Abstract
Nephrotoxicity of gasoline fumes has been documented, but the mechanisms underlying the toxicity remain vague. This study determined the residue of gasoline components in the kidney of 72 male albino rats with a view to providing the basis for a detailed understanding of the nephrotoxicity of gasoline fumes. The rats were randomized into 6 groups and daily exposed to distilled water (control) or gasoline fumes for 1, 3, 5, 7 and 9 h for 10 weeks. Some standard clinical blood biochemistry, activities of some kidney antioxidant and membrane-bound ATPase enzymes and kidney histological changes were examined. Gasoline hydrocarbons were extracted, identified and quantified in the kidney of rats with Gas Chromatography-Mass Spectrometry (GC-MS). Significant (p < 0.05) changes were observed in the biochemical parameters examined in the blood and kidney of the exposed rats compared to the control. Residues of some gasoline metabolites including 2,3-diphenylcyclopropyl) methyl phenyl sulfoxide; Cyclotrisiloxane and 2.2-Paracyclophane were identified in the kidney of rats exposed to gasoline fumes. The kidney of the exposed rats also displayed mild histological changes. Retention of some hydrocarbons in the kidney of rats exposed to gasoline fumes could potentially result in oxidative stress capable of inducing renal dysfunction.
Key policy highlights
Continuous exposure to gasoline fumes for varying hours over a period of time may result in the retention of some gasoline hydrocarbons and metabolites in the kidney of the exposed animal.
Presence of gasoline hydrocarbons and metabolites in the kidney may induce a high production of reactive oxygen species and consequently lead to oxidative damage to the organ.
Metabolic process of gasoline hydrocarbons in the kidney of the exposed animal varies with the hours of exposure.
Introduction
The global production of petroleum resources such as gasoline or petrol is on the increase due to an increase in oil demand. Gasoline or petrol is highly flammable and contains over 2000 hydrocarbons including some additives. Although the percentage composition of the hydrocarbons in this product largely depends on performance requirements, geographical location and additives, gasoline is generally made of highly volatile alkanes, alkenes and aromatic hydrocarbons, which can release toxic vapor or fumes that contain benzene, toluene, ethylbenzene and xylene (BTEX) into the environment (Office of the Environmental Health Hazard Citation2014).
Since Nigeria is known to be the largest oil-producing country in Africa (US Department of Energy Citation2019), the number of petrochemical industries including gas filling stations and petroleum exploration has significantly increased over the years. Meanwhile, a report by Carlos and Donna (Citation2008) indicated that these petrochemical industries are the major contributors to environmental pollution.
The use of gasoline or petrol in Nigeria is on the increase. It is mainly used as fuel to power vehicles and electricity generators in homes and industries (Huckabay et al. Citation1995; Isa et al. Citation2013). The rise in the daily use of gasoline has resulted in the high frequency at which humans are exposed to its fumes. Therefore, inhalation exposure is the main route through which humans including motorists and attendants are exposed to gasoline fumes (Health Protection Agency Citation2007). It is important to state here that toxic effects associated with exposure to gasoline fumes in experimental animal studies have been documented. But Chi (Citation2015) noted the length of exposure time as the major factor that determines the severity of the effects. Some of the reported toxicity of gasoline fumes include developmental abnormality in rats (Roberts et al. Citation2014); alterations to metabolic processes (Owagboriaye et al. Citation2018); nephrotoxicity (Uboh et al. Citation2009); DNA damage (Schreiner et al. Citation2014) and hepatotoxicity (Owagboriaye et al. Citation2017). But the reported nephrotoxicity of gasoline fumes in animals is of major interest.
The role of the kidney in the process of urine formation, through which some gasoline metabolites could be eliminated, has been documented (Miller and Harley Citation1996). Uboh et al. (Citation2009) reported nephrotoxicity of gasoline fumes in albino rats by only monitoring some serum renal markers and kidney histopathology. Early kidney dysfunction associated with occupational exposure to sub-threshold limit value levels of some gasoline constituents was documented by Neghab et al. (Citation2015). In addition, Mahmood et al. (Citation2013) reported nephrotoxicity of gasoline fumes among gasoline-filling station workers in Sulaimani city using plasma protein profile and some renal functions as diagnostic markers of toxicity. However, none of the studies indicates the likely mechanisms underlying the observed nephrotoxicity of gasoline vapor.
Although oxidative stress, an imbalance between the production of reactive oxygen species and (ROS) and cellular antioxidant defence system, has been implicated in the molecular mechanisms underlying pathophysiological conditions in animals exposed to gasoline fumes (Alses and Alzeer Citation2021). Renal dysfunction observed among gas station workers exposed to gasoline in Northern Ethiopia was attributed to increased ROS production (Asefaw et al. Citation2020). Exposure to petroleum hydrocarbon has been shown to induce renal dysfunction via oxidative stress in the kidney of Sprague Dawley rats (Azeez et al. Citation2013). But it remains unknown if the components of gasoline could be retained in the kidney of animals exposed to its fumes and potentiate the induction of oxidative stress. On the order hand, Uchida et al. (Citation1993) had earlier reported no effect on renal function following exposure to some gasoline constituents. This shows that studies on the nephrotoxicity of gasoline fumes in animals remain inconclusive and unclear and the need for a mechanism-based approach study.
