Abstract
This article describes key material science/technology issues to implement polycrystalline diamond scaffolds to enable processes for biological cells growth relevant for using cells grown in the laboratory for the treatment of human biological conditions. Issues investigated include
1. Synthesis/characterization of microcrystalline diamond (MCD), nanocrystalline diamond (NCD) and transformational ultrananocrystalline diamond (UNCD) coating-based scaffolds. Diamond films were grown on silicon substrates using the hot filament chemical vapor deposition (HFCVD) technique, by which filaments heated to ∼2,300 °C induce cracking of CH4 molecules into C atoms/CHx (x = 1, 2, 3) radicals, growing diamond films via chemical reaction on the substrates’ surface. MCD and NCD + films were grown flowing the H2/CH4 gas mixture. NCD- and UNCD films were grown using the Ar/CH4 gas mixture plus H2 flux (73.5%, 49%, and 9.8%), which for high fluxes, induced increased the concentration of H-containing trans-polyacetylene (T-PA) molecules in UNCD films’ grain boundaries, impacting biological performance.
2. Studies of viability and proliferation of human lung carcinoma cell line (A549) grown on surfaces of MCD, NCD, and UNCD films, using 3-[4,5-dimethylthiazol-2-yl]-2,5-diphenyl tetrazolium bromide (MTT) assay, which showed no significant difference in cell proliferation among the MCD, NCD, and UNCD films.
1. Introduction
Extensive R&D is being done to investigate potential biocompatible materials to enable new generations of implantable medical devices/prostheses/artificial cells into the human body. Materials investigated and/or currently used in implantable medical devices, including metals (eg, gold, iridium, platinum, and titanium), polymers (eg, polytetrafluoroethylene\ and polyurethane), and ceramics (eg, zirconia and zirconia-titanium alloy) [Citation1].
Ti-alloys (Ti-6Al-4V) are being extensively used for dental implants (DI) [Citation1, Citation2], which exhibit ∼15% failure worldwide in the first 4–5 years due to oral fluids that induced chemical corrosion [Citation2, Citation3]. Other prostheses (eg, hips and knees) present similar problems including chemical corrosion [Citation4]. Metals, in general, develop oxide layers upon atmosphere exposure, resulting in body fluid-induced oxide particles ejection, inducing natural human cells death, inflammation of tissues, and other biological degradation effects, as for Ti-alloys DIs [Citation3, Citation5].
A transformational biocompatible material, in the film form, is ultrananocrystalline diamond (UNCD), named UNCD because of grains’ dimensions (3–5 nm), originally developed [Citation6] and patented [Citation7] by Auciello and collaborators. UNCD, NCD (10–100 s nm), and MCD (≥ 1 µm grains) films, grown via microwave plasma chemical vapor deposition (MPCVD) and hot filament chemical vapor deposition (HFCVD) techniques, exhibit unique combination of mechanical, chemical, thermal, and biocompatible properties (see extensive review in chapters of ref. [Citation8] and references there in). The HFCVD technique provides a low-cost versatile process, enabling control of the grain size and surface roughness of polycrystalline diamond films [Citation8–12], doping [Citation8, Citation13], and growth on different substrates [Citation10, Citation14]. Large area HFCVD systems have been used since 2000s to produce commercial industrial products based on UNCD coatings (see ref. [Citation15]), and current R&D for micro-electromechanical systems (MEMS)-based sensors [Citation6, Citation16] and energy generation devices.
Key application of polycrystalline diamond films, relevant to this article, relies on their biocompatibility and surface chemistry, enabling growth of cells and negligible toxicity [see, eg, refs. [Citation8, Citation21] and references there in). These properties are based on the unique sp3 C atom bonds’ structure and chemical surface termination. A relevant process for human cells’ growth on diamond surfaces is the functionalization to produce O or H atom termination. For specific biomedical implantable devices, requiring human cells adhesion is critical to produce hydrophilic surfaces via O-plasma processing, inducing O atoms surface termination [Citation17]. Studies revealed that adsorbed biological materials, such as buffer layers, can enhance cells’ adhesion on the diamond surface [Citation22]. Alternatively, H-terminated diamond surface can be used for sensors or electronic devices [Citation18–20] but exhibit high water drop contact angle, resulting in hydrophobicity and negligible adherence of cells, like blood cells interacting with artificial heart valves or stents, which may result in life threatening thrombus formation [Citation8] or coli bacterium interaction with implantable medical devices [Citation21].
