Abstract
Emamectin benzoate (EMB) is one of the high-efficacious pesticide commonly used to control the ferocious economic crop pest S. exigua in the field. The results of S. exigua life table treated with three lethal doses of EMB (LC5, LC20 and LC50) indicated that three lethal doses of EMB could all inhibited the proliferation of S. exigua population effectively by long-lasting inhibit the survival of the S. exigua larvae and per female fecundity significantly (p < 0.05), and the inhibition effects of key life parameters were affected by EMB stress in a dose-dependent manner. 67,123 unigenes with an N50 value of 1941 bp were assembled by RNA-seq data analyses and 44,113 were expressed more than 12 samples. Furthermore, 3517, 3139 and 5135 differentially expressed genes were identified under different lethal doses of EMB treatment. Finally, 16 hub genes and three olfaction-related genes were identified by WGCNA analysis as biomarkers for the EMB response, and the cAMP-dependent proteins, P450 metabolic-related genes and ABC transporter genes might have been involved in response to EMB stress in S. exigua.
Introduction
The beet armyworm, Spodoptera exigua (Hübner) which originated from south-east Asia has now become an important worldwide agricultural pest (Hafeez et al. Citation2020). As a polyphagous pest, S. exigua has been identified to be detrimental to over 130 host plants species, as it causes considerable losses to economic crops, such as Chinese cabbage, soybean, peanut, onion, corn, scallion, ginger, pepper, sorghum, tobacco and cotton (Moulton et al. Citation2002; Zheng et al. Citation2011; Zhou et al. Citation2011; Hafeez et al. Citation2021).
The key stage for the effective control of S. exigua is before the 3rd instar stage, because the larvae at this stage are small, have poor drug resistance, and are easy to kill. After the 3rd instar, the resistance of larvae against pesticides increases. S. exigua also exhibits a phenomenon known as false death, which makes this pest difficult to control (Zhang et al. Citation2011). In field, various control measures have been applied to deal with S. exigua damage, such as biological, physical, chemical as well as mechanical control (Akter et al. Citation2019). Among these methods, chemical control, which relies on the spraying of different pesticides such as carbamate (methomyl), pyrethroid (fenpropathrin), organophosphate (chlorpyrifos), and biopesticide (Emamectin benzoate, abbreviated as EMB), is the major strategy (Liburd et al. Citation2000; Ishaaya et al. Citation2002; Huang et al. Citation2021).
Currently, chlorantraniliprole, deltamethrin, cyhalothrin, indoxacarb, pyrethroid, spinetoram, and EMB are widely used to control the S. exigua in China (Wang et al. Citation2019b; Lai et al. Citation2011). Due to the excessive and frequent application of pesticides, S. exigua has developed different resistance levels against most conventional pesticides around the world (Hartman and Clair Citation1999). For example, manual selection of S. exigua carried out in the laboratory with chlorantraniliprole resulted in an increase in resistance by 11.8-fold after continuous screening for 23 generations, and by 128.6-fold compared to the susceptible strains kept in the laboratory (Lai et al. Citation2011). Another study indicated that S. exigua developed a 309.5-fold resistance to lambda-cyhalothrin, and selection in the laboratory resulted in a 423-fold increase in resistance (Tian et al. Citation2018). Since S. exigua has developed high resistance to these conventional pesticides, there is an urgent need for highly effective pesticides in the field.
Previous studies have shown that among the different conventional insecticides, EMB is an excellent pesticide that can be used to control S. exigua in the field efficiently (López et al. Citation2010; ElSheikh Citation2015; Moustafa et al. Citation2016). As a widely used macrocyclic lactone insecticide, EMB ((4″R)-4″-deoxy-4″-(methylamino) avermectin B1 benzoate) is a biopesticide extracted from the naturally-occurring compound, ivermectin, that has high efficiency in controlling many economic crop pests (Mushtaq et al. Citation1996; López et al. Citation2010; Mokbel and Huesien Citation2020). The action mode of EMB is as a glutamate-gated chloride channel allosteric modulator (IRAC Citation2019), which disturbs the nervous and muscular system of insects, ultimately resulting in their deaths (Ishaaya et al. Citation2002; Zaka et al. Citation2014). EMB possesses excellent insecticidal potency, which can be up to 80,400 more effective compared to conventional pesticides such as fipronil, indoxacarb, cypermethrin, and fenvalerate, used on economic crops (Argentine et al. Citation2002; Ishaaya et al. Citation2002; López et al. Citation2010).
Insects mainly rely on the olfactory system to identify a variety of environmental chemicals, including pesticides and other exogenous substances. A study reported that the binding affinity of two A. lepigone pheromone binding proteins (AlepPBP2 and AlepPBP3) to phoxim is higher than that of other insecticides (Zhang et al. Citation2020a). After the amino acid residues of the protein were changed by site-directed mutagenesis, the binding affinity of AlepPBP2 and AlepPBP3 to phoxim decreased, indicating that they should play a key role in the AlepPBPs/phoxim interaction. Therefore, the relationship between the effect of pesticides on insects and the sense of smell of insects is worthy of further study. ABC transporters exist in all areas of life and have the function of natural excretion. Ryan C. Gott et al. found that it is very reasonable for insects to use these transporters to excrete insecticides and produce drug resistance (Citation2017). Studies have shown that several members of the insect ABC transporter superfamily play a very important role in pesticide resistance by inhibiting the accumulation of intracellular pesticides and their metabolites. Several members of the insect ABC transporter superfamily inhibit the accumulation of intracellular pesticides and their metabolites. It plays a very important role in pesticide resistance (Buss and Callaghan Citation2008).
The resistance to EMB due to its extensive application, has been documented in different pests, such as Amrasca devastans (Argentine et al. Citation2002), Musca domestica (Abbas et al. Citation2015; Khan et al. Citation2016), Spodoptera litura (Shad et al. Citation2010; Zaka et al. Citation2014; Wang et al. Citation2018), and also S. exigua (Ishtiaq et al. Citation2014; Abbas et al. Citation2015). A field study conducted from 2008 to 2010 in three regions in Shandong province (China) revealed that S. exigua had developed varying degrees of resistance to EMB. Yet, the molecular mechanism underlying the evolution of this resistance in S. exigua against EMB remains unclear (Zhou et al. Citation2011). Therefore, in order to systematically identify the target genes in S. exigua responsible for its resistance to EMB, this study used transcriptome sequencing, bioassay, and correlation analysis to elucidate the underlying molecular pathways that are involved in the responses of S. exigua treated with different lethal doses of EMB.
Materials and methods
Tested insects
EMB has both gastric toxicity and contact killing properties as its mode of action (MoA). The detailed information about its purity (95.5% AI,) was provided by Hebei Veyong Bio-Chemical Co. Ltd. (Source) (Sun et al. Citation2016, Citation2014). A regression equation, y = 5.8037 + 1.3285x, which described the responses of the 3rd instar larvae of S. exigua to EMB toxicity was developed in our previous study. Also determined was the final 50% lethal concentration (LC50) of EMB, which was = 0.248 mg•L–1 (SE = 0.04, the 95% confidence interval was 0.18–0.35 mg•L–1, and the R-value was 0.9971). The formula given above was used to prepare different lethal doses of EMB (LC5 = 0.0144, LC20 = 0.0578, and LC50 = 0.248 mg•L–1) which were incorporated into artificial diets to feed the 3rd instar larvae of S. exigua. Acetone and tween80 solution treatments were used as solvent contrast control (AC), while water was used as a blank control (CK).
Pre-experimental results indicated that concentrations lower than LC5, had little effects and concentrations higher than LC50, produced no results for most life parameters. Therefore, the lethal concentrations, LC5, LC20, and LC50 were chosen for this study. Samples made up of the 3rd instar larvae of S. exigua of similar sizes were selected and fed on artificial diets mixed with different doses (LC5, LC20, and LC50) of EMB, AC and CK. Each treatment was replicated six times, each repeat had 30 dishes and each dish had five larvae, resulting in every treatment containing at least 900 larvae of S. exigua.
