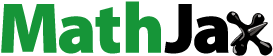
ABSTRACT
Eradication measures are used when a pest or pathogen invades a free area, the objective being to control its outbreak by eliminating or reducing its population levels in that area, which involves removing all infected plants. Xylella fastidiosa is one of the most notorious plant pathogens presently emerging in Europe. This bacterium is a pathogen of concern for many plants globally, and has a broad range of wild and cultivated hosts common in Europe. Given that there is no effective treatment to reduce the repercussions of this disease, its spread poses a severe risk to European agriculture. The EU has designed mandatory plant health measures for the purposes of implementing an eradication or containment strategy. Since an eradication strategy depends on the actions of farmers, it is vital to adopt measures that encourage behavior that helps reduce the risk of spread. In this paper, we have developed a field-scale economic analysis, which links production costs, revenue, agronomic factors, and mandatory protection measures, and allows us to estimate the losses caused over time by pests or pathogens at the farm level, and the age-dependent payments that farmers should receive. This way a confidence interval for fair compensation can be obtained. This compensation serves to design positive incentives for farmers to inform government authorities about the presence of bacteria on their farms and participate in eradication programmes. We apply our economic modelling to Xylella fastidiosa in vineyards, and on olive and citrus plants, in the Valencian Community of Spain in Europe.
1. Introduction
Invasive alien species have been recognized as a major environmental threat because of the significant adverse effects on the environment, in many cases modifying the existing ecological balance (Kropf et al., Citation2020; Simberloff et al., Citation2013; Finnoff et al., Citation2010). Some are crop pests and diseases, which, once they have invaded certain areas, are one of the main factors that can limit farm performance, causing a considerable negative impact on yield, fruit quality and production costs. Damage can be severe and may involve expensive control measures, constraining and conditioning the viability of certain crops, and forcing their substitution, and the search for other alternative productive crops. Particularly in some areas, small-scale growers with limited financial liability cannot afford to invest in more intensive and costly pest and disease management strategies and, thus, are disproportionately affected by these issues. Additionally, crop damage may increase poverty and food insecurity (Atallah et al., Citation2018).
Although most growing areas of the world are free from pests and plant diseases that affect cultivated species, there is a permanent risk that they could become contaminated by these diseases and their vectors. In addition to this risk, the changing climate and the increasing global nature of trade can make these areas more exposed and vulnerable. For this reason, pest and disease control has been one of the main priority measures implemented by national agricultural authorities for many years now. It has also become a popular area of research, leading to studies that can help develop policies and treatments for combating these pests and diseases (Bucci, Citation2018; Finnoff et al., Citation2016).
Control strategies vary depending on the plague and the crop. However, all the current control methods are based on the following basic phytopathological principles of action: exclusion, eradication, avoidance, resistance and therapy (Finnoff et al., Citation2010; Ash, Citation2003; Dominguez García-Tejero, Citation1998). There are relatively few treatments for certain plant pests or pathogens, and in some cases, there are no therapies that can restore the plant’s health once it has become infected. That is why prevention measures in certain places, such as airports, ports and roads, are essential to keep a country free from vectors and plant diseases. Furthermore, when pests or pathogens invade areas free from disease, reactive strategies tend to focus on controlling the outbreak and minimizing its impact (Macpherson et al., Citation2017; Simberloff et al., Citation2013). Eradication measures include removing the infested plants to reduce the pathogen or insect population in the affected area, usually once the disease has been detected. In this sense, early detection is recognized as an effective strategy for reducing these negative impacts. These measures aim to remove the invasive pest before its population reaches a size that is too difficult to be eradicated (Yemshanov et al., Citation2019). Efforts to manage the prevention, control and eradication of pathogen invasions often amount to a substantial expenditure for society (Diagne et al., Citation2020). Kevin et al. (Citation2020) consider that their removal results in social benefits and can be viewed as a public good, suggesting that the costs of eradication warrant compensation from authorities.
Among the organisms causing infectious plant diseases are the bacterial plant pathogens. Some of these bacteria are host-specific, while others can infect a broad range of hosts. This is the case of Xylella fastidious, or Xylella fastidiosa (Panel on Plant Health (European Food Safety Authority-EFSA) Citation2015)Footnote1; (Janse, Citation2012), a worldwide pathogen of concern for many plants. It can be hosted by many plant species, such as citrus, olive, grapevine and stone fruit plants, as well as affecting certain other plants, both ornamental and wild. Unfortunately, in a few cases out of almost 600 potential hosts, there are no treatments to suppress disease development in infected plants. As a result, in these cases, this plant pathogen kills trees and plants and hinders their growth, leading to an enormous economic impact. In fact, it is responsible for various plant diseases, including Pierce’s disease, phoney peach disease, citrus variegated chlorosis, olive quick decline syndrome, almond leaf scorch and various other leaf scorch diseases (Jeger et al., Citation2018). Additionally, X. fastidiosa infects a wide range of host plant species, most of them asymptomatically. On the American continent, it is native to Central America, and widespread over a large part of the Americas. In the United States and worldwide, X. fastidiosa infects hundreds of plant species and has also been the cause of several devastating epidemics (Burbank & Ortega, Citation2018; Sicard et al., Citation2018; Zhang et al., Citation2015). Xylella fastidiosa is regulated in the EU as quarantine pest under Regulation (EU) 2016/2031 on protective measures against plant pests. In the EU, it is expected that it will become a serious threat to citrus production, and olive, grapevine and other perennial fruit crops, such as those included in the genus Prunus (almonds, peaches, plums, apricots and cherries). These crops are the basis of Mediterranean agriculture, and their overall production in Greece, Italy, Spain and Portugal represented around 14% of the EU-27 crop production in 2019.
The PLH (Panel on Plant Health (European Food Safety Authority-EFSA) Citation2015) has not been able to accurately assess the extent of the negative impacts as yet but has concluded that crops and regions which have the conditions for the pathogen to spread, like those found within the Mediterranean agricultural system, can experience severe adverse impacts if control strategies are not implemented. Its presence has been reported in southern Apulia in Italy, on the island of Corsica, in the region of Provence-Alpes-Côte d’Azur in France, in some areas of the north, centre and south of Portugal, as well as in the autonomous region of Madrid, the province of Alicante and the Balearic Islands in Spain. The reported status is “transient, under eradication”, except for the Balearic Islands, Corsica and southern Apulia, where the status is “present with a restricted distribution, under containment” (Jeger et al., Citation2018).