Based on the information presented above, this article is focused on describing results related to biological viability and proliferation of A549 cell line on MCD, NCD, and the UNCD films surfaces, for films grown with different H2 gas flow concentration, tailoring chemical treatments of diamond films’ surfaces.
2. Experimental methods
2.1. Diamond films growth processes
The diamonds films used for cell growth were grown on several silicon (Si) substrates in a Blue-Wave HFCVD system. The Si substrates were seeded with nanocrystalline diamond particles on the surface to induce the diamond film growth using the well know seeding process extensively described in the literature (see reviews in references [Citation6, Citation9] and patent [Citation7]). The parameters for the diamond films’ growth process in the HFCVD system were tailored for growing MCD, NCD, and UNCD films based on previous research [Citation12] (see ).
Table 1. Parameters for the growth of MCD, NCD, and UNCD films. The NCD+, NCD-mid, and NCD- notation refers to the NCD’s grain sizes, which are controlled by the flow of H2 gas in the gas mixture.
2.2. Cell culture on MCD, NCD, and UNCD films’ surfaces
A549 cell line was cultured in Dulbecco’s modified Eagle’s medium (DMEM) supplemented with 10% (v/v) heat-inactivated fetal bovine serum (FBS), penicillin/streptomycin (1%), glutamine (0.75%), and sodium pyruvate (1%). Cells’ cultures were maintained in a humidified atmosphere of 95% air and 5% CO2 at 37 °C. A549 cell lines were passaged by trypsinization (0.05% trypsin/0.53 mM EDTA) every 3 days, centrifuged, re-suspended in a fresh medium, and counted using trypan blue as an exclusion coloring.
2.3. Assay of mitochondrial viability (MTT assay)
Cell viability was determined using the MTT assay (3 – (4,5-dimethylthiazol-2-yl) 5 – (3-carboxymethoxyphenyl) −2 – (4-sulfophenyl) −2H-tetrazolium), which corresponds to a colorimetry test based on the reduction in MTT to formazan crystals by mitochondrial dehydrogenase enzymes. Living cells reduced MTT to formazan, which is quantified by measuring absorbance. The A549 cell line was cultured in a culture flask, and when the growth reached 100% of confluence, the cells were detached from the culture flask by trypsinization, and the cell suspension was counted in Neubauer chamber, and it was adjusted to a concentration 100,000 cells/ml. Then, this cell suspension was deposited, fully covering the surfaces of MCD, NCD, and UNCD film’s grown on Si substrates with an area of 2.25 cm2. Previously, the cells were sterilized by autoclave at 120 °C for 20 min and placed in 12-well plates. The cells were cultured in each well in a volume of 1.5 ml of DMEM, supplemented with 10% FBS, and incubated at 37 °C with 5% CO2 during 48 h. Cells with the same density were seeded in empty wells without any materials, as control. The cells were removed using trypsin, followed by centrifugation at 1,500 rpm (348 x g), and 4 °C for 10 min. Some wells with cells were prepared for counting, using trypan blue. Other cells were used to perform the MTT assay, involving 5 µl of MTT (0.5 mg/ml in a medium) added to the well. Cells grown on the MCD, NCD, and UNCD surfaces and the control surface were incubated for 6 h at 37 °C. Finally, formazan crystals were solubilized in acidified isopropanol and the absorbance of each sample was measured at 570 and 655 nm. The data from this study are reported as the mean ± standard deviation (SD) of three independent assays. The significance of the data between experimental groups was determined by using the ANOVA method, followed by Tuckey-Kramer-type analysis, using a NCSS software 2007 version. Values of p ≤ 0.05 were considered statistically significant.
The results were plotted using a GraphPad prism software version 8.0.2. A detailed schematic of the cell culture process is shown in .