In view of this, we designed this study to determine the residue of gasoline components in the kidney of exposed animals with a view to providing the basis for a detailed understanding of the nephrotoxicity status of gasoline fumes.
Materials and methods
Animal and gasoline
We used a total of seventy-two (72) adult male Wistar albino rats of 9–10 weeks old (183 ± 10 g) for this study. The rats were acquired from the Department of Zoology and Environmental Biology, Olabisi Onabanjo University (OOU), Ago-Iwoye. We acclimatized the rats for 7 days in our laboratory under 25 ± 5°C and 65 ± 5% Relative Humidity before the exposure scenario. The rats were individually placed in wooden cages with free access to food and water. Gasoline (premium blend) was obtained from the Nigerian National Petroleum Cooperation (NNPC) gas station in Ijebu North Local Government Area, Southwest, Nigeria.
Study design
The inhalation exposure method recently described in Owagboriaye et al. (Citation2021, Citation2022) was adopted for the present study. The rats were randomly assigned to 6 groups and exposed to distilled water (control) or gasoline fumes in exposure chambers for 1, 3, 5, 7 and 9 h per day for 10 weeks. Before the exposure scenario, we saturated the chamber with gasoline fumes by placing 3 calibrated 500 mL cans containing 300 ml of gasoline in the chamber for an hour. The rats were then exposed to the fumes emanating from the gasoline for the required hour per day. After the expiration of the exposure hour, the rats were moved to another section free of gasoline fumes. We followed the guidelines of the ethical committee in the animal care unit of OOU (approval number: OOU/SCIENG/EC/0002/230620) and regulation CEE 86/609 to perform the experiment.
Collection of samples and preparation
The methods of sample collection and preparations were in accordance with Owagboriaye et al. (Citation2021; Citation2022). Retro orbital sinus technique with micro haematocrit tube was used to collect blood sample into a plain sample tube and serum was separated within 1 h after collection. The rats were sacrificed by cervical dislocation and the kidney was excised and rinsed in saline solution. The excised kidney was divided into two portions. A portion was homogenized in 10%, w/v phosphate buffer (pH 7.4) and centrifuged at 3000xg for 10 min and the supernatant obtained was used for the biochemical assays. The remaining portion was fixed in 10% neutral-buffered formalin for histopathological investigations.
Analysis of serum urea, creatinine and uric acid
Serum urea and creatinine were analysed with Chemistry Hitachi Model 917 multichannel analyzer (Roche Diagnostics, Indianapolis, IN). Serum uric acid was determined with a DiaSys reagent kit, which employed an enzymatic photometric test with TBHBA (2, 4, 6-tribromo-3- hydroxybenzoic acid) (Fossati et al. Citation1980). We used the Bio-Rad Quality controls to check the accuracy and precision.
Determination of ROS and oxidative stress parameters in the kidney of experimental rats
The level of ROS in the kidney tissue was determined by incubating 100 µL of liver aliquots with 5 µL 2′,7′-dichlorodihydrofluorescin diacetate (DCFH-DA) at 37°C for 60 min as previously described in Pérez-Severiano et al. (Citation2004) and Owagboriaye et al. (Citation2021, Citation2022). The thiobarbituric acid reactive substance (TBARS) assay described in Okhawa et al. (Citation1979), which involves the measurement of malondialdehyde (MDA) was employed for the determination of lipid peroxidation. The superoxide dismutase (SOD) activity was determined according to Soon and Tan (Citation2002). The amount of enzyme needed to yield 50% inhibition of nitroblue tetrazolium (NBT) per minute indicates a unit of SOD. The method of Hassan and Barakat (Citation2008) was followed for the determination of reduced glutathione (GSH) concentration. Catalase (CAT) activity determination was in accordance with Goth (Citation1991) and a 1µmol decrease in a hydrogen peroxide/minute denotes one unit of the enzyme. We used GSH and hydrogen peroxide as the substrates for the estimation of GPx activity in the kidney (Rotruck et al. Citation1973). A total of 1 µg of GSH consumed/minute indicates a unit of GPx.
Estimation of serum electrolytes
The colorimetric method was used for the determination of Na+, K+, HCO3– and Cl– in the serum of the experimental rats. Sodium was precipitated as sodium magnesium uracyl acetate and ethyl alcohol was used for the protein precipitation. The precipitated salts were removed by centrifugation and uracyl in excess was read against a blank with a known concentration of uracyl. Potassium was precipitated as potassium sodium cobaltinitrite salt. The precipitated salt was analysed photometrically by using the green colour produced through the addition of chloride (Teitz et al. Citation1986). Serum bicarbonate was determined by measuring the rate of disappearance of NADH at 415 nm on Roche Cobas 6000 autoanalyzer series (Roche Diagnostic Corporation, Indianapolis) while serum chloride was determined with the Biolabo chloride colourimetric test kits (BIOLABO SAS, Maizy, France).