Biosecurity belongs to the public sphere. Indeed, the control of pathogen spread in the EU is regulated by Council Directive 2000/29/EC and through emergency measures by regulations that are continually under review and updated. Currently, Commission Implementing Regulation (EU) 2019/2072, Commission Implementing Regulation (EU) 2020/1201 and its partial modification by Commission Implementing Regulation (EU) 2021/1688 establish the measures to be adopted by member countries to eradicate the plague. If eradication measures, as described in the Commission Implementing Regulation (EU) 2020/1201, are to be effective, they should be applied to any plants that show symptoms, in addition to asymptomatic plants found to be infected through sensitive laboratory tests, neighbouring plants, and all host plants of X. fastidiosa. Eradication thus means removing all infected plants, and governments and phytosanitary institutions at the regional level are responsible for taking contention and eradication measures.
The economic impacts of pest and pathogen invasions depend on ecological and agronomic factors, like the type of host (whether the host plant range is extensive or not); the growth stage of the plant (in some cases, the damage depends on the plant’s growth stage); planting density (that can speed up the spread); the establishment of monoculture areas (climatic factors, like temperature, rain, relative humidity and wind, are determinant in the geographical distribution of crops and condition which areas are more suitable for growing certain crops, often resulting in monoculture farming); or intensive or super-intensive farming systems. All of the afore-mentioned could influence the development and growth of crops, making them more or less vulnerable to pathogens. When assessing any damage, uncertainty makes it difficult to find the optimal choice of pest control measures (Feusthuber et al., Citation2017). Economic modelling offers information on appropriately allocating resources across invasion management activities – including prevention, surveillance programmes for early detection and management, and controlling the populations and spread of invasive species – to minimize long-term costs and damages (Epanchin-Niell, Citation2017). In general, there is considered to be a lack of relevant data on outcomes associated with different measures, which hinders their effective management and mitigation (Diagne et al., Citation2020).
The recent arrival of X. fastidiosa in Europe has made it imperative to implement disease control and management policies to protect European agricultural systems. These policies are designed by applying economic principles to estimate the costs of the damage caused by pests and pathogens, considering both ecological and epistemological factors. Economic measures may be used to reduce the pressure on the ecological system so that it can continue to work within the expected range of environmental conditions (Perrings, Citation2001).
Eradication is used after introduction, in the first stage of the pathogen’s arrival in an area, in order to limit its growth and eliminate it. It consists of removing several plants simultaneously to halt the spread of the disease. Early detection leads to a greater chance of eradication and a reduction in damage and loss (Perrings, Citation2001). This highlights the need for growers to be vigilant and promptly report possible infections to the national plant-protection organizations (Kevin et al., Citation2020). The control of the pathogen spread depends, in part, on human behaviour, and thus an essential step is to adopt both prevention measures to regulate it. These measures may well imply penalties to deter any behaviour that increases the risk of invasion. However, they can also include positive incentives to encourage behaviour that reduces those risks (Perrings, Citation2001; Ziwei et al., Citation2021). The existence of infected plants creates a negative externality for the rest of the plants, and the need to remove them implies greater expense for the farmer. The incentives have to be enough to align the private interest of the farmer with the general interest and encourage optimum decision-making from a social point of view (Hennessy & Wolf, Citation2018; Doménech Pascual Citation2011; Rat-Aspert & Fourichon, Citation2010).
Monetary compensation for the removal of infected plants encourages pest eradication. The estimation of this compensation is crucial to ensure farmers’ collaboration in identifying and removing the affected plants.
The Valencian Community, a region in the Spanish Mediterranean, has crops susceptible to this disease covering almost 70% of its agricultural land. Citrus fruits stand out with nearly 160,000 hectares, approximately 25% of the total agricultural land in the Valencian Community (VC). Meanwhile, non-citrus fruit plants, including apricot, cherry, plum, nectarine, peach plants, etc., together with nut plants, cover 20%. Olive groves also stand out, with roughly 15%, and vineyards for wine production, with 9%. Therefore, the invasion of this pathogen could cause significant economic damage to the VC.
X. fastidiosa was detected in the Valencian Community (Spain) in 2018. Eradication measures were taken quickly following the EU biosecurity policy. Officials were faced with the issue of assessing the losses in monetary terms in order to establish a compensation system for farmers whose crops were affected by the eradication measures. A key issue is to obtain a fair estimation of losses adjusted to the actual situation of the affected farms since positive incentives would encourage farmers to report the presence of the bacteria in their crops to government authorities and participate in eradication programmes. On the contrary, any undervaluation of losses can discourage them from informing the authorities and stop them from adopting eradication measures since they would rather keep the affected plants than replace them. Also, it might lead to the pest spreading quickly with disastrous consequences for plants, which can cause significant economic losses. In this regard, authorities need to guarantee the proper use of public resources when facing a trade-off between undervaluation and overvaluation. Economic analyses can help align the decisions made by public and private decision-makers to achieve socially desirable outcomes. As in the first stage of the invasion, private decision-makers, that is the farmers, tend to under-control invasion relative to socially optimal levels because they generally account for their own costs and benefits of control (Epanchin-Niell, Citation2017).
In this paper, we have developed a field-scale economic analysis that links production costs, revenue, ecological and agronomic factors, and mandatory protection measures to estimate the losses caused over time by X. fastidiosa. The model takes into account that this bacterium can infect several plant species present in Europe.
The main assumption in our research work is that economic damage or losses caused by plagues or infections can be estimated by comparing the value of the agricultural plantations before and after they have been infested or infected. We develop a model that calculates the difference between their present values in both scenarios and apply it to several widespread crops in the Mediterranean agricultural system in southern Europe. For each crop, we have determined the most likely intervals for the variables of several parameters related to plant growth stages and farming systems. The mean distribution of the losses is obtained using simulation techniques, more specifically, the bootstrap method.
The model has broad applicability, and the results may be indicative of possible losses in other situations. We apply the proposed economic model in the Valencian Community.
This study makes two distinct contributions. First, motivated by the gaps in the literature in the field of agricultural economics, we develop an incentive system model for pest eradication to reduce the risk of pest spreading. Our second contribution is an empirical application that shows how the theoretical model can be parameterized to obtain a distribution of the average losses according to the crop, plant’s age and farming system. This empirical application in our paper seeks to define an appropriate incentive system for supporting the eradication of X. fastidiosa in Europe.
2. Materials and methods
There are few estimation methods for controlling the damages or costs caused by pests and pathogens in agriculture, forestry or fisheries. The InvaCost project, started in 2014, is considered the most up-to-date, comprehensive, harmonized and robust global-scale data compilation and description of economic cost estimates associated with invasive species. It works with a standardization protocol to extract data from different sources to compile and compare costs across a multitude of descriptors (costs of a given invasive species, in a given region or a given activity sector, for example), and as a result, estimates the global cost of biological invasions (Diagne et al., Citation2020). Therefore, some authors suggest that the social benefits derived from some measures to control the invasion of a pest can be viewed as a public good, and the costs of these measures warrant compensation from authorities (Kevin et al., Citation2020).