Figure 1. Schematic showing the cell culture process on surfaces of diamond films grown on Si substrates, and the measurement of viability of cell growth via the MTT assay and analysis of cell adherences on surfaces via optical microscopy.
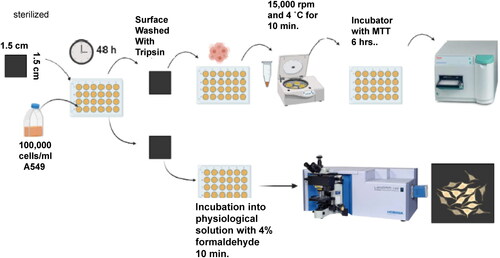
The cell viability was also measured by counts in a Neubauer chamber. The protocol used for counting A549 cells include (1) A549 cells growth on diamond and silicon surfaces; (2) after 48 h, cells were removed by trypsin and centrifuged as described above; (3) cells were counted in a Neubauer chamber, involving optical microscopy; (4) A549 cells were counted over each of the four grid blocks with trypan blue 1:1 diluted. The results are shown in in Section 3.2.
Table 2. A549 Cell count result using Neubauer chamber.
The surface topography of the diamond films was characterized in a Zeiss Supra 40 scanning electron microscope (SEM) operated at 15.00 kV for MCD and NCD films and 10.00 kV for UNCD films. The chemical bonding of diamond films was studied in a LabRAM HR Raman spectrometer equipped with an Ar ion laser (λ = 532 nm) as an excitation source. Optical microscopy images of the adhered cells on the diamond films and silicon were obtained using the microscope of the Raman spectrometer. Prior to observation, the 48 h incubated cells grown on the surface of the MCD, NCD, and UNCD films were washed and incubated during 10 min in physiological solution and fixed with 4% formaldehyde solution. Finally, the MCD, NCD, and UNCD films’ surfaces were observed using the optical microscopy.
3. Discussion
3.1. Raman spectroscopy and SEM analysis of MCD, NCD, and UNCD films
shows typical normalized Raman spectra of MCD, NCD, and UNCD films. Raman spectra of MCD and NCD + films are shown in , which reveal the fingerprint of diamond with the signal of C-C sp3 chemical bonds at 1332 cm−1 and the prominent G-band at ∼1567 cm−1 associated to C-C sp2 chemical bond of C atoms in the gran boundaries (see review in ref. [Citation5]). The difference between the high of the diamond 1332 cm−1 peak of MCD and NCD films is correlated with the grain size [Citation23]; the only difference between both films were the growth time (8 h for MCD and 4 h for NCD + films). The Raman spectra shown in , of the three films grown with different gas flows, correspond to the NCD-mid film grown with 73.9% H2, NCD- film grown with 48% H2 and UNCD film grown with 9.8% H2. The three films show two main bands, the 1344 cm−1 signal that is associated to the D-band, encapsulating the peak at 1332 cm−1 and the Raman signal at ∼1580 cm−1, corresponding to the G-band which has been shown, for microcrystalline diamond films, to correlate with sp2 chemical bonds of C-C atoms in the grain boundaries of the films, but not in a graphite structure [Citation5, Citation23]. In addition, two Raman signals at ∼1164 cm−1 and ∼1489 cm−1, respectively, have been identified as correlated with trans-polyacetylene (T-PA)/C-H molecules, with chemical bonds associated with C-C stretching and C = C stretching in T-PA molecules, respectively [Citation17, Citation18]. The intensities of the Raman signal related to diamond at 1332 cm−1 are reduced in correlation with the grain size reduction from MCD to UNCD films [Citation18]. The Raman spectra of UNCD films grown with 9.8% of H2 show the maximum G-band near ∼1580 cm−1 with more C-C sp2 chemical bonds due to larger grain boundaries network. In addition, as the gas flow increase, during film growth, the Raman peak related to the G-band moves to ∼1548 cm−1 (see , middle for NCD-) with more C-C sp2 chemical bonds and increase in the Raman signal of T-PA molecules correlated with ∼1164 of C and ∼1489 cm−1. For NCD- mid film with major H2 gas flow concentration, during the film growth, the diamond peak at ∼1332 cm−1 can still be seen, but with very low intensity (, top). The NCD-mid with 73.9% of H2 and NCD- with 48% of H2 films show increased Raman signal correlated with T-PA molecules and C-C sp2 chemical bonds in grain boundaries [Citation24–26].
