flavus by three medicinal plants (Lamiaceae). Food Control. 31:218–223.
- Hajare SS, Hajare SH, Sharma A. 2005. Aflatoxin inactivation using aqueous extract of Ajowan (Trachyspermum ammi) seeds. J Food Sci. 70:29–34.
- Hemalatha S, Wahi AK, Singh PN, Chansouria JPN. 2003. Anticonvulsant and free radical scavenging activity of Hybanthus enneaspermus: a preliminary screening. Indian J Tradit Knowl. 2:383–388.
- Iram W, Anjum T, Iqbal M, Ghaffar A, Abbas M. 2015. Mass spectrometric identification and toxicity assessment of degraded products of aflatoxin B1 and B2 by Corymbia citriodora aqueous extracts. Sci Rep. 5:14672.
- Iram W, Anjum T, Iqbal M, Ghaffar A, Abbas M. 2016a. Structural elucidation and toxicity assessment of degraded products of aflatoxin B1 and B2 by aqueous extracts of Trachyspermum ammi. Front Microbiol. 7:346.
- Iram W, Anjum T, Iqbal M, Ghaffar A, Abbas M, Khan AM. 2016b. Structural analysis and biological toxicity of aflatoxins B1 and B2 degradation products following detoxification of Ocimum basilicum and Cassia fistula aqueous extracts. Front Microbiol. 7:1105.
- Kaale LD, Kimanya ME, Macha IJ, Mlalila N. 2021. Aflatoxin contamination and recommendations to improve its control: a review. World Mycotoxin J. 14:27–40.
- Kannan K, Velazhahan R. 2014. The potential of leaf extract of Barleria lupulina for detoxification of aflatoxins. Indian Phytopathol. 67:298–302.
- Khan R, Ghazali FM, Mahyudin NA, Samsudin NIP. 2021. Aflatoxin biosynthesis, genetic regulation, toxicity, and control strategies: A review. J Fungi. 7:606.
- Kumar A, Pathak H, Bhadauria S, Sudan J. 2021. Aflatoxin contamination in food crops: causes, detection, and management: A review. Food Prod Process Nutr. 3:7.
- Kumar P, Mahato DK, Kamle M, Mohanta TK, Kang SG. 2017. Aflatoxins: A global concern for food safety, human health and their management. Front Microbiol. 7:2170.
- Lee LS, Stanley JB, Cucullu AF, Pons WA, Goldblatt LA. 1974. Ammoniation of aflatoxin B1: Isolation and identification of the major reaction product. J Assoc Off Anal Chem. 57:626–631.
- Mahato DK, Lee KE, Kamle M, Devi S, Dewangan KN, Kumar P, Kang SG. 2019. Aflatoxins in food and feed: an overview on prevalence, detection and control strategies. Front Microbiol. 10:2266.
- Mendez-Albores A, Nicolas-Vazquez I, Miranda-Ruvalcaba R, Moreno-Martinez E. 2008. Mass spectrometry/mass spectrometry study on the degradation of B-aflatoxins in maize with aqueous citric acid. Am J Agric Biol Sci. 3:482–489.
- Nazhand A, Durazzo A, Lucarini M, Souto EB, Santini A. 2020. Characteristics, occurrence, detection and detoxification of aflatoxins in foods and feeds. Foods. 9:644.
- Negera M, Washe AP. 2019. Use of natural dietary spices for reclamation of food quality impairment by aflatoxin. J Food Qual. 2019:4371206.
- Park SN, Lim YK, Freire MO, Cho E, Jin D, Kook JK. 2012. Antimicrobial effect of linalool and α-terpineol against periodontopathic and cariogenic bacteria. Anaerobe. 18:369–372.
- Patel DK, Kumar R, Sairam K, Hemalatha S. 2013. Hybanthus enneaspermus (L.) F. Muell: a concise report on its phytopharmacological aspects. Chin J Nat Med. 11:199–206.
- Peana AT, D'Aquila PS, Panin F, Serra G, Pippia P, Moretti MDL. 2002. Anti-inflammatory activity of linalool and linalyl acetate constituents of essential oils. Phytomedicine. 9:721–726.