At the local or regional level, assessing the economic implications of pest and pathogen invasion requires a specific and appropriate function that describes the changes inflicted by the presence of a pest or pathogen and its economic consequences. To date, a small number of estimations have been carried out on the economic effects of pests and pathogens. Wittwer et al. (Citation2005) applied a dynamic, computable general equilibrium (CGE) approach based on the Monash Multi-Regional Forecasting (MMRF) model to examine the regional and national economic impacts of a Karnal bunt incursion. Hodda & Cook (Citation2009) present a full assessment of the economic impact of a potato cyst nematode spread in Australia, in which they linked the spread, the pest’s population growth rate, the costs of preplant testing, chemical treatments to reduce crop losses, regulations, and certifications for exports, together with the loss of export markets. Kevin et al. (Citation2020) propose an economic model developed to compute impact based on discounted foregone profits and losses. The model projects the economic impacts on Italy, Greece and Spain, as these countries account for around 95% of the European olive oil production. Using different spread models, the authors analyse sites for the establishment and spread of X fastidiosa, and explore the annual rate of dispersal and its economic impact.
However, for the purposes of calculating incentives, we are more interested in assessing the disaggregated impacts to use these estimations to calculate the financial support growers should receive. In this regard, a field-scale economic analysis is needed. A study by Cook et al. (Citation2015) estimated the total cost for farmers who had suffered the effect of the FOC TR4 disease on their banana crops due to a change in producer surplus, which was caused by the negative supply shift when the disease struck the banana crops and prices dropped. More recently, Taylor & Cook (Citation2018) proposed a model calculating the present value of damage caused by Plasmopara viticola by converting yield losses and variable production cost increases into present values. When analysing the loss of healthy animals due to disease transmission, Horan et al. (Citation2018) proposed a model to calculate the loss in value from net benefits, assuming a discount rate of r, to calculate the present value of net benefits (discounted to time T) for the ex-post problem.
Following the work of Horan et al. (Citation2018), we start from an assumption. Let us suppose there is an agricultural area in Europe, with many plants, such as citrus, olive, grapevine and stone fruit (almond, peach, plum) plants, which produce fruits with an economic value. These crops, along with other trees and ornamental plants in that area, are at risk of a potential invasion by X. fastidiosa. We assume for simplicity that the introduction and establishment of the pathogen and the economic damage inflicted by these processes co-occur as a result of an invasion of the pathogen at date T. By dividing the time period into an ex-ante or pre-invasion interval t < T, and an ex-post or post-invasion interval t ≥ T, we can focus on how the invasion affects the crop conditions in the post-invasion management problem. The result for each moment is given by an algorithm based on the net present value (NPV) method (Ziwei et al., Citation2021). The cash flows (CF) are calculated from the sale of the crops (revenue) and the production costs (direct, indirect and crop start-up costs). The total loss of value can be determined as the sum of the cash flows lost because of removing both the infected and the non-infected crops during the demarcation period. We also consider agronomic factors in the model: the crops’ growing cycle, rotation length, age, whether an irrigated or a rainfed system is used, planting density and farming systems (intensive/super-intensive). On the other hand, characteristics of pests or certain ecological aspects of pathogens, such as their transmission, speed of progression, etc., are ignored since they are not necessary for computing the losses and the incentive system.
Farm losses over time caused by X. fastidiosa can be obtained by the difference between the ex-ante pre-invasion value and the post-invasion value:
The ex-ante value can be estimated as the value of the future cash flows considering that there is no infection at time t, i.e. the ex-ante net present value (PV) using r as the discount rate.
The ex-post net present value is estimated as the value of the future cash flows, considering the production system is infected at time T.
Hence, the damage will be given by:
where and
are the cash flows per area unit at time t without infection (ex-ante cash flows) and with infection (ex-post cash flows), respectively.
Because it is too cumbersome to obtain a function of the cash flows per area unit throughout each year, we have chosen to work with annualized revenue and expenditure, assuming time as a discrete variable.
We develop a model to estimate the ex-ante invasion value of each crop where the net present value (PV) in euros per hectare includes the start-up costs and the profit from harvesting.
2.1. Methodology
2.1.1. Economic model
For a given crop, the annual net cash flows (CF), at time t, are provided by the difference between the receipts Ct (product of the harvest price, p [EUR per kg] and the crop’s yield ([kg per hectare] per year), and the annual production costs Pt. The latter includes direct costs, i.e. consumption directly and unequivocally associated with output, such as seeds, bulbs, cuttings, pots, tubs, etc.; and indirect costs, i.e. resources consumed and needed for both general tasks (watering, cleaning, etc.) and particular tasks (e.g. pest treatments) for the maintenance of the various products. Depreciation and opportunity costs are not taken into account. The initial investment or start-up costs (planting cost per hectare) are included in the first cash flow.
In both assessments (ex-ante and ex-post), the present value will depend on the production plan, which determines the future cash flows. After each planting cycle of N years, the assumption is that another planting cycle of the same type will follow. Therefore, for a plantation of age E, the total value of the plot, without damage, and assuming discrete-time and t = 0, is shown in Equationequation [5](5)
(5) :
where N is the crop’s rotation length. The first addend of Equationequation [5](5)
(5) is the value of the plants, while the second is the value of the land according to the production plan that will be implemented after the current production cycle is finished.
If the net present value of the future cash flows of a complete production cycle from planting until the end of life is called PVC (see, Equationequation [6(6)
(6) ]), then the ex – ante PV can be transformed into Equationequation [7]
(7)
(7) :
In order to estimate the ex post value, the uprooting measures included in EU regulations are taken into account (Council Directive 2000/29/EC, Commission Implementing Regulation (EU) 2019/2072, Commission Implementing Regulation (EU) 2020/1201 and its partial modification Commission Implementing Regulation (EU) 2021/1688). These require the establishment of a demarcated area, which shall consist of an infected zone and a buffer zone:
infected zone: a radius of 50 metres around the infected plants;
buffer zone: 2.5 km or 5 km depending on the type of measures, eradication or containment. The buffer zone surrounding the infected zone established for eradication purposes may be reduced to a width of no less than 1 km in specified conditions.