Figure 2. Raman spectra of diamond films: (a) MCD (grain sizes 1–1.2 µm) and NCD+ (grain sizes: 200–400 nm); (b) NCD-mid (grown with 73.9% H2/grain sizes: 20–60 nm), NCD- (grown with 49% H2/grain sizes: 10–20 nm), and UNCD (grown with 9.8% H2/grain sizes: 6–8 nm) films (see for grain size information). All spectra were obtained by shining a laser beam with wavelength λ = 535 nm on the surface of the films; the peak at 1332 cm −1 is the fingerprint for diamond, which can be seen with visible laser wavelength λ = 532 nm only for NCD+, NCD-mid, and MCD films; NCD- and UNCD films do not reveal the 1332 cm−1 peak with laser beam of λ = 532 nm because of the extreme small grains (UV laser (λ = 244 nm) is necessary to reveal the diamond peak).
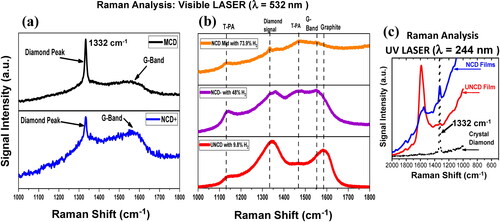
shows SEM images of the surface morphology of MCD, NCD+, NCD-mid (grown with 73.5% H2 (75 sccm)), NCD- (grown with 49% H2 (50 sccm)), and UNCD (grown with 9.8% H2 (25 sccm)) films (see for detailed gas flow conditions, including flows for CH4 and Ar gases). The surfaces of MCD and NCD + films exhibit high and medium surface roughness (, respectively). The surfaces of the NCD-mid and NCD- films exhibit smoother surfaces (, respectively). The surface of the UNCD films is the smoothest, due to the extremely small grain sizes (3–10 nm) (). The thickness of the different diamond films was measured by cross-section SEM (see inserts in , and thickness values listed in and plotted in .
Figure 3. SEM images: (a) MCD film grown for 8 h (H2-200 sccm/CH4-3 sccm); (b) NCD + film grown for 4 h (H2-200 sccm/CH4-3 sccm); (c) NCD-mid film grown for 2 h (H2-72 sccm/CH4-2 sccm/Ar-25 sccm) (see gas flows in ); (d) NCD-film grown for 2 h (H2-50 sccm/CH4-2 sccm/Ar-50 sccm (see gas flows in ); (e) UNCD film grown for 2 h (H2-25 sccm/CH4-2 sccm/Ar-75 sccm (see gas flows in ); (f) grain size (Y axis) vs H2 flows (X axis) and film growth times (grain size (nm) in black points and line and film thickness (nm) in blue points and line).
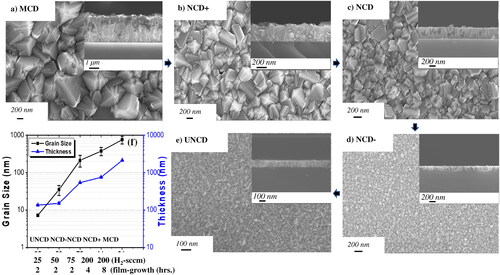
3.2. Measurement of cell proliferation via MTT analysis and cell adherence observation via optical microscopy on diamond surfaces and control Si surface
Investigation of cell viability and proliferation on MCD, NCD, and UNCD films were determined using the MTT assay. Optical microscopy provided valuable information to confirm the growth of A549 cells surfaces and morphology on the diamond surfaces after 48 h (). The optical images of cell growth on the MCD, NCD+, NCD-mid, NCD-, and UNCD films showed good cell attachment and proliferation, with morphological normal elongated shape cells and formation of cells’ cumulus. The roughness of diamond films observed by SEM in apparently does not induce differences correlated with affinity between A549 cells and diamond films. The Si surface shows only small remains of cells with no proliferation ().