Estimation of membrane-bound ATPase activity in the kidney
We determined the activities of membrane-bound ATPase enzymes (including Ca2+ATPase, Na+/K+ATPase and Mg2+ATPase) in the kidney homogenates of the experimental rats according to the protocol described in Dedeke et al. (Citation2018) and Owagboriaye et al. (Citation2022). Protein was estimated according to Lowry et al. (Citation1951).
Extraction, identification and quantitative determination of hydrocarbons in the kidney tissue
Hydrocarbons in the kidney of the rats were extracted, identified and determined using the protocols described in Lintas et al. (Citation1978); Faruq et al. (Citation2012); Henley et al. (Citation2014) and as modified in Owagboriaye et al. (Citation2021, Citation2022). Gasoline hydrocarbons in the kidney were extracted by dissolving 2 g of the kidney tissue (already washed with diethyl ether) in 10 mL dichloromethane or n-pentane and ultra-sonicated. The mixture was eluted, evaporated and filtered following the modified protocol described in Owagboriaye et al. (Citation2021; Citation2022). Excess moisture was removed from the filtrate with anhydrous sodium sulfate and later concentrated at 30°C on a rotary evaporator. The extract was eluted with a mixture (1:1) of pentane and diethyl ether and the eluate was collected in a graduated flask. We added iso-octane to the eluate and concentrated it to 1 mL on a rotary evaporator. The concentrated extracts were analysed for their hydrocarbon compositions on gas chromatography (7890A Model) coupled with Mass Selective Detector (MSD Model 5975C). The oven temperature was initially set at 40°C for 2 min and gradually increased to 300°C for 11 min. A sample volume of 1µ/L was injected and separated on Agilent technologies HP5MS column (30 m × 0.25 mm × 0.320 µm). We used Helium gas at 65 psi as the carrier gas. Each separated compound was identified at the end of each run by comparing its total ion chromatogram (TIC) and mass spectrum with the 2004 NIST e-library as previously described in Owagboriaye et al. (Citation2021, Citation2022).
Histological investigation of the kidney
We routinely processed the kidney excised for histopathological investigation and sectioned at 4-5um thick. The obtained liver sections were stained with hematoxylin and eosin (H&E) stain before mounting in a neutral DPX medium as described in Owagboriaye et al. (Citation2021). Prepared slides were examined at 400 X magnifications.
Statistical methods
Statistical analysis of the data was carried out with version 20.0 of the IBM-SPSS Statistical Package (IBM Corp. Citation2011). The mean values comparison was done through the Analysis of Variance (ANOVA) and the results were presented as Mean ± Standard Error of Mean (SEM). We used the Student–Newman–Keuls (SNK) to carry out the post hoc test and a significant level was set at a probability value less than 0.05.
Results
Levels of serum urea, creatinine and uric acid
The serum levels of kidney biomarkers (including serum urea, creatinine and uric acid) of rats exposed to gasoline fumes for varying hours are shown in Figure . These markers were significantly (p < 0.05) increased in rats exposed to gasoline fumes for 9 h compared to control. There were no significant (p > 0.05) differences in the levels of serum urea, creatinine and uric acid of rats exposed to gasoline fumes for 1 and 3 h compared to control.
Levels of oxidative stress parameters in the kidney of experimental rat
The results of oxidative stress markers in the kidney of the rats exposed to gasoline fumes for varying hours are presented in Table . There were significant (p < 0.05) reductions in the activities of SOD, catalase, GPx and concentration of GSH in the kidney of rats exposed to gasoline fumes for 3, 5, 7 and 9 h compared to control. However, these markers were observed to insignificantly (p > 0.05) (except SOD) increase in the kidney of rats exposed to gasoline fumes for 1 h compared to control. On the other hand, the production of ROS was observed to significantly (p < 0.05) increase in the kidney of the experimental rats (except those exposed for 1 h) with an increase in the daily hours of gasoline exposure.
Table 1. Oxidative stress parameters in the kidney of rats exposed to gasoline fumes for varying hours.
Level of MDA in the experimental rat
There was no significant (p > 0.05) difference in the level of MDA between rats exposed to gasoline fumes for 1 h and control as well as between those exposed to gasoline fumes for 5 and 7 h. In general, MDA was observed to increase in the kidney of the experimental rats with an increase in the daily hours of gasoline fumes exposure (Figure ).
Levels of serum electrolytes
The levels of serum electrolytes of rats exposed to gasoline fumes for varying hours are shown in Table . Results showed that K+ was significantly increased (p < 0.05) in rats exposed to gasoline fumes for 9 h compared to control. The level of K+ in rats exposed to gasoline fumes for 3, 5 and 7 h was not significantly different (p > 0.05). The level of HCO3− in rats exposed to gasoline fumes for 5, 7 and 9 h was not significantly different (p > 0.05). On the other hand, levels of Na+ and Cl- were observed to significantly reduce with an increase in the daily hours of gasoline exposure.
Table 2. Serum electrolytes (nmol/L) of rats subjected to varying hours of daily gasoline exposure.