The original colony of S. exigua was provided by the Plant Protection Institute of Jiangsu Academy of Agricultural Sciences (China). S. exigua larvae were reared on an artificial diet and did not have any contact with pesticides in the climate room. The room was set at 27 ± 1°C, RH 65 ± 5% humidity and 14: 10 d/night light regime. A 10% honey water was supplied to the adults (Dai Citation2015; Zhao et al. Citation2018). After feeding on the diet mixed with EMB for 72 h, surviving insects were switched to a non-toxic fresh artificial diet. Data on larval mortality, oviposition and hatching ability were recorded following the methods described by Zhao et al. (Citation2018) and Sun et al. (Citation2016) with slight modifications. For example, within 72 h from the beginning of the experiment, individual insects which did not move when their bodies were touched repeatedly with a brush dipped in water were considered dead. Finally, we also considered insects that did not successfully pupate as dead. We defined ultimate mortality as the mortality of larvae from the beginning of treatment to the end of the 5th instar larvae, that is, the total mortality of the larvae under different concentrations of EMB. In addition, the following formula was used to calculate ‘growth inhibition rate’: [(WATCK–WBTCK)–(WATLC–WBTLC)]/(WATCK–WBTCK). (WATCK is weight after treatment under blank control; WBTCK is weight before treatment under blank control; WATLC is weight after treatment under lethal concentration treatment; WBTLC is weight before treatment under lethal concentration treatment). Data analyses were carried out with the SAS v.9.0 software (SAS Institute, Cary, NJ, USA). Data were mean ± standard deviation and the differences between means were analyzed using a one-way analysis of variance (ANOVA), followed by Tukey’s honest significant difference (HSD) test. Samples of S. exigua fed with the diet mixed with EMB for 72 h were collected for later transcriptome sequencing and quantitative RT–PCR analysis. The sample from each treatment contained at least 100 larvae of S. exigua.
RNA isolation and sequencing
Total RNA was extracted from S. exigua larvae treated with different concentrations of EMB (CK, AC, LC5, LC20, LC50), with the SV Total RNA Isolation kit (Promega, Madison, WI, USA) following the manufacturer’s instructions (Tian et al. Citation2018). Magnetic beads with Oligo (dT) were used to enrich eukaryotic mRNAs to construct the sequencing library, after which a fragmentation buffer was added to break the mRNAs into short pieces. Six random base primers were used to synthesize the first strand of cDNA using mRNA as templates; after which the double-strand cDNA was synthesized by adding buffer, dNTPs, DNA polymerase I and RNase H. The AMPure XP beads were used to select the fragment size. Finally, PCR amplification was performed, and the AMPure XP beads were used to purify the PCR product to obtain the final library.
Qubit 2.0 was used to initially quantify the constructed library and was diluted to 1.5 ng•μl–1. An Agilent 2100 bioanalyser was used to detect the insert size of the library. The Q-PCR method was used to quantify the effective concentration of the library (> 2nM) after achieving the expected insert size (Wang et al. 2019). Then, the different libraries were pooled for sequencing on the Illumina HiSeq platform, based on their effective concentration and the required raw data.
Quality control and functional annotation of unigenes
Adapters and low-quality reads were first removed using fastp (Chen et al. Citation2018). Using the clean reads, a de novo transcriptome assembly was performed using Trinity, and the longest transcripts of genes were selected as unigenes (Grabherr et al. Citation2011). The CDS sequences were generated using TransDecoder and then were aligned to protein databases, including the NCBI non-redundant protein database, Swiss-Prot database, KOG and KEGG database using Blastx with E-values < 1E-5. GO annotation of the unigenes was performed using Blast2GO and InterProScan5 software (Conesa et al. Citation2005; Jones et al. Citation2014).
Analysis of differentially expressed genes (DEGs)
For each sample, high-quality reads were mapped to unigenes using Tophat and Bowtie (Langmead et al. Citation2009; Trapnell et al. Citation2009; Kim et al. Citation2013). Cufflinks calculated the expression level (FPKM, fragment per kilobase per million reads). Differentially-expressed genes (DEGs) between groups were identified using cuffdiff with FDR (false discovery rate) <0.01 and a 2-fold change (Roberts et al. Citation2011).
GO and KEGG pathway analysis
Based on the GO and KEGG annotation results, a hypergeometric test was used to analyze the functional enrichment of DEGs. The dhyper function in the R language was used to calculate the p-value in the enrichment analysis. Also, the multiple-test corrected p-value (q-value) was calculated using the Benjamini-Hochberg function implemented in the R library. Q value <0.05 was established as the significance threshold.
Co-expression network analysis
Weighted gene co-expression network analysis (WGCNA) was used to construct a scale-free network from gene expression data (Langfelder and Horvath Citation2008). Also, a hybrid dynamic tree-cutting algorithm was used to allocate modules, which included at least 15 genes. After studying the correlation between the gene expression modules and phenotype characteristics, the correlation coefficient, and the p-value of correlation analysis were used to determine whether the module was related to the phenotype. Also, an MM (module membership) value was used to identify the hub genes in the modules. The Cytoscape software was used to visualize these networks (Killcoyne et al. Citation2009).
qRT–PCR data analysis
Total RNA of S. exigua samples treated with different concentrations of EMB (CK, AC, LC5, LC20, LC50) was extracted using the SV total RNA isolation kit (Promega, Madison, WI, USA) following the manufacturer's instructions. An MMLV Reverse Transcriptase (Promega, Madison, WI, USA) treated with ribonuclease H (TCytoscapeakara, Tokyo, Japan) was used to synthesize the cDNA library, and spectrophotometry was used to quantify the library. The primers used for real-time quantitative reverse transcription (RT)-PCR (SYBR Green I) were designed based on eight randomly-selected genes from the RNA-seqdata (Table ). Also, the S. exigua housekeeping gene, β-actin, was used as an endogenous reference for data normalization (GenBank accession no. JN616391). The primers for β-actin were 5′-CCAGCCTTCCTTCTTGGGTAT-3′ and 5′- AGGTCCTTACGGATGTCAACG-3′ (Zhao et al. Citation2018). The other 15 random sequences that were used to verify differential transcriptome sequence results in this study are listed in Table .
Table 1. Fifteen RT-Q-PCR primer sequences used to verify differential transcriptome sequencing results in this study.
Primer 5.0 software was used to design all EST-specific primers. The reactions were carried out using the SYBR Premix Ex Taq Kit (Takara, Tokyo, Japan) and were run on the Bio-rad iCycler real-time quantitative RT–PCR detection system. Melting curve analysis and gel electrophoresis were also used to confirm these genes. The reactions of each treatment were replicated four times; non-template control reactions were performed in triplicate for each primer pair. The expression levels of each gene in S. exigua under the different treatments (expressed as the relative quantification (RQ) values), were calculated using the 2−ΔΔCt method (Livak and Schmittgen Citation2001). Significant differences in gene expressions were detected with Duncan’s new multiple range method.
Results
Physiological indices of S. exigua under different treatments
After S. exigua larvae were treated with different doses of EMB, the S. exigua all showed a series of long-lasting effect phenotypes, such as non-feeding, growth retardation, slower pupal development and reduction in oviposition. Specifically, the growth inhibition rate, the final mortality rate and the mortality rate after 72 h of larvae treated with different lethal doses of EMB were all significantly higher compared to the CK and AC controls (p < 0.05). By contrast, larval weight was significantly lower than that in the control groups (p < 0.05), and no significant difference was found between the two controls (p > 0.05) (Table ). The mortality after 72 h treatment of EMB was close to the 5%, 20%, and 50% mortality rates predicted by the LC5, LC20, and LC50 respectively. However, the LC5, LC20, and LC50 values were higher than 5%, 20%, and 50% of final mortality rates recorded respectively. This indicated that the EMB treatment had a sustained lethal effect on the S. exigua larvae; specifically, an inhibitory effect on the development and survival of the S. exigua larvae.