Figure 4. Optical microscopy images of A549 cells on (a) MCD film (grown with H2 200 sccm-8 h), (b) NCD + film (grown with H2 200 sccm-4 h), (c) NCD mid film (grown with H2 75 sccm-2 h), (d) NCD- film (grown with H2 50 sccm-2 h), (e) UNCD film (grown with H2 25 sccm-2 h), and (f) Si surface.
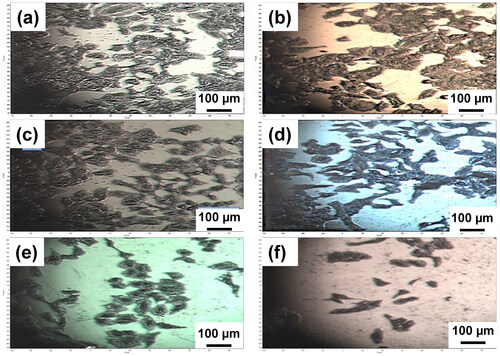
The total numbers of cells were counted after 48 h. The results of MTT assay are shown in . Cell culture on NCD– film, grown with 49% of H2, exhibits the highest percent of cell proliferation on 2.25 cm2. shows that MCD, NCD, and UNCD films exhibit similar high cell growth and proliferation ∼10x higher than for Si surfaces. The quantitative data shown in indicate that diamond films provide excellent scaffolds for growing human cells and eventually make them differentiate into other human cells, via electrical excitation, as the Auciello group is now demonstrating in new experiments, to be reported in a future article. The hypothesis to explain the excellent biological performance of diamond surfaces is that the diamond films used for scaffolds are made of C atoms with unique chemical bonds and tailored surface chemical termination, enabling biological cells to recognize C atoms on the surface of the diamond films as C atoms in cells. The results shown in can be compared positively with the results from other groups on interactions of cells with nanodiamond particles with dimensions (3–5 nm) [Citation27, Citation28] identical to the grain size of UNCD films [Citation6–8].
Figure 5. Proliferation of A549 cells determined by MTT assay after 48 h. Data shown are mean values ± SD of three independent experiments, each done in triplicate. Different columns show differences in cell proliferation for the MCD, NCD (with different gran sizes), UNCD and Si surfaces, showing the superior cell proliferation on the diamond surfaces.
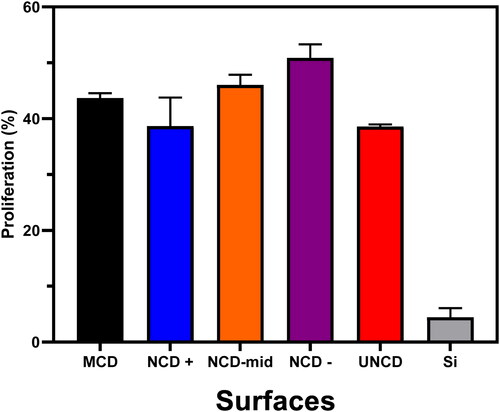
shows the values of cell concentration after 48 h measurements in a Neubauer chamber with the optical microscope. The surfaces of MCD, NCD, and UNCD films were cultured with the cell density of 1x105 cells/ml. The MCD and NCD + surfaces show higher cells concentration with a rate growth of 3125 cells/hours and 2,760.41 cells/hour, respectively, as indicated by mean values from three independent assays. The NCD-mid, NCD-, and UNCD surfaces show similar cell proliferation rates (1,041.66 cells/hour, 1,770.83 cells/hour, and 1,875 cells/hour, respectively). The Si surface shows a negative result associated to death cells with a rate death of 364.58 cell/hr.
4. Conclusions
Key conclusions, based on the fundamental and applied materials and biological related science described in this article, are
Microcrystalline diamond (MCD- grains sizes ≥ 1 µm), nanocrystalline diamond (NCD- grains sizes 20–100s nm), and ultrananocrystalline diamond (UNCD-grain sizes 3–8 nm) films can be grown with very good chemical and biocompatible properties, using the HFCVD technique, to provide scaffolds for investigating the growth and proliferation of A549 cells, demonstrating the feasibility of using the polycrystalline diamond surfaces for studies of other biological cells’ growth.