The activity of membrane-bound ATPase enzyme in the kidney of experimental rats
Figure presents the activities of membrane-bound ATPase enzymes (Mg2+ATPase, Ca2+ ATPase, Na+/K+ ATPase) in the kidney of rats daily exposed to gasoline fumes for varying hours. There were no significant (p > 0.05) differences in the activities of the kidney membrane-bound enzymes between the control and rats exposed to 1 and 3 h of gasoline fumes. However, significant (p < 0.05) reductions in the activities of the membrane-bound enzymes were observed in rats exposed to 5, 7 and 9 h of gasoline fumes.
Levels of hydrocarbon compositions of gasoline residues and their metabolites in the kidney of experimental rat
The gasoline used in the present study contains 23 hydrocarbons (Supplementary Table 1). The gasoline contains a large amount of toluene and a trace amount of azulene and cyclopentane. A total of 15 hydrocarbons were observed in the kidney of rats exposed to gasoline fumes (Table ). Kidney of rats exposed to gasoline fumes for 1 h/day recorded the highest number (13) of hydrocarbons. Also, 2,3-diphenylcyclopropyl) methyl phenyl sulfoxide was present in the kidney of rats subjected to varying periods of daily gasoline exposure. This was significantly higher in the kidney of rats exposed to gasoline fumes for 5 h/day. Ethylbenzene was only found in the kidney of rats exposed to gasoline fumes for 1 h/day while paracyclophane was only present in the kidney of rats exposed to gasoline fumes for 5 h/day.
Table 3. Hydrocarbon compositions (ppm) in the kidney of rats subjected to varying periods of daily gasoline fumes exposure.
Kidney histopathological examination
Photomicrographs of the kidney of rats exposed to gasoline fumes at varying hours are shown in Figure . Normal histoarchitecture of renal glomerular capillaries, glomerular space, proximal convoluted and distal convoluted tubules with normal epithelium were observed in the control. Kidney sections of rats exposed to gasoline fumes for 1 and 3 h showed mild distortions characterized by distal convoluted tubule with a large lumen. Severe cytoarchitectural lesions, characterized by degenerated glomerular capillaries with enlarged glomerular space, intertubular blood vessel dilation with haemolysed blood vessel, the appearance of pyknotic nuclei, congestion of interstitial cells and infiltration of the mononuclear cell around the glomerular capillaries as well as a focal area of inflammation were observed in the kidney sections of rats exposed to gasoline fumes for 5 h. Kidney sections of rats exposed to gasoline fumes for 7 and 9 h mainly showed slight degeneration characterized by disseminated congestion of vessels.
Figure 4. Photomicrographs of the kidney sections of rats exposed to gasoline fumes. H&E stain 200X. (a) Control showing normal cytoarchitecture of renal glomerular capillaries, glomerular space, proximal convoluted tubule and distal convoluted tubules. (b) Exposed to gasoline fumes for 1 h showing mild distal convoluted tubule with large lumen (red arrow). (c) Exposed to gasoline fumes for 3 h showing mild distal convoluted tubule with large lumen (red arrow). (d) Exposed to gasoline fumes for 5 h showing degenerated glomerular capillaries with enlarged space (black arrow), intertubular blood vessel dilation (blue arrow), haemolysed blood vessel (green arrow) and pyknotic nuclei as well as, congestion of interstitial cell and infiltration of mononuclear cell around the glomerular capillaries (yellow arrow). (e) Exposed to gasoline fumes for 7 h showing disseminated congestion of vessels (purple arrow). (f) Exposed to gasoline fumes for 9 h showing disseminated congestion of vessels (purple arrow). (See colour figure online).
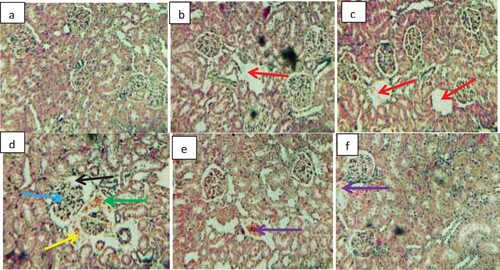
Discussion
Serum creatinine, uric acid and urea are good biomarkers of renal damage by environmental pollutants. Serum creatinine, a breakdown product of creatine phosphate, reflects the rate of renal (glomerular) filtration (Gregory et al. Citation2004) and a rise in serum urea has been recognized as a good diagnostic marker of renal dysfunction (Tizhe et al. Citation2013). Thus, the increased levels of serum uric acid, urea and creatinine observed in the rats exposed to gasoline fumes may indicate a reduced glomerular filtration process and dysfunction of the kidney tubules.