In the infected zone, the following shall be immediately removed: (a) plants known to be infected by X. fastidiosa; (b) plants showing symptoms indicating possible infection by that pest or suspected of being infected by that pest; (c) plants that belong to the same species as that of the infected plant, regardless of their health status; (d) plants of other species than that of the infected plant, which have been found infected in other parts of the demarcated area; (e) specified plants, other than the ones referred to in points (c) and (d), which have not been immediately subjected to sampling and molecular testing and have not been found free from the X. fastidiosa. In addition, it is forbidden to plant any host plants in the infected zones, and authorities must notify the Commission so that the zone in question can be included in the list of demarcated areas. Surveys and monitoring of the infected area are carried out in order to establish whether the specified organism still exists in that area and if it is not detected for four years, then its demarcation may be lifted. The last modifications of the European regulations open up the possibility of authorizing the planting of specified plants in the eradication strategy for species that have not been to be infected after an intense two-year surveillance. The specific eradication plan activated by the Generalitat Valenciana, published in December of 2020, includes these actions described for the purposes of X. fastidiosa eradication.
These control measures imply critical economic losses for farmers due to losses incurred in the harvest of crops, trees or ornamental plants.
For a given plot in a given area, and assuming that no alternative crop can be grown, which would mean that the plot is set aside forcefully for “l” years (demarcation periodFootnote2) with no production allowed until farming activity can be taken up once again, the ex post value would be:
where ExAD are the expenses for uprooting and removing the plants and trees, and ExMantt are the expenses for maintaining the plot without any production during the “l” years, including taxes, weeding and cleaning tasks, etc.
If we assume optimizing behaviour, the farmers’ current crop is the best alternative. Any other crop would offer a lower return and would reduce the compensation. Although it is a strong assumption, the optimizing behaviour would lead to their production plan being postponed instead of changed. To change the production may imply changing the capital structure and acquiring new know-how. Furthermore, the most likely replacement crops are also affected by Xylella. In some cases, depending on the plant, replanting with resistant cultivars may be possible, as Kevin et al. (Citation2020) suggests.
The difference between both PVs is the present value of the damage caused by the pest.
The first addend is the plantation’s value, given by the monetary value of the plants in the plot at the time of infestation.
The second addend gathers the expenses (Ex) for uprooting and removing the plants.
The third addend includes the expenses for maintaining the plot in the demarcated area.
The fourth addend measures the change in the value of the plot because of the change in the production plan. This addend takes a positive value when the remaining useful life of the uprooted plantation (N-E) is longer than the demarcation period (4 years).
Horticultural and fruit production is highly variable because of external factors, such as prices, weather, and pests, as well as internal factors like the location, planting density, soil characteristics, water availability and quality, water price, etc. In order to deal with this variability, simulation techniques can be used within the economic model.
A considerable number of parameters can be used to estimate each crop´s agronomic processes of growth and harvests and their economic consequences for production costs, crop yield, and revenue (see the parameters and their definitions in ). For this study, we have determined the growth cycle of each crop analysed and defined its rotation length, which includes three growth stages: initial, development, and maximum harvesting.
Table 1. Parameter definitions for each crop.
2.1.2. Bootstrapping
Natural systems with high parameter uncertainty and variability are often assessed with stochastic probability models. These are based on random number generators which simulate random events, such as certain ecological processes (Taylor & Cook, Citation2018). If the distribution of a given statistic needs to be obtained, e.g. the mean, then non-parametric techniques like the bootstrap method can be used. Bootstrapping is a data-based simulation tool for statistical inference (Efron, Citation1979; Davison & Hinkley, Citation1997), allowing us to correct for non-normality. Furthermore, bootstrap methods are used when computation issues appear, such as biases, standard errors, and confidence limits (Chernick & LaBudde, Citation2011). This technique has been applied for decision-making purposes in investment project assessments (Cruz, Citation2012) and to estimate the discount rate in firm valuations (Vidal & Ribal, Citation2017; Breuer et al., Citation2014).
Here, we are interested in the confidence intervals of the economic and agronomic parameters in the proposed economic model. We used the bootstrapping of the fundamental parameters or variables with which we calculated the PVdamage that considers the different potential situations of damage for each crop analysed. We took into account economic parameters (receipts C(t); the price of the product at harvest, p (EUR/kg); and the yield of the crop (kg/ha) per year); annual production costs, P(t), including direct and indirect payments, plus expenses related to machinery and labour; the discount rate; and agronomic crop parameters (growth cycle, rotation length, irrigation systems, planting density, and crop yield). We take n multiple samples from each parameter, which is resampled to obtain a bootstrap resample. Using the results of all the bootstrap resample parameters, we first calculate the CF values and then the confidence intervals of the PVdamage. These PV intervals are determined in EUR per hectare and EUR per plant for each crop. It is essential to calculate the value of a plant in the production cycle to be able to calculate the costs to farmers if isolated plants are eradicated. The empirical bootstrap process is shown in .
The bootstrap technique was applied to obtain 10,000 simulations of the average flow value per crop type and age. First, in , each parameter of crop j was bootstrapped 10,000 times, thereby obtaining 10,000 mean values with which to calculate the distribution of the mean value. Next, the average PVdamage of the crop was computed through Equationequation [9](9)
(9) . shows the process applied to each crop.
Figure 2. Process to calculate the PV of damage to an Orange grove.
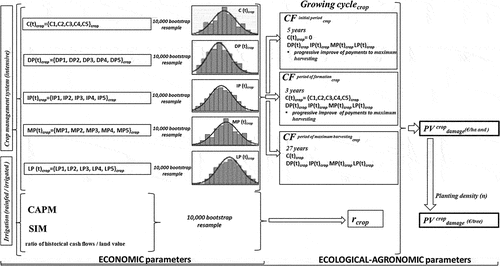
Example of a bootstrap resample for receipt C(t) for crop j:
we have n multiple samples of variable C(t) for crop j:
C (t)j = {C1, C2, C3, C4, … Cn}j
we resample n data to obtain a bootstrap resample for the receipts:
C (t)jR1 = {C1, C2, C2, C3, C4, C1, …, Cn, … }jR1
we compute the mean of this resample and obtain the first bootstrap receipts’ mean: μ1*
Note that there are some duplicates; this is because bootstrap resampling is performed by sampling with replacement from the data. The bootstrap resample size equals the number of observations in the original data set.
In the same way, a second resample for crop j C(t)jR2 can be obtained, and a second bootstrap mean can also be calculated for the receipts: μ2*. This procedure is repeated as many times as desired to obtain a series of bootstrap means, which is an empirical bootstrap distribution (EBD) of the sample mean, in this case, of average receipts. According to Efron and Tibshirani (2007), a high number of bootstrap iterations, as many as 10,000, can produce stable results.