The H2 flow during the growth of UNCD to NCD films appears to affect the presence of trans-polyacetylene (T-PA) molecules in the grain boundaries, as revealed by the Raman signal correlated with T-PA molecules. However, the T-PA molecules’ concentration appear not to make a substantial difference in the proliferation of cells on the surface of all MCD, NCD, and UNCD films, as shown in .
The MTT assay, using A549 cells, showed very small difference in the cell growth and proliferation on the surfaces of the MCD, NCD, and UNCD films, and all showed about 10x higher cell proliferation than Si surfaces, which may be explained by the fact that the diamond surface provides C atoms with sp3-diamond bonds and chemical surface termination enabling superior cells growth and proliferation.
The negative results about the cell growth on the Si surface support the results obtained in the measurements by the MTT assay and counts in the Neubauer chamber, because the A549 cells’ counts, corresponding to the top surface of diamond films, are not affected by the Si substrate underneath the diamond films.
The surface roughness and topography exhibit some correlation with the cell proliferation and rate growth. MCD and NCD films show major rate growth cell.
Work in progress will provide more valuable information towards exploring growing embryonic stem cells and induced differentiation towards other human cells, via electrical excitation.
Acknowledgments
J.A. Montes-Gutiérrez with CVU 387879 acknowledges support for Postdoctoral fellowship 2021 from CONACyT, Rubio Pharma y Asociados S.A de C.V., Núcleo de Investigación Científica y de Desarrollo Tecnológico for the use of the facilities (cell growth labs) and professional and Dr. Roberto Carrillo Torres personal technical of Raman Spectrometer from Universidad de Sonora for collaborate in this project.
O. Auciello acknowledges support from the University of Texas at Dallas through his Distinguished Endowed Chair Professor position.
J.J. Alcantar-Peña acknowledges the support of CONACYT-FORDECyT through the project FORDECYT-297497.
O.E. Contreras-López acknowledges support of CONACYT-FORDECyT through the project FORDECYT-272894.
Disclosure statement
No potential conflict of interest was reported by the authors.
References
- Saini M, Singh Y, Arora P, et al. Implant biomaterials: a comprehensive review. WJCC. 2015;3(1):52. doi:10.12998/wjcc.v3.i1.52.
- López-Chávez G, editor, Auciello O, Main Collaborator. Implant dentistry/regenerative oral surgery. Ciudad de México, México: Cols Publisher; 2020.
- Kang K, Auciello O, Olmedo DG, et al. Ch. 5: Science and technology of ultrananocrystalline diamond (UNCDTM) Coatings for new generation of implantable prostheses: dental implants, artificial hips and knees, ultrananocrystalline diamond coatings for new generation high-tech and medical devices, O. Auciello, editor. New York, NY, USA: Cambridge Publisher; 2022. p. 141.
- Manivasagam G, Dhinasekaran D, Rajamanickam A. Biomedical implants: corrosion and its prevention – a review. Recent Pat Corros Sci. 2010;2(1):40–54.
- Tasat DR, Bruno ME, Domingo M, et al. Biokinetics and tissue response to ultrananocrystalline diamond nanoparticles employed as coating for biomedical devices. J Biomed Mater Res. 2017;105(8):2408–2415. doi:10.1002/jbm.b.33777.
- Auciello O, Sumant AV. Status review of the science and technology of ultrananocrystalline diamond (UNCD™) films and application to multifunctional devices (invited). Diamond Relat Mater. 2010;19(7–9):699–718.
- Naguib N, Birrell J, Elam J, Carllisle JA, Auciello O. A method to grow carbon thin films consisting entirely of diamond grains 3–5 nm in size and high-energy grain boundaries. US Patent #7. 2006;128:8893.
- Auciello O, editor. Ultrananocrystalline diamond coatings for new generation high-tech and medical devices. New York, NY, USA: Cambridge Publisher; 2022.
- Butler JE, Sumant AV. The CVD of nanodiamond materials. Chem Vap Depos. 2008;14(7–8):145–160.
- Williams OA. Nanocrystalline diamond. Diamond Related Materials. 2011;20(5–6):621–640.