Table 2. Effects of different lethal doses of EMB on key life-history parameters of S. exigua larvae.
The life span of S. exigua significantly increased (p < 0.05) following an increase in lethal doses (LC5 to LC50) compared to the CK and AC controls, while the eclosion rate of S. exigua significantly decreased (p < 0.05) (Table ). Similar downward trends were recorded for egg hatching rates, intrinsic rate of increase, per female fecundity following increases in lethal doses (LC5 to LC50). The intrinsic rate of increase and per female fecundity showed significant decreases after treatment with different lethal doses of EMB (p < 0.05) compared to the controls (Table ). There were no significant differences in egg hatching rate, larvae and total periods between the S. exigua treated with EMB at LC5 dose and two controls (p > 0.05). However, these were significantly lower (egg hatching rate) or higher (larvae and total periods) in S. exigua treated with EMB at LC20 and LC50 doses than that in two controls or S. exigua treated with EMB at LC5 dose (p < 0.05). In addition, there was no significant difference in all of the key life-span parameters between the blank (CK) and solvent (AC) controls (p > 0.05) (Tables and ). These results indicated that key life parameters, such as larval final mortality rate, per female fecundity and intrinsic growth rate in S. exigua were affected by EMB stress in a dose-dependent manner; and different lethal doses of EMB could long-lasting inhibited the development and survival of the S. exigua larvae, and significantly reduced per female fecundity and intrinsic growth rate of S. exigua (p < 0.05), therefore effectively inhibited the proliferation of S. exigua population.
Table 3. Effects of different lethal doses of EMB on key life-history parameters of S. exigua.
De novo assembly of transcriptome and functional annotation of unigenes
The Illumina HiSeqTM 4000 sequencing platform was used to construct transcriptome sequence containing 15 cDNA libraries. Consequently, a total of 90 Gb of 150-bp paired raw reads were generated in this study. De novo transcriptome assembly was performed using trinity which generated 67,123 unigenes with an N50 value of 1941 bp.
A total of 26,358 unigenes were annotated in at least one public database, such as NR, NT, COG, GO, KEGG, Swissport, and Interpro protein database (Additional file 1). Out of this total, 16,654 unigenes were annotated to gene ontology terms. These unigenes were found in 49 functional groups: 23 groups in the ‘biological process’, 16 groups in the ‘cellular component’, and ten groups in the ‘molecular function’. In addition, the top five species from which the most BLAST hits were obtained included Bombyx mori, Danaus plexippus, Microtus ochrogaster, Helicoverpa armigera, and Tribolium castaneum (Figure (A)).
In the KEGG database, a total of 8591 unigenes were assigned to 32 functional categories; the largest category was signal transduction (971 unigenes), followed by carbohydrate metabolism (685 unigenes), translation (654 unigenes), and then other pathways (Figure (B)).
Differential expression and GO enrichment analysis
To determine the expression of S. exigua unigenes at different EMB treatments, the clean RNA-seq reads were mapped to the 67,123 unigenes. Gene expression levels were calculated as FPKM values. Finally, we found that 44,113 of 67,123 expressed in at least 12 samples. In this study, differential gene expression analysis was conducted between the different EMB treatments and the control (AC and CK) with fold change levels ≥2 with a FDR ≤0.05. Removal of the DEGs between the water (CK) and the solvent controls (AC) resulted in the identification of 3517 DEGs between AC and LC5. Of these 1,622 unigenes were down-regulated, while 1895 genes were up-regulated under LC5 treatment (Additional file 7). In addition, 3139 DEGs were identified between the AC and LC20. Of these, 1644 genes were down-regulated, while 1495 were up-regulated following the LC20 treatment (Additional file 8). Also, 5135 unique DEGs were found between the AC and LC50 treatment. Out of these 2351 were down-regulated, while 2784 were up-regulated following the LC50 treatment (Figure (A), Additional file 9). More importantly, the number of DEGs was the highest in samples treated with the LC50 of EMB.
Figure 2. (A) the up-regulated genes compared to down-regulated genes in LC after removal of the differentially-expressed genes between AC and CK (B) The enrichment GO terms of DEGs.
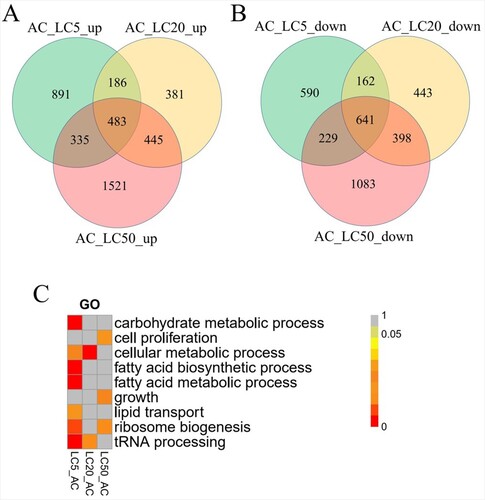
To understand the functions of the DEGs, the GO terms of DEGs were performed by GO enrichment analysis. As shown in Figure (C), ‘growth’ (GO: 0040007) was enriched between LC50 and AC. This indicated that the growth of S. exigua was inhibited by the EMB treatment. We also found that the EMB treatment can cause concentration-specific gene expression patterns. GO enrichment analysis results indicated that the DEGs were also related to ‘carbohydrate metabolic process’ (GO: 0005975), ‘cell proliferation’ (GO: 0008283), and ‘cellular metabolic process’ (GO: 0044237), but these GO terms were enriched in different EMB treatment concentrations.
Devlopment and immunity-related pathway analysis by KEGG
KEGG pathway enrichment analyses was carried out to understand the functions of DEGs. A total of 21 KEGG pathways were enriched in the DEGs between LC5 and AC. One of these was the peroxisome pathway which is related to insect development and immunity. It is a remarkbale fact that KEGG pathways of ‘apoptosis’, ‘ABC transporters’, ‘RNA degradation’, ‘olfactory transduction’, ‘folate biosynthesis’ and ‘lysosome pathways’ were significantly enriched in DEGs between LC20 and AC. These pathways are responsible for insect development, reproduction and immunity. As the Figure shows, the Lysosomal-associated membrane protein 1 (LAMP-1) gene was up-regulated under EMB treatement and three cathepsins genes were up-regulated under EMB treatement. Also, 17 pathways which including the ribosome, folate biosynthesis, and phagosome pathways were enriched in the DEGs between LC50 and AC. These are related to insect development and immunity. These results indicated that the regulation of the expression of these genes involved in insect development and immunity could be negatively affected by lethal concentrations of EMB.
Figure 3. Lysosome pathway and the expression of the related DEGs. In the heatmaps, there are five samples in each row, which represent the expression level of the gene in the five samples of AC, CK, LC5, LC20, LC50. Red represents a high level of expression, while green represents a low level of expression.
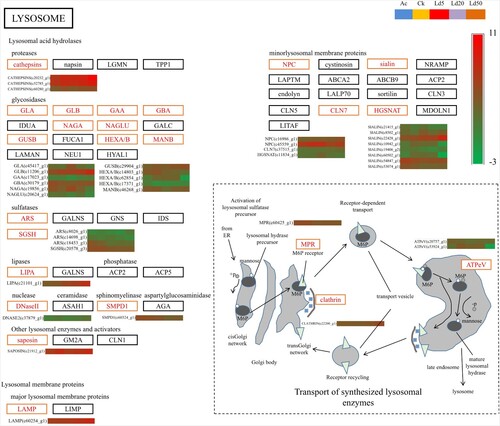
Effects of gene expression levels on ABC transporters and insect olfaction
KEGG pathway analysis of the transcriptome data showed that the ABC transporter was an important gene family involved in the response of the S. exigua to different lethal stress of EMB (Figure ). The ABC transporter-related genes c15483_g1(ABCB4, ATP-binding cassette subfamily B member 4), c15483_g2(ABCB1, ATP-binding cassette subfamily B member 1) and c17259_g2(ABCA3, ATP-binding cassette subfamily A member 3), were highly expressed under LC20 and LC50 treatment (p < 0.05), and only c1698_g1 and c22475_g1 were highly expressed under LC50 treatment (p < 0.05).