We repeated the bootstrap procedure for all the model variables, i.e. the economic, ecological, and agronomic variables for each crop, thereby obtaining the empirical distribution of the PVjdamage for each crop in EUR per hectare and EUR per plant.
2.2. Empirical validation
Our study used data from the Agriculture and Food Ministry official reports on farm costs and returns (ECREA, 2010, 2011, 2012, 2013, 2014). This report, published every year since 2010, gathers the overall economic results, including the production expenses for the most important crops in the VC.
We used the last five years published (2010-2014) for the Valencian Community (NUTS-2). Another essential data source in this study was the Official Report on the Valencian Agricultural Sector (ISAV), which gathers information and statistics related to the production, yields, land distribution, and input and output prices of the agri-food sector in the VC.
The process shown in was repeated for oranges, rainfed olives, irrigated olives, rainfed grapevines, and irrigated grapevines. All the crop parameters analysed in this study are defined in . Each parameter for each crop j was bootstrapped, applying the process shown in .
The mean results correspond to plants in the stage of maximum yield. Next, we applied the growth cycle and rotation length parameters to these values. As a result, we obtained 10,000 values of CForange per year in the cycle.
The discount rate is a key parameter in any discount model. The discount rate reflects the annual required return, considering each farm as an investment. We have used three different models to compute it ():
A model based on the Capital Asset Pricing Model (CAPM), which is a method used to obtain the required return of financial investments and portfolios, including real estate investments (Galagedera, Citation2007). In this model, it is assumed that investors diversify their investments across multiple assets and industries. The model can provide a unique expected return for investments in farmland.
A similar model to the CAPM, which considers that investors only invest in the farmland market. It uses a farmland market index, termed as SIM (Single Index Model); (Unterschultz & Quagrainie, Citation1996; Ribal et al., Citation2003). The model provides a unique required return for every kind of plantation.
And finally, a model that relates historic farmland cash flows to the farmland value as the annual return of investment (ROI), and consequently, the discount rate can be obtained for each kind of crop.
Table 2. Discount rate.
Using three models permits the consideration of several approaches to the calculation of the farmer’s expected return and moreover, it provides useful information to be used within the bootstrap process.
As the model considers non-limited life and variable cash flows, the discount rate is not a capitalization rate. The CAPM and SIM approaches consider the market behaviour in an extended time horizon to work out the corresponding Beta coefficient and then the expected return. However, the ROI approach is computed on an annual basis and then averaged as a proxy of the average capital return. It might be preferable to work out the ROI as an Internal Rate of Return, but that would imply singling out each farm plot which would not be suitable for a massive valuation case.
Kevin et al. (Citation2020) fix a deterministic 3% for the purposes of discounting the foregone profits and losses caused by Xylella fastidiosa in European olives and a uniform distribution of between 3 and 7% for a sensitivity analysis in the same paper.
Given the complexity of accurately determining the plantation’s age to be able to establish the compensation farmers should receive, our model allows age intervals to be built, thus making the work of field inspectors easier. For this purpose, K-means clustering was carried out, i.e. we clustered the data according to their similarity in damage value (EUR/ha). Specifically, values were grouped into four clusters to allow us to obtain a homogeneous value. It must be stressed, however, that it was not our intention to use this clustering procedure to obtain the homogeneity of the production cycle nor that of the cash flows. The last interval can be useful for plantations that are older than the expected age limit or even for plantations with low yields.
3. Results
Through the model, we were able to generate 10,000 simulations of the damage mean value for each plant type by age. This provided us with an empirical distribution of the damage mean values for each plant type by age. Consequently, we obtained an empirical distribution of net present value (PV) for each species according to the age of the plants. These values are the losses suffered by the producers of these species (citrus, olive, or grapevine), and these amounts can then be translated into economic compensations that the public administration would have to pay farmers.
As can be seen in , compensation is higher for irrigated crops since crop yields are also higher. The highest value of the plantations as regards citrus is in the period from 5 to 18 years, when there is full production and costs stabilize. Thus, a compensation amount of more than EUR 19,392 per hectare is established. In the case of irrigated vineyards, the highest value of the plantations is in the long period from 3 to 33 years, with the compensation amount being slightly higher than EUR 18,500 per hectare. Rainfed vineyards have a much lower compensation in the same period, at only EUR 7,136 per hectare. Similarly, while irrigated olives have a compensation of EUR 13,742 per hectare in the period from 3 to 48 years, in the case of rainfed olives, it is only EUR 6,231 per hectare.
Table 3. Results of the value of crops by age group of the plantation per hectare (EUR/ha).
Although not included in , the compensation for one plant is directly determined by the planting density. For instance, due to the higher planting density of vineyards, the compensation amount for vines is much lower (EUR 5.80 per irrigated grapevine compared to EUR 68.49 per citrus plant).
4. Discussion
The introduction of some plant pests has the potential to cause widespread mortality in both native and cultivated plant populations. Much of the existing research work analyses the expected economic impact from the new introduction of some pests, such as Potato Cyst Nematodes in Australia (Hodda & Cook, Citation2009), Fusarium oxysporum in the banana crop in Australia (Cook et al. (Citation2015), or Xylella fastidiosa in the olive crop in some areas of Europe (Kevin et al., Citation2020), when these spread in dense areas with sensitive crops. Once established, the cost of the mitigation and eradication and the damage and loss incurred by these plant pests often exceed the cost of preventing their introduction. In these studies, economic impact analysis seeks to quantify the post-invasion cost which, in turn, justifies the introduction of prevention measures. In contrast, our research work is centred on the eradication measures to avoid the spread. The results are interesting for the initial control after pest arrival.
Biosecurity strategies are traditionally within the public sphere (de la Cruz, 2020). However, in cases such as the spread of plant disease, close collaboration with the farmers is needed. As explained, eradication measures are aimed at removing the infected plants to avoid pest spreading. European legislation defines the rules and practices whose aim is to control both pests and pathogens (Regulation EU No 652/2014 and 702/2014) and establishes that monetary compensation is a farmers’ right in order to balance prevention and eradication expenses such as the loss of sacrificed animals or removed plants, and even transport expenses, cleaning and disinfection expenses, revenue losses and the need for new plantations.
Eradication compensation systems in plant health are less common than in animal or public health (Mumford, Citation2011). Some models are proposed to estimate incentives or compensation to eradicate livestock diseases (Gilbert & Rushton, Citation2018, Hennessy & Wolf, Citation2018, Rat-Aspert & Fourichon, Citation2010), but what is less common is research that analyses and proposes incentive models to eradicate plant pests or pathogens. The models proposed in both cases are associated with incomes and costs for farmers ex-ante and ex-post the arrival of the disease. In some of the models proposed to control livestock diseases, additional compensation is introduced if the farmer has applied prevention measures, such as vaccination (Rat-Aspert & Fourichon, (Citation2010), or monitoring and control (Mato-Amboage, Pitchford, and Touza 2019).