- Piazza F, Morell G. Synthesis of diamond at sub 300 °C substrate temperature. Diamond Relat Mater. 2007;16(11):1950–1957.
- Fuentes-Fernandez EMA, Alcantar-Peña JJ, Lee G, et al. Synthesis and characterization of microcrystalline diamond to ultrananocrystalline diamond films via hot filament chemical vapor deposition for scaling to large area applications. Thin Solid Films. 2016;603:62–68.
- Kalish R. The search for donors in diamond. Diamond Relat Mater. 2001;10(9–10):1749–1755.
- Prelas M, Popovici G, Bigelow LK. Handbook of industrial diamonds and diamond films. Usp Fiziol Nauk. 1997;41(10);1149.
- Advanced Diamond Technologies (Company founded by Auciello and colleagues (2003, profitable 2014, sold to large company 2019), www.thindiamond.com. 2019.
- Auciello O, Lee G. Science and technology of integrated multifunctional piezoelectric oxides/ultrananocrystalline diamond (UNCDTM) films for a new generation of biomedical MEMS energy generation, drug delivery and sensor devices, in book ultrananocrystalline diamond coatings for new generation High-Tech and medical devices, Auciello O, editor. New York, NY, USA: Cambridge Publisher; 2022. p. 237.
- Montaño-Figueroa AG, Alcantar-Peña JJ, Tirado P, et al. Tailoring of polycrystalline diamond surfaces from hydrophilic to superhydrophobic via synergistic chemical plus micro-structuring processes. Carbon. 2018;139:361–368.
- Denisenko A, Aleksov A, Pribil A, et al. Hypothesis on the conductivity mechanism in hydrogen terminated diamond films. Diamond Relat Mater. 2000;9(3–6):1138–1142.
- Sasaki Y, Osanai H, Ohtani Y, et al. Influence of hydrogen gas flow ratio on the properties of silicon-and nitrogen-doped diamond-like carbon films by plasma-enhanced chemical vapor deposition. Diamond Relat Mater. 2022;123:108878.
- Wade T, Geis MW, Fedynyshyn TH, et al. Effect of surface roughness and H–termination chemistry on diamond’s semiconducting surface conductance. Diamond Relat Mater. 2017;76:79–85.
- Budil J, Lišková PM, Artemenko A, et al. Anti-adhesive properties of nanocrystalline diamond films against Escherichia coli bacterium: influence of surface termination and cultivation medium. Diamond Relat Mater. 2018;83:87–93.
- Garguilo JM, Davis BA, Buddie M, et al. Fibrinogen adsorption onto microwave plasma chemical vapor deposited diamond films. Diamond Relat Mater. 2004;13(4–8):595–599.
- Dychalska A, Popielarski P, Franków W, et al. Study of CVD diamond layers with amorphous carbon admixture by Raman scattering spectroscopy. Mater Sci-Pol. 2015;33(4):799–805.
- Montes-Gutiérrez JA, Alcantar-Peña JJ, de Obaldia E, et al. Afterglow, thermoluminescence and optically stimulated luminescence characterization of micro-, nano- and ultrananocrystalline diamond films grown on silicon by HFCVD. Diamond Relat Mater. 2018;85:117–124. doi:10.18502/keg.v3i1.144121.
- de Obaldia E, Tirado P, Alcantar-Peña JJ, et al. Photoluminescence in raman scattering: Effects of HfO2 template layer on ultrananocrystalline diamond (UNCD) films grown on stainless steel substrates. KEG. 2018;3(1):263–272.
- Adamopoulos G, Robertson J, Morrison N, et al. Hydrogen content estimation of hydrogenated amorphous carbon by visible raman spectroscopy. J Appl Phys. 2004;96(11):6348–6352.
- Hemelaar SR, Saspaanithy B, L’Hommelet SR, et al. The response of HeLa cells to fluorescent nanodiamond uptake. Sensors. 2018;18(2):355.
- Ong SY, Van Harmelen RJJ, Norouzi N, et al. Interaction of nanodiamonds with bacteria. Nanoscale. 2018;10(36):17117–17124.