Figure 4. ABC transporter pathway and the expression of the related DEGs. In the heatmaps, there are five samples in each row, which represent the expression level of the gene in the five samples of AC, CK, LC5, LC20, LC50. Red represents a high level of expression, while green represents a low level of expression.
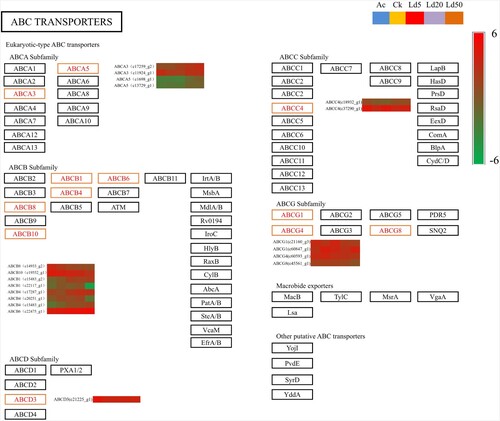
Olfaction has an important role in many aspects of insect behavior, such as foraging. The analyses revealed several DEGs involved in the olfactory transduction pathway under EMB treatment (Additional file 7-9). One of such was the cAMP-dependent protein kinase catalytic subunit-like gene that was up-regulated in the S. exigua larvae treated with EMB. While the cGMP-dependent protein kinase G gene was down-regulated under EMB treatment. Also, the calmodulin gene, c21765_g1, in the olfactory transduction pathway and cAMP-dependent protein kinase catalytic subunit gene, c20528_g1, were all highly expressed under LC50 treatment (p < 0.05), and only the calmodulin gene, c4625_g2 was highly expressed under LC5 treatment (p < 0.05).
Weighted correlation network analysis and identification of hub genes
Furthermore, we performed weighted gene co-expression network analysis (WGCNA) to explore the dynamic patterns of S. exigua gene expression and identify genes associated with complex phenotypes. WGCNA divided the DEGs into 42 different modules that depicted by a branch of different colors (Figure (F)). Association of the detected modules and the physiological indices showed that five modules (orange, blue, royal blue, skyblue3 and light yellow), containing 668 unigenes, exhibited a strong correlation between gene expression and some key life-history parameters (|correlation coefficient| > 0.6, p < 0.01), such as growth inhibition rate, larval period, mortality after 72 h treatment, ultimate mortality, life-span, adult emergence rate, per female fecundity, hatching rate of eggs and intrinsic rate of increase. These suggested that these DEGs played an important role in S. exigua when in response to EMB stress.
Figure 5. (A–E) Network of genes in the blue, yellowlight, skyblue3, orange and royalblue modules. (F) Association of modules with key life-history parameters. Forty-two modules were identified by WGCNA analysis. For each module, a module eigengenes (ME) was selected as representative of the module gene expression. The correlation coefficients and p values were marked on the diagram.
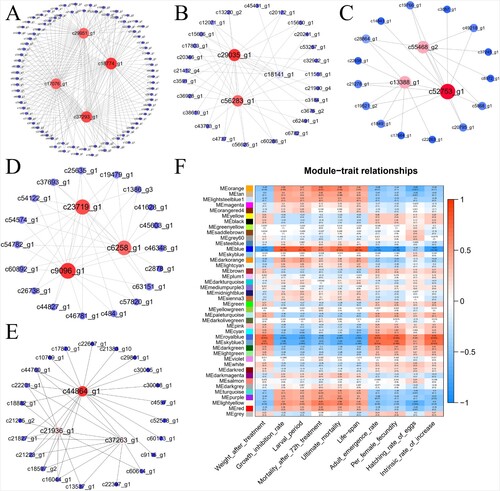
Hub genes, defined as genes which are mostly connected to other genes in the modules, are more important than other genes in the cluster. According to this principle, four, three, three, three, and three hub genes in orange, blue, royal blue, skyblue3, and lightyellow modules were identified, respectively (Figure (A–E), Additional file 2-6). For further elucidation of the functional properties of the genes in important modules, KEGG pathway enrichment analysis was also conducted (Figure ). The results indicated that genes in the orange module were enriched in protein digestion and absorption and carbohydrate digestion and absorption pathways, which indicated that the food digestion and absorption of S. exigua were affected by EMB treatment. The yellow light module was positively related to the mortality after 72 h treatment and negatively correlated with the hatching rate of eggs. The genes in lightyellow module were enriched in the mRNA surveillance pathway, fat digestion, absorption pathway and drug metabolism – cytochrome P450. This module included two P450 metabolic-related unigenes, which may played an important role in the regulation of detoxification function. Unigene c13436_g1 was significantly down-regulated under LC20 and LC50 treatment (p < 0.05), and unigene c21930_g4 was significantly up-regulated under LC5 treatment (p < 0.05). In addition, the blue module, which was significantly correlated with seven key life-history parameters, was mainly enriched in pathways apoptosis, toll-like receptor signaling pathway, and DNA replication.
qRT-Data analysis
A total of eight genes were selected according to their significance of differentially expression to confirm the gene expression data generated from the quantitative RT–PCR results. They showed that the c10037 was responsible for one kind of anti-peronosporomycetal agent synthesis; the c10172 and c10095 genes were responsible for 4-hydroxyproline betaine 2-epimerase (hpdD) and norathyriol-6-O-beta-D-glucoside (phytochemical compounds, br08003, https://www.genome.jp/kegg/kegg2.html) synthesis; the c10291 and c10070 genes had critical roles in alizarin 2-methyl ether (br08003) and isogentisin (br08003) synthesis; the c10160 and c10310 genes had important roles in myosmine (br08003) and chimaphilin (br08003) synthesis; the c10441 gene was responsible for 4-p-coumaroylquinic acid synthesis. The transcriptional changes in these eight genes between RNA-seq and RT-qPCR are described in Figure (a–h). A significant correlation (R2 = 0.79) was also observed between the results of RT-qPCR and the RNA-seq analysis (Figure (i)), indicating the reliability of the RNA-seq data results.
Discussion
EMB is a highly efficient pesticide that is commonly used for pest control in the field (López et al. Citation2010; Moustafa et al. Citation2016). However, S. exigua has begun to develop resistance to EMB. A previous study revealed no significant difference in the key life parameters (such as spawning rate or larvae mortality) between a conventional strain with EMB resistance and a normal strain (p > 0.05) (Che et al. Citation2015). Simultaneously, the D value calculated from LC50 of the two-parent strains and their F1 progeny was 0.6, which confirmed incomplete dominance of resistance to EMB in the resistance strain. It also indicated the return of the physiological indices to normal levels, even when the resistance of S. exigua against EMB appeared (Che et al. Citation2015). However, in this study, treatment with different lethal doses of EMB resulted in a significantly higher larval mortality and a prolonged larval stage (ultimate mortality of larvae stage) (p < 0.05). They also had a significant negative effect on adult parameters, such as female fecundity, adult emergence rate and egg hatching rates (p < 0.05). These showed that EMB had a great long-lasting control effect on conventional stains of S. exigua. However, the resistant strains of S. exigua may have evolved physiological or molecular mechanisms to overcome the toxicity of EMB (Che et al. Citation2015).