The incentive systems are established to motivate farmers to inform authorities of the presence of pests and pathogens in their crops (Gilbert & Rushton, Citation2018; Hennessy & Wolf, Citation2018). In this sense, the control of pests and pathogens depends on the behaviour of the farmer. The compensation must be adequate to push growers to promptly report introductions and initiate eradication actions to prevent further dispersal (Hennessy & Wolf, Citation2018). Therefore, to create a system of incentives that motivates farmers to inform authorities of the presence of the pest or pathogen in their crops, it is first essential to obtain a fair estimation of the losses caused by pests and pathogens adjusted according to the actual situation of the affected farms. The calculated compensation has to be sufficient so that each farmer can establish a new production plan that guarantees the survival not only of the farms but also of the agrarian system. On the contrary, any undervaluation of losses would discourage farmers from informing the authorities about the infection. Thus, the authorities should ensure that public resources are used efficiently to avoid facing a trade-off situation between undervaluation and overvaluation. A field-scale economic analysis such as the one we propose is necessary. On the other hand, the existing research makes use of country-wide averages of prices and operational costs per ton of the analysed crops (Kevin et al., Citation2020). However, in our study, we make use of the average prices and operational costs per ha of different types of crops in the Valencian Community (in the case of citrus fruit, we can differentiate between oranges, mandarins and lemons), and different farming systems (rainfed or irrigated/intensive or super-intensive), the growing cycle, the rotation length, age, and planting density, using the available data at finer spatial resolution. So, we adjust the compensation better to the current crop in the field. In this sense, by taking the cropping-system-specific conditions into account, the compensation can encourage the growers to detect any pest or pathogen early and report it promptly.
The definition of an eradication compensation system remains the responsibility of national authorities, based on requirements in international agreements (Mumford, Citation2011). In our case, the Valencian Community has to base the regulations on the national and European regulations.
In the case of animal healthcare, what traditionally happens in Spain is that the Administration sets reference values per affected animal considering the species and age. Following EU rules, the reference value cannot be greater than the market value of the animals, and these reference values are periodically updated. As far as plant health is concerned, most of the compensation systems are for arable crops; the compensation covers the removal of the crop and the harvest value considering the expected yield and market prices. The damage is estimated without considering the effect of any specific protection measure, such as demarcated areas or crop replacement.
The proposed model follows EU measures to fix compensation by considering the demarcation period for the Xylella pest. The assumption is that the existing crop will be replaced by an identical one. If it were replaced by a different one, then the model would have to be adjusted. If alternative crops are allowed, and a new crop can be planted immediately after the uprooting, inactivity will last for one year at the most, hence there are no maintenance costs in the demarcated area (art. 6 Regulation (EU) 2020/1201). The differences in the ex-ante and ex-post value will depend on the chosen alternative crop, so, a vast range of cases can be considered. However, whenever the yields of the chosen alternative crop are higher than those of the existing crop, the value of that alternative crop will also be higher than that of the existing one, and therefore, the compensation value will be lower. To determine the alternative crop, it is imperative to check the existing crops not only in the area affected by X. fastidiosa, but also in the surroundings of the affected farm. The financial viability of the alternative crop should be assessed by means of technical-economic studies, considering not only agronomic aspects but also ensuring that the necessary management, transformation, and marketing structures exist for the products to be introduced into the market. Conversely, if the alternative crop involves significant changes in the production structure of the farm, then additional damage costs should be estimated in order to take into account the new capital investment.
It is of critical importance that any information regarding eradication measures should flow towards the farmers. Farmers’ unions and cooperatives are the primary sources to convey these measures. However, these farmers’ representatives need to be rightly informed about the measures to be taken. Furthermore, representatives and authorities should meet to agree on the compensation value within the framework provided by the model. The model’s introduction of variability and bootstrap confidence intervals makes it easier for the parties to reach an agreement.
The impacts on crops and agricultural enterprises are generally better understood and accounted for than the damage that affects values that are not usually measured in economic terms, such as agrosystem services’ aesthetic or recreation values. Nonetheless, these services are receiving increased attention as, depending on the agrosystem, the farmers may manage landscapes, soil or ethnological heritage of high value. The estimation of potential impacts should, therefore, not only take into account the consequences for crops and agricultural enterprises but also the impacts of pests on the products and services that agrosystems provide to society. Simply put, by understanding exposure, we can obtain an estimation of all the values at risk. Further developments of the model might need to incorporate multiple ecosystem services complementary to crop yields to assess the impact on joint production. Recent work by Ali et al. (Citation2021) provides a first assessment of the broader environmental impacts of X. fastdiosa subsp. pauca in Puglia (Italy), which can serve as a basis for formulating hypotheses for further investigation.
5. Conclusion
This study addresses a relevant policy issue, in that Xylella. fastidiosa is a pathogen of global concern for many plants. Moreover, the spread of this pathogen is a threat to some of the main crops in Europe, which are the basis of the Mediterranean agricultural system. Indeed, the consequences of Xylella fastidiosa invading and spreading in European agricultural holdings could be disastrous because of the high yield losses and other damage it could cause. The different options to reduce the aforementioned risks include strategies that prevent the introduction of pathogens and contain their outbreaks, with control measures that are economically and environmentally costly. If the spread is to be contained, it is imperative to apply drastic measures as soon as possible to eradicate the disease from the infected crop. These measures consist of removing several plants simultaneously to stop the disease from spreading.
The projection of future discounted cash flows enables us to calculate the compensation value for each crop based on easily identifiable variables at the regular cultivation plot level.
The developed model combines production costs, revenue, and agronomic factors to estimate farm-level losses caused by pests and pathogens over time. The main contribution of the model is to offer a systematic estimation of the value of the crop during the production cycle, which allows an estimation to be made of the age-dependent compensation payments to farmers. The compensation calculated must be sufficient for each farmer to establish a new production plan that guarantees the survival of the farms. And this should encourage farmers to report the disease to government authorities and participate in eradication programmes.
It is a field-scale model and it allows officials:
To take into account specific features of each farm by fixing the compensation within the confidence interval
To have a quantitative framework to negotiate with farmers’ representatives
To fix the economic compensation for single plants
To fix the compensation according to the plant’s development stage if the age is unknown.