Body cells are generally protected against the damaging influence of ROS by the enzymatic (CAT, GPx, SOD) and non-enzymatic (GSH) antioxidant defence system (Chelikani et al. Citation2004). An imbalance between the production of ROS and antioxidant defence activity results in oxidative stress. It is clearly seen in this study that exposure to gasoline fumes increased the production of ROS, which overwhelmed the protective roles of the antioxidant molecules in the kidney of the exposed rats and consequently resulted in peroxidation of the renal membrane polyunsaturated fatty acids. The mechanism behind the observed increase in ROS production is unknown, but suggestions of some possible mechanisms that could explain this finding can be made. First, gasoline fumes could have enhanced the electron transferring activity of NADPH oxidase from NADPH to form superoxide, which is rapidly converted to hydrogen peroxide in the kidney of the exposed rats (Chabrashvili et al. Citation2002; Araujo and Wilcox Citation2014; Holterman et al. Citation2015). Second, superoxide generated by NADPH oxidase could, in turn, increase the production of another superoxide in complex I and complex III of the electron transport chain in the mitochondria by influencing intracellular regulatory systems that increase intracellular calcium, open K+ATP channels and increase membrane potential (Brodsky et al. Citation2002; Thomas et al. Citation2008). Third, gasoline fumes could have interfered with the renal vasculature cells such as endothelial cells, adventitial fibroblast, and resident and inflammatory cells, to produce ROS that promotes renal pathology (Ratliff et al. Citation2016). ROS generation has been shown to have direct impacts on vasoreactivity in renal vessels with increased ROS associated with enhanced renal vascular resistance (Welch et al. Citation2001). Fourth, the presence of gasoline fumes could have prompted mesangial and endothelial cells to recruit leukocytes and infiltrate the glomerulus and perivascular regions of the kidney (Satriano et al. Citation1997). It has been reported that infiltrating leukocytes, which invade the glomerulus and perivascular regions, release a large number of proinflammatory cytokines [including TNFα and interleukin-1beta (IL-1β)] and undergo a respiratory burst to release superoxide, hydroxyl radical-like species and hydrogen peroxide (Rosen et al. Citation2002; Yang Citation2011). Meanwhile, a positive correlation has been shown to exist between oxidative stress, inflammation and organ dysfunctions in animals exposed to toxic agents (Mahmoud et al. Citation2017; Elgebaly et al. Citation2018). Since we did not investigate the role of pro-inflammatory cytokines in this study, we recommend further study in this area. In addition, it will be interesting to know the status of other oxidative stress markers such as lipid hydroperoxides, carbonyl protein, total sulphydryl groups and GSH-dependent enzymes (including glutathione reductase and glutathione-s-transferase) in the kidney of animals exposed to gasoline fumes. Meanwhile, the major hydrocarbon in the gasoline that could be responsible for the oxidative stress observed in the kidney of the exposed rats is unknown. But we suspect benzene since scientific evidence showed induction of oxidative stress among workers in gas filling stations who are occupationally exposed to benzene (Uzma et al. Citation2010). In addition, benzene metabolites including 1, 2, 4-benzenetriol and hydroquinone have been documented to induce oxidative stress by generating ROS and impairing the antioxidant defence system (Uzma et al. Citation2010). Thus, the presence of benzene in the kidney of the exposed rats may be responsible for the oxidative stress-induced in the organ.
Electrolyte balance in animals plays a major role in regulating the fluid distribution and maintaining osmotic pressure, cellular acido-basic equilibrium and neuro-muscular activity. Serum concentrations of electrolytes including Na+, K+, Cl- and HCO3- are vital tools used to indicate the functional integrity of the kidney and any disturbance to the homeostatic balance of the electrolytes could indicate impairment to the kidney function (Yakubu et al. Citation2003; Garba et al. Citation2007). The observed imbalance in the serum electrolytes of the rats exposed to gasoline fumes may indicate abnormalities in the proximal convoluted tubules of their kidneys (Krishma and Ramachandran Citation2009).
As reported by Kempaiah and Srinivasan (Citation2006), membrane-bound ATPase enzymes are good markers of membrane dysfunctions under oxidative stress. The enzymes supply energy via hydrolytic reaction to control membrane permeability and transportation of ions including Ca2+, K+ and Na+ across the membrane (Langeswaran et al. Citation2012). The reduced Na+/K+-ATPase and Mg2+-ATPase activities observed in the kidney of rats exposed to gasoline fumes could be one of the consequences of an increased MDA in the tissue. Because it has been reported that both Mg2+ATPase and Na+/K+ATPase depend on lipid for their activities and are both sensitive to the peroxidation of lipid (Miltonprabu et al. Citation2016). Likewise, Miltonprabu et al. (Citation2016) suggested that inhibition of Na+/K+ATPase could result in disruption of membrane permeability orchestrated by a reduction in sodium efflux. This event may result in Ca2+ ion leakage into the cytosol and consequently, reduce the membrane Ca2+ATPase activity as previously suggested in Owagboriaye et al. (Citation2021, Citation2022).
It seems that our study is the first to show the residue of some gasoline compositions and metabolites such as 2,3-diphenylcyclopropyl) methyl phenyl sulfoxide, 1,3,5-Triphenyl-1,5-pentanedione, 13-Tetradece-11-yn-1-ol, Cyclotrisiloxane and 2.2-Paracyclophane in the kidney of animals exposed to gasoline fumes for varying hours. This shows the possibility of gasoline fumes condensing and settling in the renal tissue of the exposed animal. Meanwhile, some hydrocarbons were metabolized in the renal tissue, but it seems that the metabolic process depends on the exposure time. It has been reported that the metabolism of xenobiotics may sometimes result in toxic metabolites capable of inflicting serious injuries on the body system (Schlichting et al. Citation2000; Murphy Citation2001). In view of this, it remains unknown if the nephrotoxicity observed in this study is due to the gasoline residues or its metabolites.