Similar to our results, other reports also indicated that EMB had a significant impact on the growth and spawning of pests. For example, Muhammad et al. (Citation2018) reported a significant negative effect on the development, feeding potential and body weight in Paederus fuscipes treated with LC30 of EMB. Also, treatment of Spodoptera littoralis with EMB resulted in a significant reduction in body weight and decreased survival rate (ElSheikh Citation2015). Further, treatment of Mamestra brassicae with different doses of EMB resulted in prolonged development, sustained mortality, and decreased egg production (Moustafa et al. Citation2016). The above results indicated that pests treated with EMB showed similar inhibitory phenotypes in growth, development, and population reproduction. In addition to EMB, another study showed that the effect of inhibitory phenotypes in growth, development, or population reproduction of S. exigua was also shown after its larvae treated with Heliothis virescens ascovirus 3 h (HvAV-3 h) (Yu et al. Citation2019, Citation2018) or cadmium stress (Su et al. Citation2020). However, expressed genes of S. exigua larvae treated with EMB, HvAV-3 h or cadmium stress presented a huge difference at the transcriptome level, indicating that when coping with different stresses, even if the similar phenotypes may be shown in S. exigua, different corresponding mechanism at the molecular level may be existed.
In order to further and more accurately discover the primary cues involved in resistance to EMB, we used entire life history parameters to elucidate the physiological phenotype of S. exigua under different lethal stresses of EMB. A previous study reported by Che et al. Citation2015 identified these cues by only using resistance strains (Che et al. Citation2015). Compared with our study, the inhibitory phenotypes in growth, development, and population reproduction were not shown in the results using EMB resistant population of S. exigua by Che et al. Citation2015, indicating that some molecular mechanism must be regulated to help S. exigua defend against the initial stress of EMB, so as to restore its normal phenotype. Therefore, in this study, gene expression networks of S. exigua larvae treated with three different lethal concentrations of EMB was evaluated. Our results revealed that a series of important metabolic pathways (such as ABC transporters, olfactory recognition, and metabolic detoxification) may be involved in the response of the S. exigua against EMB stress and may further participate in the later evolution of resistance.
Previous studies indicated that ABC transporters play an important role in pests resistance to pesticides and other toxic compounds (Epis et al. Citation2014; Pignatelli et al. Citation2018; Zuo et al. Citation2018). Specifically, some studies have reported that ABC transporter genes were up-regulated in mosquito larvae after insecticide exposure (Figueira-Mansur et al. Citation2013; Mastrantonio et al. Citation2017). Also, the expression of ABCC, which belongs to ABC subfamilies, was increased in Nilaparvata lugens nymphs treated with sub-lethal concentrations of triazophos (Bao et al. Citation2010). In addition, another study also reported that the ABC transporter gene (ABCG3) was involved in imidacloprid detoxification in Q-type Bemisia tabaci (He et al. Citation2019). Also, ABC transporter genes were the important genes for S. exigua defense against insecticidal protein, such as 11 ABC transporter unigenes were reported up-regulated in Cry1Ca-treated S. exigua (Ren et al. Citation2020). In our study, KEGG pathway analysis of transcriptome data showed that the ABC transporter was also an important gene family involved in the response of the S. exigua to the different lethal stress of EMB. ABC transporter genes such as c15483_g1, c15483_g2 and c17259_g2 were all highly expressed under LC5, LC20 and LC50 treatment. By contrast, c13729_g1 and c17287_g1 were highly expressed under LC20 and LC50 treatment and only c1698_g1(ABCA5) and c22475_g1 (ABCB6) were highly expressed under LC50 treatment. Thus, these seven ABC transporter genes are very likely to have an important role in the evolution of resistance to EMB in the S. exigua and should be further studied.
The insect olfactory system plays an important role in directing insects towards safe food, away from toxic compounds, and locating mating partners or oviposition sites (Sato and Touhara Citation2009; Leal Citation2013; Pelosi et al. Citation2018; Yang et al. Citation2020). Silencing of the odorant receptor co-receptor (BmOrco) gene was in Bombyx mori, resulted in anti-feeding behavior and reduction in mate selection ability (Liu et al. Citation2017). After the olfactory-related gene Orco was disrupted, olfactory responses in both adults and larvae of Anopheles coluzzii were severely disrupted, especially for olfactory-related behaviors, such as host-seeking or oviposition in adult females (Sun et al. Citation2020). Further, current studies have shown that the key proteins and genes of olfactory systems have also become important molecular response targets to insecticide stress (Sato and Touhara Citation2009; Leal Citation2013; Pelosi et al. Citation2018; Yang et al. Citation2020). For example, Athetis lepigone responded to pesticides with general-odorant binding proteins (GOBPs). Previous ligand binding assays demonstrated that AlepGOBP2 in A. lepigone showed high bindings with sex pheromones, maize plant volatiles and insecticides frequently used in the field (Zhang et al. 2020). Furthermore, a study of GOBPs in Plutella xylostella indicated that they might be involved in guiding larvae to their pheromones for foraging better food (Zhu et al. Citation2016). In addition, two A. lepigone pheromone-binding proteins AlepPBP2 and AlepPBP3 had higher binding affinities to phoxim than to other insecticides, which indicated that they play crucial roles in the AlepPBPs/phoxim interactions (Zhang et al. 2020).
Many genes in S. exigua, such as odorant receptors (ORs), odorant binding proteins (OBPs) involved in olfactory recognition had also been identified in adults (Du et al. Citation2018). However, the information about olfactory recognition related gene in S. exigua larvae was still insufficient, especially the olfactory recognition related genes in response to the pesticide stress. In view of larval stage is the main chemical control period of S. exigua, identifying genes related to the special olfactory recognition of the larvae in response to pesticides was the key way to clear the resistance evolution of S. exigua against pesticides (Zhang et al. Citation2012; Jin et al. Citation2015; Xiong et al. Citation2019). In our study, the S. exigua treated with EMB, showed series of physiological phenotypes such as reduced egg-laying rate, refusal to feed, and growth retardation, which could be influenced by changes in the expression of olfactory genes. The calmodulin gene c21765_g1 in the olfactory transduction pathway and cAMP-dependent protein kinase catalytic subunit gene c20528_g1, were highly expressed under LC50 treatment. By contrast, the calmodulin gene c4625_g2 was highly expressed under LC5 treatment. These three olfactory genes may have important roles in the normal behavior of S. exigua, such as mating, oviposition, feeding and escaping from toxic compounds, and consequently may be special molecular targets in S. exigua against EMB stress.
Detoxification enzymes are involved in the evolution of pesticide resistance (Ketterman et al. Citation2011; Tang et al. Citation2014) . Insects usually use P450 detoxification enzymes to metabolize pesticides in their bodies (Yang et al. Citation2013; Scanlan et al. Citation2020; Wu et al. Citation2020). In the present study, we explored two genes involved in the P450 metabolic pathway by transcriptome sequencing. The results showed that, the gene, c13436_g1, was significantly down-regulated under LC20 and LC50 treatment, while gene c21930_g4 was significantly up-regulated under LC5 treatment compared to AC samples. The significant decrease in expression of gene c13436_g1 reduced the expression of related genes in the P450 metabolic pathway. This may have resulted in the malfunctioning of this pathway which may have resulted in the death of S. exigua larvae. Moreover, the significant up-regulation of gene c21930_g4 under LC5 treatment indicated that under low stress, a significant increase in the expression of some detoxification enzymes could overload the expression of the metabolic detoxification pathways to achieve detoxification. However, high selection pressures under LC20 and LC50 could cause this detoxification enzyme system to exceed its range, to cause a decreased expression of this gene under these two treatments.
In conclusion, the present study showed that key life parameters, such as larval final mortality rate, per female fecundity and intrinsic growth rate in S. exigua were affected by EMB stress in a dose-dependent manner. Different lethal doses of EMB (LC5, LC20, and LC50) could all effectively inhibited the proliferation of S. exigua population by long-lasting inhibition the survival of the S. exigua larvae, and significantly reduction of per female fecundity and intrinsic growth rate of S. exigua (p < 0.05). In addition, this study also highlighted many genes responsible for EMB resistance evolution by differential transcriptome analysis. Subsequently, genes involved in the key metabolic pathways were explored, and 16 hub genes were identified as biomarkers (Additional file 10). These results help to elucidate the mechanisms underlying the resistance of S. exigua against EMB and are very significant for a comprehensive understanding of the evolution of this resistance.