- Ponzilacqua B, Rottinghaus GE, Landers BR, Oliveira CAF. 2019. Effects of medicinal herb and Brazilian traditional plant extracts on in vitro mycotoxin decontamination. Food Control. 100:24–27.
- Rabib H, Elagdi C, Hsaine M, Fougrach H, Koussa T, Badri W. 2020. Antioxidant and antibacterial activities of the essential oil of Moroccan Tetraclinis articulata (Vahl) masters. Biochem Res Int. 2020: ID 9638548.
- Roze LV, Hong SY, Linz JE. 2013. Aflatoxin biosynthesis: current frontiers. Annu Rev Food Sci Technol. 4:293–311.
- Rushing BR, Selim MI. 2019. Aflatoxin B1: A review on metabolism, toxicity, occurrence in food, occupational exposure, and detoxification methods. Food Chem Toxicol. 124:81–100.
- Sahoo S, Kar DM, Mohapatra S, Rout SP, Dash SK. 2006. Antibacterial activity of Hybanthus enneaspermus against selected UTI pathogens. Indian J Pharm Sci. 68:653–655.
- Sandosskumar R, Karthikeyan M, Mathiyazhagan S, Mohankumar M, Chandrasekar G, Velazhahan R. 2007. Inhibition of Aspergillus flavus growth and detoxification of aflatoxin B1 by the medicinal plant zimmu (Allium sativum L. x Allium cepa L.). World J Microbiol Biotechnol. 23:1007–1014.
- Sarma UP, Bhetaria PJ, Devi P, Varma A. 2017. Aflatoxins: implications on health. Indian J Clin Biochem. 32:124–133.
- Sivanandhan G, Vasudevan V, Selvaraj N, Lim YP, Ganapathi A. 2015. L-Dopa production and antioxidant activity in Hybanthus enneaspermus (L.) F. Muell regeneration. Physiol Mol Biol Plants. 21:395–406.
- Stashenko EE, Martínez JR. 2008. Sampling flower scent for chromatographic analysis. J Sep Sci. 31:2022–2031.
- Sun B, Wu L, Wu Y, Zhang C, Qin L, Hayashi M, Kudo M, Gao M, Liu T. 2020. Therapeutic potential of Centella asiatica and its triterpenes: a review. Front Pharmacol. 11:1373.
- Udomkun P, Wiredu AN, Nagle M, Müller J, Vanlauwe B, Bandyopadhyay R. 2017. Innovative technologies to manage aflatoxins in foods and feeds and the profitability of application–A review. Food Control. 76:127–138.
- van Egmond HP, Schothorst RC, Jonker MA. 2007. Regulations relating to mycotoxins in food. Anal Bioanal Chem. 389:147–157.
- Velazhahan R. 2017. Bioprospecting of medicinal plants for detoxification of aflatoxins. Int J Nutr Pharmacol Neurol Dis. 7:60–63.
- Velazhahan R, Vijayanandraj S, Vijayasamundeeswari A, Paranidharan V, Samiyappan R, Iwamoto T, Friebe B, Muthukrishnan S. 2010. Detoxification of aflatoxins by seed extracts of the medicinal plant, Trachyspermum ammi (L.) Sprague ex Turrill – Structural analysis and biological toxicity of degradation product of aflatoxin G1. Food Control. 21:719–725.
- Ventura M, Guillen D, Anaya I, Broto-Puig F, Lliberia JL, Agut M, Comellas L. 2006. Ultra-performance liquid chromatography/tandem mass spectrometry for the simultaneous analysis of aflatoxins B1, G1, B2, G2 and ochratoxin A in beer. Rapid Commun Mass Spectrom. 20:3199–3204.
- Vijayanandraj S, Brinda R, Kannan K, Adhithya R, Vinothini S, Senthil K, Ramakoteswara Rao C, Paranidharan V, Velazhahan R. 2014. Detoxification of aflatoxin B1 by an aqueous extract from leaves of Adhatoda vasica Nees. Microbiol Res. 169:294–300.
- Ying DL. 2008. The research progress in chemical constituents, pharmacological effects and clinic application of Eclipta prostrata. China Pharm. 19:2876–2877.