Exposure to gasoline fumes and hydrocarbons resulted in various histopathological changes observed in the kidney tissue of the exposed rats. Kidney sections of the rats showed cytoarchitectural lesions characterized by degenerated glomerular capillaries with enlarged glomerular space, intertubular blood vessel dilation with haemolysed blood vessel, and the appearance of pyknotic nuclei, congestion of interstitial cells and infiltration of mononuclear cells around the glomerular capillaries. In addition, slight degeneration characterized by disseminated congestion of vessels was also observed. This finding confirms Uboh et al. (Citation2009) who also observed various degrees of histological lesions in the kidney of rats exposed to gasoline fumes for varying hours. This clearly indicates that the kidney is one of the target organs of gasoline fumes-induced injury.
Conclusion
This study shows that exposure to gasoline fumes can lead to the retention of some hydrocarbons in the kidney of the exposed animal and consequently result in renal dysfunction through oxidative stress. Additional study is required to understand the main hydrocarbon in gasoline fumes that could be responsible for the observed nephrotoxicity.
Supplemental Material
Download MS Word (17.2 KB)Acknowledgements
The management of Olabisi Onabanjo University and the Department of Zoology and Environmental Biology granted us permission to use its facilities for this study. Conceptualization – F.O.; Design/Methodology – F.O. and G.D.; Investigation – F.O.; S.A.; R.O.; T.S. and Ad.Ad.; Data analysis and interpretation – F.O. and Ad.Al; Writing – original draft preparation – F.O.; Writing – review and editing – all authors; Supervision – G.D.; project administration – F.O.; All authors agree to be accountable for integrity and accuracy in all aspects of the work.
Disclosure statement
No potential conflict of interest was reported by the author(s).
Data availability statement
The data that support the findings of this study are openly available in Zenodo at https://zenodo.org/record/7104679#.YyxE8nbMLDc.
References
- Alses M, Alzeer S. 2021. Evaluation of some biological parameters of gasoline station attendants in Damascus, Syria. Heliyon. 7:e07056.
- Araujo M, Wilcox CS. 2014. Oxidative stress in hypertension: role of the kidney. Antioxid Redox Signal. 20:74–101.
- Asefaw T, Wolde M, Edao A, Tsegaye A, Teklu G, Tesfay F, Gebremariam G. 2020. Assessment of liver and renal function tests among gasoline exposed gas station workers in Mekelle city, Tigray region, Northern Ethiopia. PLoS One. 15(10):e0239716.
- Azeez OM, Akhigbe RE, Anigbogu CN. 2013. Oxidative status in rat kidney exposed to petroleum hydrocarbons. J Nat Sc Biol Med. 4:149–154.
- Brodsky SV, Gao S, Li H, Goligorsky MS. 2002. Hyperglycemic switch from mitochondrial nitric oxide to superoxide production in endothelial cells. Am J Physiol Heart Circ Physiol. 283:2130–2139.
- Carlos JSP, Donna M. 2008. Human mercury exposure and adverse health effects in the Amazon: a review. Cad Saúde Pública. 24(4):503–520.
- Chabrashvili T, Tojo A, Onozato ML, Kitiyakara C, Quinn MT, Fujita T, Welch WJ, Wilcox CS. 2002. Expression and cellular localization of classic NADPH oxidase subunits in the spontaneously hypertensive rat kidney. Hypertension. 39:269–274.
- Chelikani P, Fita I, Loewen PC. 2004. Diversity of structures and properties among catalases. Cell Mol Life Sci. 61(2):192–208.
- Chi N. 2015. 8 occupational health hazards in oil and gas industry that you must know (part1). http://oilandgasmanpowerprovider.blogspot.com/2015/10/occupational-health-hazards-in-oil-and-gas-industry.html.
- Dedeke GA, Owagboriaye FO, Ademolu KO, Olujimi OO, Aladesida AA. 2018. Comparative assessment on mechanism underlying renal toxicity of commercial formulation of roundup herbicide and glyphosate alone in male albino rat. Int J Toxicol. 37(4):285–295.
- Elgebaly HA, Mosa NM, Allach M. 2018. Olive oil and leaf extract prevent fluoxetine-induced hepatotoxicity by attenuating oxidative stress, inflammation and apoptosis. Biomed Pharmacother. 98:446–453.
- Faruq UZ, Runde M, Danshehu BG, Yahaya HN, Zuru AA, Muhammad AB. 2012. Comparative studies of gasoline samples used in Nigeria. Niger J Basic Appl Sci. 20(2):87–92.
- Fossati P, Prencipe L, Berti G. 1980. Use of 3,5-dichloro2-hydroxy-benzenesulfonic acid/ 4-aminophenazone chromogenic system in direct enzymatic assay of uric acid in serum and urine. Clin Chem. 26:227–231.
- Garba S, Adelaiye A, Mshelia L. 2007. Histopathological and biochemical changes in the rats kidney following exposure to a pyrethroid based mosquito coil. J Appl Sci Res. 3:1788–1793.