Supplemental Material
Download Zip (10.3 MB)Authors’ contributions
YS, HYD, LXB, YJZ, and XDK conceived and designed the experiments. YS, WZ, BJ, and SMT contributed to paper writing. YS, JZ, SMT, BSL, and HYD conducted the experiment. YS, SMT, and XDK contributed to the data analysis.
Availability of data and materials
The data are presented in the manuscript and the supporting materials. The raw reads data are submitted to the Short Read Archive (SRA) and BioProject accession number PRJNA523075 (https://www.ncbi.nlm.nih.gov/bioproject/PRJNA523075).
Disclosure statement
No potential conflict of interest was reported by the author(s).
Additional information
Funding
References
- Abbas N, AliShad S, Ismail M. 2015. Resistance to conventional and new insecticides in house flies (Diptera: Muscidae) from poultry facilities in Punjab, Pakistan. J Econ Entomol. 108:826–833. doi:10.1093/jee/tou057.
- Akter MS, Siddique SS, Momotaz R, Arifunnahar M, Mohiuddin SJ. 2019. Biological control of insect pests of agricultural crops through habitat management was discussed. J Agr Chem Environ. 8:1–13. doi:10.4236/jacen.2019.81001.
- Argentine JA, Jansson RK, Halliday WR, Rugg D, Jany CS. 2002. Potency, spectrum and residual activity of four new insecticides under glass house conditions. Flo Entomol. 85:552–562. doi:10.1653/0015-4040(2002)085[0552:PSARAO]2.0.CO;2.
- Bao YY, Li BL, Liu ZB, Xue J, Zhu ZR, Cheng JA, Zhang CX. 2010. Triazophos up-regulated gene expression in the female brown planthopper, Nilaparvata lugens. J Insect Physiol. 56:1087–1094. doi:10.1016/j.jinsphys.2010.03.004.
- Buss DS, Callaghan A. 2008. Interaction of pesticides with p-glycoprotein and other ABC proteins: a survey of the possible importance to insecticide, herbicide and fungicide resistance. Pestic Biochem Phy. 90(3):141153. doi:10.1016/j.pestbp.2007.12.001.
- Che W, Huang J, Guan F, Wu Y, Yang Y. 2015. Cross-resistance and inheritance of resistance to Emamectin benzoate in Spodoptera exigua (Lepidoptera: Noctuidae). J Econ Entomol. 108:2015–2020. doi:10.1093/jee/tov168.
- Chen S, Zhou Y, Chen Y, Gu J. 2018. Fastp: an ultra-fast all-in-one FASTQ preprocessor. Bioinformatics. 34:884–890. doi:10.1101/274100.
- Conesa A, Götz S, García-Gómez JM, Terol J, Talón M, Robles M. 2005. Blast2GO: a universal tool for annotation, visualization and analysis in functional genomics research. Bioinformatics. 21:3674–3676. doi:10.1093/bioinformatics/bti610.
- Dai HY. 2015. The adaptation mechanism of Spodoptera exigua to emamectin benzoate stress with different sublethal dose. [Master Thesis]. Nanjing Agricultural University.
- Du LX, Liu Y, Zhang J, Gao XW, Wang B, Wang GR. 2018. Identification and characterization of chemosensory genes in the antennal T transcriptome of Spodoptera exigua. Comp Biochem Phys D. 27:54–65. doi:10.1016/j.cbd.2018.05.001.
- ElSheikh ESA. 2015. Comparative toxicity and sublethal effects of emamectin benzoate, lufenuron and spinosad on Spodoptera littoralis Boisd. (Lepidoptera: Noctuidae). Crop Prot. 67:228–234. doi:10.1016/j.cropro.2014.10.022.
- Epis S, Porretta D, Mastrantonio V, Urbanelli S, Sassera D, DeMarco L, Mereghetti V, Montagna M, Ricci I, Favia G. 2014. Temporal dynamics of the ABC transporter response to insecticide treatment: insights from the malaria vector Anopheles stephensi. Sci Rep-UK. 4:7435. doi:10.1038/srep07435.
- Figueira-Mansur J, Ferreira-Pereira A, Mansur JF, Franco TA, Alvarenga ES, Sorgine MH, Neves BC, Melo AC, Leal WS, Masuda H. 2013. Silencing of P-glycoprotein increases mortality in temephos-treated Aedes aegypti larvae. Insect Mol Biol. 22:648–658. doi:10.1111/imb.12052.
- Gott RC, Kunkel GR, Zobel ES, Lovett BR, Hawthorne DJ. 2017. Implicating ABC transporters in insecticide resistance: research strategies and a decision framework. J Econ Entom. 110(2):667–677. doi:10.1093/jee/tox041.
- Grabherr MG, Haas BJ, Yassour M, Levin JZ, Thompson DA, Amit I, Adiconis X, Fan L, Raychowdhury R, Zeng Q. 2011. Full-length transcriptome assembly from RNA-Seq data without a reference genome. Nat Biotechnol. 29:644–652. doi:10.1038/nbt.1883.
- Hafeez M, Liu S, Yousaf HK, Jan S, Wang RL, Fernández-Grandon GM, Li X, Gulzar A, Ali B, Rehman M. 2020. RNA interference-mediated knockdown of a cytochrome P450 gene enhanced the toxicity of α-cypermethrin in xanthotoxin-fed larvae of Spodoptera exigua (Hübner). Pestic Biochem Phys. 162:6–14. doi:10.1016/j.pestbp.2019.07.003.
- Hafeez M, Ullah F, Khan MM, Li XW, Zhang ZJ, Shah S, Imran MA, Assiri MG, Mandela FG, Desneux N, et al. 2021. Metabolic-based insecticide resistance mechanism and ecofriendly approaches for controlling of beet armyworm Spodoptera exigua: a review. Environ Sci Pollut Res. 10:1–17. doi:10.1007/s11356-021-16974-w.
- Hartman JB, Clair DAS. 1999. Combining ability for beet armyworm, Spodoptera exigua, resistance and horticultural traits of selected Lycopersicon pennellii-derived inbred backcross lines of tomato. Plant Breed. 118:523–530. doi:10.1046/j.1439-0523.1999.00437.x.
- He C, Liang J, Liu S, Wang S, Wu Q, Xie W, Zhang Y. 2019. Changes in the expression of four ABC transporter genes in response to imidacloprid in Bemisia tabaci Q (Hemiptera: Aleyrodidae). Pestic Biochem Phys. 153:136–143. doi:10.1016/j.pestbp.2018.11.014.
- Huang JM, Zhao YX, Sun H, Ni H, Liu C, Wang X, Gao CF, Wu SF. 2021. Monitoring and mechanisms of insecticide resistance in Spodoptera exigua (Lepidoptera: Noctuidae), with special reference to diamides. Pestic Biochem Phys. 174:104831. doi:10.1016/j.pestbp.2021.104831.
- IRAC. 2019. IRAC mode of action classification scheme. Version 9.3. https://www.irac-online.org/modes-of-action; p. 1–26.
- Ishaaya I, Kontsedalov S, Horowitz AR. 2002. Emamectin, a novel insecticide for controlling field crop pests. Pest Manag Sci. 58:1091–1095. doi:10.1002/ps.535.
- Ishtiaq M, Razaq M, Saleem MA, Anjum F, Noorul AM, Raza AM, Wright DJ. 2014. Stability, cross-resistance and fitness costs of resistance to emamectin benzoate in a re-selected field population of the beet armyworm, Spodoptera exigua (Lepidoptera: Noctuidae). Crop Prot. 65:227–231. doi:10.1016/j.cropro.2014.08.007.
- Jin R, Liu NY, Liu Y, Dong SL. 2015. A larval specific OBP able to bind the major female sex pheromone component in Spodoptera exigua (Hübner). J Integr Agr. 14:1356–1366. doi:10.1016/S2095-3119(14)60849-2.