We apply our theoretical model to find an appropriate incentive regime for eradicating X. fastidiosa in certain crops: orange, olive, and grapevine. To this end, we define the production cycle of each crop, and its rotation length, which is divided into three growth stages: initial, development, and maximum harvesting stage. The regulations to lift the demarcated areas have changed as more knowledge has been acquired about the pest and its biology. However, the model can be adapted to these regulation changes. The model also considers the progressive losses in harvests and, consequently, harvest cash inflows by determining a final senescence period. Governments can use the results to establish the amounts of compensation that should be given to farmers whose crops are affected by the implementation of eradication measures.
Abbreviations employed
CAPM (Capital Asset Pricing Model), CF (Cash Flows), EBD (Empirical Bootstrap Distribution), ECREA (Agriculture and Food Ministry official reports on farm costs and returns), EFSA (European Food Safety Authority), EU (European Union), ISAV (Official Report on the Valencian Agricultural Sector), MMRF (Monash Multi-Regional Forecasting), PLH (Panel on Plant Health), PV (Net Present Value), CGE (Computable General Equilibrium), SIM (Single Index Model), VC (Valencian Community).
Disclosure statement
No potential conflict of interest was reported by the author(s).
Additional information
Funding
Notes
[1] Panel on Plant Health (PLH). European Food Safety Authority (EFSA).
[2] Article 6. Lifting the demarcated areas (COMMISSION IMPLEMENTING REGULATION (EU) 2020/1201). Where the pest has not been detected in a demarcated area for a period of four years, this demarcation may be lifted.
References
- Ali, B. M., van der Werf, W., & Oude Lansink, A. (2021).August 2020 Assessment of the environmental impacts of Xylella Fastidiosa Subsp. Pauca in Puglia. Crop Protection, 142, 105519. https://doi.org/10.1016/j.cropro.2020.105519
- Almeida, R.P.P., & Nunney, L. (2015). “How do plant diseases caused by Xylella fastidiosa emerge?“ Plant Disease, 11(99), 1457–1467. https://doi.org/10.1094/PDIS-02-15-0159-FE
- Ash, G. (2003). Pest and disease management handbook. In J. Pratley, & Charles Sturt University (Ed.), Principles of field crop Production (pp. 378–417). Oxford University Press.
- Atallah, S. S., Gómez, M. I., & Jaramillo, J. (2018). A bioeconomic model of ecosystem services provision: Coffee berry borer and shade-grown Coffee in Colombia. Ecological Economics, 144, 129–138. https://doi.org/10.1016/j.ecolecon.2017.08.002
- Breuer, W., Fuchs, D., & Mark, K. (2014). Estimating cost of capital in firm valuations with arithmetic or geometric mean–or better use the Cooper estimator? The European Journal of Finance, 20(6), 568–594.
- Bucci, E. M. (2018). Xylella Fastidiosa, a new plant pathogen that threatens global farming: Ecology, molecular biology, search for remedies. Biochemical and Biophysical Research Communications, 502(2), 173–182. https://doi.org/10.1016/j.bbrc.2018.05.073
- Burbank, L. P., & Ortega, B. C. (2018). Novel amplification targets for rapid detection and differentiation of Xylella Fastidiosa subspecies fastidiosa and multiplex in plant and insect tissues. Journal of Microbiological Methods, 155(November), 8–18. https://doi.org/10.1016/j.mimet.2018.11.002
- Cook, D. C., Taylor, A. S., Meldrum, R. A., & Drenth, A. (2015). Potential economic impact of Panama disease (tropical race 4) on the Australian banana industry. Journal of Plant Diseases and Protection, 122(5), 229–237.
- Cruz Aranda, F. (2012). Procesos estocásticos en la valuación de proyectos de inversión, opciones reales, árboles binomiales, simulación bootstrap y simulación Monte Carlo: Flexibilidad en la toma de decisiones. ContaduríA Y administración, 57(2), 83–112.
- Davison, A. C., & Hinkley, D. V. (1997). Bootstrap methods and their application (No. 1). Cambridge university press.
- Diagne, C., Leroy, B., Gozlan, R. E., Vaissière, A. C., Assailly, C., Nuninger, L., Roiz, D., Jourdain, F., Jarić, I., & Courchamp, F. (2020). InvaCost, a Public Database of the Economic Costs of Biological Invasions Worldwide. Scientific Data, 7(1), 1–12. https://doi.org/10.1038/s41597-020-00586-z
- Doménech Pascual, G. (2011). Justificación de las indemnizaciones por sacrificios impuestos en la lucha contra epizootias y plagas InDret: Revista para el Análisis del Derecho, 4, 7–34. https://www.academia.edu/9433398/Justificacion_de_las_indemnizaciones_por_los_sacrificios_impuestos_en_la_lucha_contra_epizootias_y_plagas?from=cover_page
- Dominguez García-Tejero, F. (1998). Plagas y Enfermedades de Las Plantas Cultivadas 9a. edited by S. A. M.-P. LIBROS. S.A. MUNDI-PRENSA LIBROS.
- Efron, B. (1979). Bootstrap Methods: Another Look at the Jackknife. Ann. Statist, 7(1), 1–26. doi:10.1214/aos/1176344552.