- Goth L. 1991. A simple method for determination of serum catalase activity and revision of reference range. Clin Chim Acta. 196(3):143–151.
- Gregory L, Birgel Junior E, D’angelino FJ. 2004. Valores de referência dos teores séricos da uréia e creatinina em bovinos da raça Jersey criados no Estado de São Paulo. Influência dos fatores etários, sexuais e da infecção pelo virus da leucose dos bovinos. Arquivos do Instituto Biológico. 71:39–345.
- Hassan AM, Barakat AH. 2008. Assessment of oxidative stress induced by nickel chloride and antioxidant effects of basil (Ocimum basilicum L) and (Thymus vulgarisL). J Genetic Eng Biotech. 6(2):29–38.
- Health Protection Agency. 2007. Petrol: toxicological overview. Prepared by Chilcott RP. CHAPD HQ, United Kingdom. 2nd version; p. 1–16.
- Henley M, Daniel J, Letinski JC, Mario LC, Wayne D, Russell W. 2014. Health assessment of gasoline and fuel oxygenate vapors: generation and characterization of test materials. Regul Toxicol Pharmacol. 70:S13–S17.
- Holterman CE, Thibodeau JF, Kennedy CR. 2015. NADPH oxidase 5 and renal disease. Curr Opin Nephrol Hypertens. 24:81–87.
- Huckabay P, Wendy D, VanCleave C, Ostrander J. 1995. Petroleum sector notebook paper, Cameron University. J Appl Sci Environ Manag. 6:84–86.
- IBM Corporation. 2011. IBM SPSS statistics for windows, version 20.0. Armonk, NY: IBM Corp.
- Isa HA, Hamisu S, Lamin HS, Ya’u MZ, Olayande JS. 2013. The perspective of Nigeria’s projected demand for petroleum products. J Petrol Gas Eng. 4(7):184–187.
- Kempaiah RK, Srinivasan K. 2006. Protective effect of curcumin, capsaicin and garlic on erythrocyte integrity in high fat fed rats. J Nutritional Biochem. 17:471–478.
- Krishma H, Ramachandran AV. 2009. Biochemical alterations induced by the acute exposure to combination of chlorpyrifos and lead in Wistar rats. Biol Med. 1(2):1–6.
- Langeswaran K, Jagadeesan AJ, Balasubramanian MP. 2012. Modulation of membrane bound ATPases and metabolizing enzymes against N-nitosodiethylamine (DEN) induced primary liver cancer in Wistar albino rats. Int J Pharma Bio Sci. 3(2):156–165.
- Lintas C, Balduzzi AM, Bernardini MP, Di Muccio A. 1978. Distribution of hydrocarbons in bovine tissues. Lipids. 14(3):298–303.
- Lowry OH, Rosenbrough NJ, Farr AL, Randal R. 1951. Protein measurement with the folin phenol reagent. J Biol Chem. 193:265–270.
- Mahmood NM, Sharef DM, Hussain SA. 2013. Plasma proteins profile and renal function relative to exposure time of gasoline filling station workers in Sulaimani city. Int J Pharm Pharmaceut Sci. 5(4):334–338.
- Mahmoud AM, Germoush MO, Alotaibi MF. 2017. Possible involvement of Nrf2 and PPARgamma up-regulation in the protective effect of umbelliferone against cyclophosphamide-induced hepatotoxicity. Biomed Pharmacother. 86:297–306.
- Miller AM, Harley JP. 1996. Zoology. Dubuque, Iowa, USA: Wm. C. Brown Publishers.
- Miltonprabu S, Nazimabashir, Manoharan V. 2016. Hepatoprotective effect of grape seed proanthocyanidins on cadmium-induced hepatic injury in rats: possible involvement of mitochondrial dysfunction, inflammation and apoptosis. Toxicol Rep. 3:63–77.
- Murphy PJ. 2001. Xenobiotic metabolism: a look from the past to the future. Drug Metab Dispos. 29(6):779–780.
- Neghab M, Hosseinzadeh K, Hassanzadeh J. 2015. Early liver and kidney dysfunction associated with occupational exposure to sub-threshold limit value levels of benzene, toluene, and xylenes in unleaded petrol. Saf Health Work. 6:312–316.
- Office of Environmental Health Hazard Assessment. 2014. Benzene reference exposure levels technical support document for the derivation of noncancer reference exposure levels appendix D. p. 1–73.
- Okhawa H, Ohishi N, Yagi K. 1979. Assay for lipid peroxides in animal tissues by thiobarbituricacid reaction. Anal Biochem. 95:351–358.
- Owagboriaye FO, Aina SA, Oladunjoye RY, Salisu T, Adenekan A, Dedeke GA. 2021. Insights into the potential mechanism underlying liver dysfunction in male albino rat exposed to gasoline fumes. Egypt J Basic Appl Sci. 8(1):302–316. doi:10.1080/2314808X.2021.1992118.
- Owagboriaye FO, Aina SA, Salisu T, Oladunjoye RY, Adenekan A, Aladesida AA, Dedeke GA. 2022. Insights into the mechanism underlying reproductive toxicity of gasoline fumes in Male albino rat. Comp Clin Path. doi:10.1007/s00580-022-03343-3.