- Jones P, Binns D, Chang HY, Fraser MW, Anulla M. 2014. Interproscan 5: genome-scale protein function classification. Bioinformatics. 30:1236–1240. doi:10.1093/bioinformatics/btu031.
- Ketterman AJ, Saisawang C, Wongsantichon J. 2011. Insect glutathione transferases. Drug Metab Rev. 43:253–265. doi:10.3109/03602532.2011.552911.
- Khan HA, Akram W, Khan T, Haider MS, Iqbal N, Zubair M. 2016. Risk assessment, cross-resistance potential, and biochemical mechanism of resistance to emamectin benzoate in a field strain of house fly (Musca domestica Linnaeus). Chemosphere. 151:133–137. doi:10.1016/j.chemosphere.2016.02.077.
- Killcoyne S, Carter G, Smith J, Boyle J. 2009. Cytoscape: A community-based framework for network modeling. Methods Mol Biol (Clifton, N.J.). 563:219–239. doi:10.1007/978-1-60761-175-2_12.
- Kim D, Pertea G, Trapnell C, Pimentel H, Kelley R, Salzberg SL. 2013. Tophat2: accurate alignment of transcriptomes in the presence of insertions, deletions and gene fusions. Genome Biol. 14:R36. doi:10.1186/gb-2013-14-4-r36.
- Lai T, Li J, Su J. 2011. Monitoring of beet armyworm Spodoptera exigua (Lepidoptera: Noctuidae) resistance to chlorantraniliprole in China. Pestic Biochem Phys. 101:198–205. doi:10.1016/j.pestbp.2011.09.006.
- Langfelder P, Horvath S. 2008. WGCNA: an R package for weighted correlation network analysis. BMC Bioinformatics. 9:559. doi:10.1186/1471-2105-9-559.
- Langmead B, Trapnell C, Pop M, Salzberg SL. 2009. Ultrafast and memory-efficient alignment of short DNA sequences to the human genome. Genome Biol. 10:R25. doi:10.1186/gb-2009-10-3-r25.
- Leal WS. 2013. Odorant reception in insects: roles of receptors, binding proteins, and degrading enzymes. Annu Rev Entomol. 58:373–391. doi:10.1146/annurev-ento-120811-153635.
- Liburd OE, Funderburk JE, Olson SM. 2000. Effect of biological and chemical insecticides on Spodoptera species (Lep. Noctuidae) and marketable yields of tomatoes. J Appl Entomol. 124:19–25. doi:10.1046/j.1439-0418.2000.00418.x.
- Liu Q, Liu W, Zeng B, Wang G, Hao D, Huang Y. 2017. Deletion of the Bombyx mori odorant receptor co-receptor (BmOrco) impairs olfactory sensitivity in silkworms. Insect Biochem Molec. 86:58–67. doi:10.1016/j.ibmb.2017.05.007.
- Livak KJ, Schmittgen TD. 2001. Analysis of relative gene expression data using real-time quantitative PCR and the 2−ΔΔCT method. Methods. 25:402–408. doi:10.1006/meth.2001.1262.0.
- López JD, Latheef MA, Hoffmann WC. 2010. Effect of emamectin benzoate on mortality, proboscis extension, gustation and reproduction of the corn earworm, Helicoverpa zea. J Insect Sci. 10:1–16. doi:10.1673/031.010.8901.
- Mastrantonio V, Ferrari M, Epis S, Negri A, Scuccimarra G, Montagna M, Favia G, Porretta D, Urbanelli S, Bandi C. 2017. Gene expression modulation of ABC transporter genes in response to permethrin in adults of the mosquito malaria vector Anopheles stephensi. Acta Trop. 171:37–43. doi:10.1016/j.actatropica.2017.03.012.
- Mokbel ES, Huesien A. 2020. Sublethal effects of emamectin benzoate on life table parameters of the cotton leafworm, Spodoptera littoralis (Boisd.). Bull Nat Res Cen. 44(1):1–8. doi:10.1186/s42269-020-00412-x.
- Moulton JK, Pepper DA, Jansson RK, Dennehy TJ. 2002. Pro-active management of beet armyworm (Lepidoptera: Noctuidae) resistance to tebufenozide and methoxyfenozide: baseline monitoring, risk assessment, and isolation of resistance. J Econ Entomol. 95:414–424. doi:10.1603/0022-0493-95.2.414.
- Moustafa MAM, Kákai Á, Awad M, Fónagy A. 2016. Sublethal effects of spinosad and emamectin benzoate on larval development and reproductive activities of the cabbage moth, Mamestra brassicae L. (Lepidoptera: Noctuidae). Crop Prot. 90:197–204. doi:10.1016/j.cropro.2016.09.004.
- Muhammad M K, Muhammad N, Hua H X, Cai W L, Zhao J. 2018. Lethal and sublethal effects of emamectin benzoate on the rove beetle, Paederus fuscipes, a non-target predator of rice brown planthopper. Nilaparvata lugens. Ecotoxicology and Environmental Safety. 165:19–24. doi:10.1016/j.ecoenv.2018.08.047.
- Mushtaq M, Syintsakos LR, Krieter PA, Colletti A, Wislocki PG. 1996. Absorption, tissue distribution, excretion, and metabolism of 3H- and 14C-labeled emamectin benzoate in rats. J Agr Food Chem. 44:3342–3349. doi:10.1021/jf960034j.
- Pelosi P, Iovinella I, Zhu J, Wang G, Dani FR. 2018. Beyond chemoreception: diverse tasks of soluble olfactory proteins in insects. Biol Rev. 93:184–200. doi:10.1111/brv.12339.
- Pignatelli P, Ingham VA, Balabanidou V, Vontas J, Lycett G, Ranson H. 2018. The Anopheles gambiae ATP-binding cassette transporter family: phylogenetic analysis and tissue localization provide clues on function and role in insecticide resistance. Insect Mol Biol. 27:110–122. doi:10.1111/imb.12351.
- Ren XL, Wang YY, Ma YJ, Jiang WL, Ma XY, Hu HY, Wang D, Ma Y. 2020. Midgut de novo transcriptome analysis and gene expression profiling of Spodoptera exigua larvae exposed with sublethal concentrations of Cry1Ca protein. 3 Biotech. 10(3):138. doi:10.1007/s13205-020-2129-2.
- Roberts A, Pimentel H, Trapnell C, Pachter L. 2011. Identification of novel transcripts in annotated genomes using RNA-Seq. Bioinformatics. 27:2325–2329. doi:10.1093/bioinformatics/btr355.
- Sato K, Touhara K. 2009. Insect olfaction: receptors, signal transduction, and behavior. Results Prob Cell Differ. 47:121–138. doi:10.1007/400_2008_10.
- Scanlan JL, Gledhill-Smith RS, Battlay P, Robin C. 2020. Genomic and transcriptomic analyses in Drosophila suggest that the ecdysteroid kinase-like (EcKL) gene family encodes the ‘detoxification-by-phosphorylation’ enzymes of insects. Insect Biochem Molec. 123:103429. doi:10.1016/j.ibmb.2020.103429.
- Shad SA, Sayyed AH, Saleem MA. 2010. Cross-resistance, mode of inheritance and stability of resistance to emamectin in Spodoptera litura (Lepidoptera: Noctuidae). Pest Manag Sci. 66:839–846. doi:10.1002/ps.1950.
- Su HH, Yang Y, Zou JC, Cheng YQ, Yang Y, Wu JJ, Pollak P, Yang YZ. 2020. Transcriptome analysis of the ovary of beet armyworm Spodoptera exigua under different exposures of cadmium stress. Chemophere. 251:126372. doi:10.1016/j.chemosphere.2020.126372.