- Epanchin-Niell, R. S. (2017). Economics of invasive species policy and management. Biological Invasions, 19(11), 3333–3354. https://doi.org/10.1007/s10530-017-1406-4
- Feusthuber, E., Mitter, H., Schönhart, M., & Schmid, E. (2017). Integrated modelling of efficient crop management strategies in response to economic damage potentials of the western corn rootworm in Austria. Agricultural Systems, 157, 93–106. https://doi.org/10.1016/j.agsy.2017.07.011
- Finnoff, D., Horan, R. D., Shogren, J. F., Reeling, C., & Berry, K. (2016). Natural vs anthropogenic risk reduction: Facing invasion risks involving multi-stable outcomes. Journal of Economic Behavior & Organization, 132(Part B), 113–123. https://doi.org/10.1016/j.jebo.2016.05.015
- Finnoff, D., Macintosh, C., Shogren, J. F., Sims, C., & Warziniack, T. (2010). Invasive species and endogenous risk. Annual Review of Resource Economics, 2, 77–100. https://doi.org/10.1146/annurev.resource.050708.144212
- Galagedera, D. U. A., & Galagedera, D. U. A. (2007). A Review of Capital Asset Pricing Models. Managerial Finance, 33, 821–832. https://doi.org/10.1108/03074350710779269
- Gilbert, W., & Rushton, J. (2018). Incentive perception in livestock disease control. Journal of Agricultural Economics, 69(1), 243–261. https://doi.org/10.1111/1477-9552.12168
- Hennessy, D. A., & Wolf, C. A. (2018). Asymmetric information, externalities and incentives in animal disease prevention and control. Journal of Agricultural Economics, 69(1), 226–242. https://doi.org/10.1111/1477-9552.12113
- Hodda, M., & Cook, D. C. (2009). Economic impact from unrestricted spread of potato Cyst nematodes in Australia. Phytopathology, 99, 1387–1393. https://doi.org/10.1094/PHYTO-99-12-1387
- Horan, R. D., Finnoff, D., Berry, K., Reeling, C., & Shogren, J. F. (2018). Managing wildlife faced with pathogen risks involving multi-stable outcomes. Environmental and Resource Economics, 70, 713–730. https://doi.org/10.1007/s10640-018-0227-y
- Janse, J. D. (2012). Bacterial diseases that may or do emerge, with (Possible) economic damage for Europe and the Mediterranean Basin: Notes on epidemiology, risks, prevention and management on first occurrence. Journal of Plant Pathology. http://www.jstor.org/stable/45156305
- Jeger, M., Caffier, D., Candresse, T., Chatzivassiliou, E., Dehnen‐Schmutz, K., Gilioli, G., Grégoire, J., Anton Jaques Miret, J., MacLeod, A., Navajas Navarro, M., Niere, B., Parnell, S., Potting, R., Rafoss, T., Rossi, V., Urek, G., Van Bruggen, A., Wopke Van der, W., West, J., & Bragard, C. (2018). Updated Pest Categorisation of Xylella Fastidiosa. . https://doi.org/10.2903/j.efsa.2018.5357
- Kevin, S., van der Werf, W., Cendoya, M., Mourits, M., Navas-Cortés, J. A., Vicent, A., & Oude Lansink, A. (2020). Impact of Xylella Fastidiosa subspecies Pauca in European olives. Proceedings of the National Academy of Sciences of the United States of America, 117(17), 9250–9259. https://doi.org/10.1073/pnas.1912206117
- Kropf, B., Schmid, E., Schönhart, M., & Mitter, H. (2020). Exploring Farmers’ behavior toward individual and collective measures of Western corn rootworm control – A case study in South-East Austria. Journal of Environmental Management, 264, 110431. https://doi.org/10.1016/j.jenvman.2020.110431
- Mumford, J. D. (2011). Compensation for quarantine breaches in plant health. Journal Fur Verbraucherschutz Und Lebensmittelsicherheit, 6(SUPPL. 1), 49–54. https://doi.org/10.1007/s00003-011-0676-5
- Panel on Plant Health (European Food Safety Authority-EFSA). (2015). Scientific opinion on the risks to plant health posed by Xylella Fastidiosa in the EU Territory, with the identification and evaluation of risk reduction options). https://doi.org/10.2903/j.efsa.2015.3989
- Perrings, C. (2001). The economics of biological invasions. Land Use and Water Resources Research, 1(3), 1–9. https://ageconsearch.umn.edu/record/47851/files/paper01-03.pdf
- Rat-Aspert, O., & Fourichon, C. (2010). Modelling Collective effectiveness of voluntary vaccination with and without Incentives. Preventive Veterinary Medicine, 93(4), 265–275. https://doi.org/10.1016/j.prevetmed.2009.11.004
- Ribal, J., Segura, B., & Guadalajara, N. (2003). Modelos Modificados de Sharpe Para El Mercado de La Tierra En España. Estudios Agrosociales y Pesqueros 199(199), 119–137. https://doi.org/10.22004/ag.econ.184346
- Sicard, A., Zeilinger, A. R., Vanhove, M., Schartel, T. E., Beal, D. J., Daugherty, M. P., & Almeida, R. P. P. (2018). Xylella Fastidiosa : Insights into an emerging plant Pathogen. Annual Review of Phytopathology, 56(1), 181–202. https://doi.org/10.1146/annurev-phyto-080417-045849
- Simberloff, D., Louis Martin, J., Genovesi, P., Maris, V., Wardle, D. A., Aronson, J., Courchamp, F., Galil, B., García-Berthou, E., Pascal, M., Pyšek, P., Sousa, R., Tabacchi, E., & Vilà, M. (2013). Impacts of biological invasions: What’s what and the way forward. Trends in Ecology and Evolution, 28(1), 58–66. https://doi.org/10.1016/j.tree.2012.07.013
- Sun, J., Chernick, M. R., & LaBudde, R. A. (2011). A bootstrap test for comparing two variances: Simulation of size and power in small samples. Journal of Biopharmaceutical Statistics, 21(6), 1079–1093.
- Taylor, A. S., & Cook, D. C. (2018). An Economic assessment of the impact on the Western Australian Viticulture industry from the Incursion of Grapevine Downy Mildew. Journal of Plant Diseases and Protection, 125(4), 397–403. https://doi.org/10.1007/s41348-018-0152-x
- Unterschultz, J. R., & Quagrainie, K. 1996. Investment Analysis of Agri-Food Ventures: What Risk Premia are Appropriate? The Silence of the Literature. Ssrn Journal. https://doi.org/10.2139/ssrn.52302
- Vidal, R., & Ribal, J. (2017). Valuation of agrifood SMEs. lessons to be learnt from the stock market. Spanish Journal of Agricultural Research, 15(4), e0118–e0118. https://doi.org/10.5424/sjar/2017154-11668
- Wittwer, G., McKirdy, S., & Wilson, R. (2005). Regional economic impacts of a plant disease incursion using a general equilibrium approach. The Australian Journal of Agricultural and Resource Economics, 49(1), 75–89.
- Yemshanov, D., Haight, R. G., Koch, F. H., Venette, R. C., Swystun, T., Fournier, R. E., Marcotte, M., Chen, Y., & Turgeon, J. J. (2019). Optimizing surveillance strategies for early detection of invasive alien species. Ecological Economics, 162, 87–99. https://doi.org/10.1016/j.ecolecon.2019.04.030
- Zhang, S., Chakrabarty, P. K., Fleites, L. A., Rayside, P. A., Hopkins, D. L., & Gabriel, D. W. (2015). Three new Pierce's disease pathogenicity effectors identified using Xylella fastidiosa biocontrol strain EB92-1. PloS One, 10(7), e0133796.
- Ziwei, L., Zhiming, Q., Jiang, Q., & Sima, N. (2021). An Economic analysis software for evaluating best management practices to mitigate greenhouse gas emissions from Cropland. Agricultural Systems, 186, 102950. doi:10.1016/j.agsy.2020.102950.
- Macpherson, M. F., Kleczkowski, A., Healey, J. R., & Hanley, N. (2017). Payment for multiple forest benefits alters the effect of tree disease on optimal forest rotation length. Ecological Economics, 134, 82–94.