- Owagboriaye FO, Dedeke GA, Aladesida AA, Bamidele JA, Olooto WE. 2018. Assessment of the effect of gasoline fume on stress hormones, antioxidant status and lipid peroxidation in albino rat. J King Saud Univ Sci. 30:393–399.
- Owagboriaye FO, Dedeke GA, Ashidi JS, Aladesida AA, Olooto WE. 2017. Hepatotoxicity and genotoxicity of gasoline fumes in albino rats. Beni Suef Univ J Basic App Sci. 6:253–259.
- Pérez-Severiano F, Santamaría A, Pedraza-Chaverri J, Medina-Campos ON, Ríos C, Segovia J. 2004. Increased formation of reactive oxygen species, but no changes in glutathione peroxidase activity, in striata of mice transgenic for the Huntington’s disease mutation. Neurochem Res. 29:729–733.
- Ratliff BB, Abdulmahdi W, Pawar R, Wolin MS. 2016. Oxidant mechanisms in renal injury and disease. Antioxid. Redox Signal. 25(3). doi:10.1089/ars.2016.6665.
- Roberts LG, Gray TM, Marr MC, Tyl RW, Trimmer GW, Hoffman GM, Murray FJ, Clark CR, Schreiner CA. 2014. Health assessment of gasoline and fuel oxygenate vapors: developmental toxicity in mice. Regul Toxicol Pharmacol. 70(2):S58–S68.
- Rosen H, Crowley JR, Heinecke JW. 2002. Human neutrophils use the myeloperoxidase-hydrogen peroxidechloride system to chlorinate but not nitrate bacterial proteins during phagocytosis. J Biol Chem. 277:30463–30468.
- Rotruck JT, Pope AL, Ganther HE, Swanson AB, Hafeman DG, Hoekstra WG. 1973. Selenium: biochemical role as a component of glutathione peroxidase. Science. 179:588–590.
- Satriano JA, Banas B, Luckow B, Nelson P, Schlondorff DO. 1997. Regulation of RANTES and ICAM-1 expression in murine mesangial cells. J Am Soc Nephrol. 8:596–603.
- Schlichting I, Berendzen J, Chu K, Stock AM, Maves SA, Benson DE, Sweet RM, Ringe D, Petsko G, Sligar SG. 2000. The catalytic pathway of cytochrome p450cam at atomic resolution. Science. 287:1615–1622.
- Schreiner CA, Hoffman GM, Gudi R, Clark CR. 2014. Health assessment of gasoline and fuel oxygenate vapors: micronucleus and sister chromatid exchange evaluations. Regul Toxicol Pharmacol. 70:S29–S34.
- Soon YY, Tan BKH. 2002. Evaluation of the hypoglycemic and antioxidant activities of Morindaofficinalisin streptozotocininduced diabetic rats. Singapore Med J. 43:77–85.
- Teitz NW, Pruden EL, Siggaard-Andersen O. 1986. Electrolytes, blood gases and acid-base balance. In: N. W. Tietz, editor. Textbook of clinical chemistry. Philadelphia (PA): Saunders; p. 1188.
- Thomas SR, Witting PK, Drummond GR. 2008. Redox control of endothelial function and dysfunction: molecular mechanisms and therapeutic opportunities. Antioxid Redox Signal. 10:1713–1765.
- Tizhe EV, Ibrahim ND, Fatihu MY, Igbokwe IO, George BD, Ambali SF, Shallangwa JM. 2013. Serum biochemical assessment of hepatic and renal functions of rats during oral exposure to glyphosate with zinc. Comp Clin Path. 22. doi:10.1007/s00580-013-1740-6.
- Uboh FE, Ekaidem IS, Ebong PE, Umoh IM. 2009. The hepatoprotective effect of vitamin A against gasoline vapor toxicity in rats. Gastro Res. 2(3):162–167.
- Uchida Y, Nakatsuka H, Ukai H, Watanabe T, Liu YT, Huang MY, Wang YL, Zhu FZ, Yin H, Ikeda M. 1993. Symptoms and signs in workers exposed predominantly to xylenes. Int Arch Occup Environ Health. 64:597–605.
- U.S. Department of Energy. 2019. Petroleum and other liquids. Information Administration. Accessed from https://www.eia.gov/petroleum/ on June 6, 2020.
- Uzma N, Kumar BS, Hazari MAH. 2010. Exposure to benzene induces oxidative stress, alters the immune response and expression of p53 in gasoline filling workers. Am J Ind Med. 53(12):1264–1270. doi:10.1002/ajim.20901.
- Welch WJ, Baumgart H, Lubbers D, Wilcox CS. 2001. Nephron pO2 and renal oxygen usage in the hypertensive rat kidney. Kidney Int. 59:230–237.
- Yakubu MT, Bilbis LS, Lawal M, Akanji MA. 2003. Evaluation of selected parameters of rat liver and kidney function following repeated administration of yohimbine. Biokemistri. 15(2):50–56.
- Yang C. 2011. Infection in the kidney. In: Miyata T, Eckardt K., Nangaku M., editors. Studies on renal disorders. New York: Humana Press; p. 293–304.