- Sun H, Liu F, Ye Z, Baker A, Zwiebel LJ. 2020. Mutagenesis of the orco odorant receptor co-receptor impairs olfactory function in the malaria vector Anopheles coluzzii. Insect Biochem Molec. 127:103497. doi:10.1016/j.ibmb.2020.103497.
- Sun Y, Sheng Y, Bai L, Zhang Y, Xiao Y, Xiao L, Tan Y, Shen Y. 2014. Characterizing heat shock protein 90 gene of Apolygus lucorum (Meyer-Dür) and its expression in response to different temperature and pesticide stresses. Cell Stress Chaperon. 19:725–739. doi:10.1007/s12192-014-0500-0.
- Sun Y, Zhao J, Sheng Y, Xiao YF, Zhang YJ, Bai LX, Tan Y, Xiao LB, Xu GC. 2016. Identification of heat shock cognate protein 70 gene (Alhsc70) of Apolygus lucorum and its expression in response to different temperature and pesticide stresses. Insect Sci. 23:37–49. doi:10.1111/1744-7917.12193.
- Tang XK, Zhou XM, Wu J, Li JB, Bai LY. 2014. A novel function of sanshools: the alleviation of injury from metolachlor in rice seedlings. Pestic Biochem Phys. 110:44–49. doi:10.1016/j.pestbp.2014.02.006.
- Tian X, Liu J, Guo Z, Hu B, Kibe MD, Wang S, Wei Q, Su J. 2018. The characteristics of voltage-gated sodium channel and the association with lambda cyhalothrin resistance in Spodoptera exigua. J Asia-Pac Entomol. 21:1020–1027. doi:10.1016/j.aspen.2018.07.013.
- Trapnell C, Pachter L, Salzberg SL. 2009. Tophat: discovering splice junctions with RNA-Seq. Bioinformatics. 25:1105–1111. doi:10.1093/bioinformatics/btp120.
- Wang W, Wang J, Wei Q, Wei Q, Li B, Zhong X, Hu T, Hu H, Bao C. 2019a. Transcriptome-wide identification and characterization of circular RNAs in leaves of Chinese cabbage (Brassica rapa L. ssp. pekinensis) in response to calcium deficiency-induced tip-burn. Sci rep. 9(1):1–9. doi:10.1038/s41598-019-51190-0.
- Wang X, Huang Q, Hao Q, Ran S, Wu Y, Cui P, Yang J, Jiang C, Yang Q. 2018. Insecticide resistance and enhanced cytochrome P450 monooxygenase activity in field populations of Spodoptera litura from Sichuan, China. Crop Prot. 106:110–116. doi:10.1016/j.cropro.2017.12.020.
- Wang X, Lou L, Su J. 2019b. Prevalence and stability of insecticide resistances in field population of Spodoptera litura (Lepidoptera: Noctuidae) from Huizhou, Guangdong province, China. J Asia-Pac Entomol. 22:728–732. doi:10.1016/j.aspen.2019.05.009.
- Wu H, Liu Y, Shi X, Zhang X, Ye C, Zhu KY, Zhu F, Zhang J, Ma E. 2020. Transcriptome analysis of antennal cytochrome P450s and their transcriptional responses to plant and locust volatiles in Locusta migratoria. Int J Biol Macromol. 149:741–753. doi:10.1016/j.ijbiomac.2020.01.309.
- Xiong W, Gao S, Lu Y, Wei L, Mao J, Xie J, Cao Q, Liu J, Bi J, Song X. 2019. Latrophilin participates in insecticide susceptibility through positively regulating CSP10 and partially compensated by OBPC01 in Tribolium castaneum. Pestic Biochem Phys. 159:107–117. doi:10.1016/j.pestbp.2019.06.005.
- Yang H, Dong J, Sun Y, Hu Z, Lv Q, Li D. 2020. Antennal transcriptome analysis and expression profiles of putative chemosensory soluble proteins in Histia rhodope Cramer (Lepidoptera: Zygaenidae). CBPD. 33:100654. doi:10.1016/j.cbd.2020.100654.
- Yang X, Xie W, Wang SL, Wu QJ, Pan HP, Li RM, Yang NN, Liu BM, Xu BY, Zhou X. 2013. Two cytochrome P450 genes are involved in imidacloprid resistance in field populations of the whitefly, Bemisia tabaci, in China. Pestic Bioche Phys. 107:343–350. doi:10.1016/j.pestbp.2013.10.002.
- Yu H, He L, Li ZQ, Li N, Yang YYO, Huang GH. 2019. Altering of host larval (Spodoptera exigua) calcineurin activity in response to ascovirus infection. Pest Manag Sci. 76:1048–1059. doi:10.1002/ps.5615.
- Yu H, Li ZQ, He L, Yang YYO, Li N, Huang GH. 2018. Response analysis of host Spodoptera exigua larvae to infection by Heliothis virescens ascovirus 3h (HvAV-3h) via transcriptome. Sci. Rep. 8:5367. doi:10.1038/s41598-018-23715-6.
- Zaka SM, Abbas N, Shad SA, Shah RM. 2014. Effect of emamectin benzoate on life history traits and relative fitness of Spodoptera litura (Lepidoptera: Noctuidae). Phytoparasitica. 42:493–501. doi:10.1007/s12600-014-0386-5.
- Zhang B, Liu H, Helen HS, Wang JJ. 2011. Effect of host plants on development, fecundity and enzyme activity of Spodoptera exigua (Hübner) (Lepidoptera: Noctuidae). Agr Sci China. 10:1232–1240. doi:10.1016/S1671-2927(11)60114-4.
- Zhang XQ, Yan Q, Li LL, Xu JW, Mang D, Wang XL, Hoh HH, Ye J, Ju Q, Ma Y. 2020a. Different binding properties of two general-odorant binding proteins in Athetis lepigone with sex pheromones, host plant volatiles and insecticides. Pestic Biochem Phys. 164:173–182. doi:10.1016/j.pestbp.2020.01.012.
- Zhang Y, Dong X, Liu J, Hu M, Zhong G, Geng P, Yi X. 2012. Molecular cloning, expression and molecular Modeling of chemosensory protein from Spodoptera litura and its binding properties with Rhodojaponin III. PloS one. 7:e47611. doi:10.1371/journal.pone.0047611.
- Zhang YN, Xu JW, Zhang XC, Zhang XQ, Li LL, Yuan VX, Mang DZ, Zhu XY, Zhang F, Dewer Y. 2020b. Organophosphorus insecticide interacts with the pheromone-binding proteins of Athetis lepigone: implication for olfactory dysfunction. J Hazard Mater. 397:122777. doi:10.1016/j.jhazmat.2020.122777.
- Zhao J, Sun Y, Xiao LB, Tan YA, Jiang YP, Bai LX. 2018. Vitellogenin and vitellogenin receptor gene expression profiles in Spodoptera exigua are related to host plant suitability. Pest Manag Sci. 74:950–958. doi:10.1002/ps.4794.
- Zheng XL, Cong XP, Wang XP, Lei CL. 2011. A review of geographic distribution, overwintering and migration in Spodoptera exigua Hubner (Lepidoptera: Noctuidae). J Entomol Res Soc. 13:39–48.
- Zhou C, Liu Y, Yu W, Deng Z, Gao M, Liu F, Mu W. 2011. Resistance of Spodoptera exigua to ten insecticides in Shandong, China. Phytoparasitica. 39:315. doi:10.1007/s12600-011-0157-5.
- Zhu J, Ban LP, Song LM, Liu Y, Pelosi P, Wang GR. 2016. General odorant-binding 765 proteins and sex pheromone guide larvae of Plutella xylostella to better food. Insect Biochem Mol Biol. 72:10–19. doi:10.1016/j.ibmb.2016.03.005.
- Zuo YY, Huang JL, Wang J, Feng Y, Han TT, Wu YD, Yang YH. 2018. Knockout of a P-glycoprotein gene increases susceptibility to abamectin and emamectin benzoate in Spodoptera exigua. Insect Mol Biol. 27:36–45. doi:10.1111/imb.12338.