Abstract
This study provides the evaluation of oxygen carrying capacity of the novel perfluorocarbon emulsion (Neo-PFC) produced by the new emulsifying technology named High Pressure Process. For the performance comparison of oxygen carrying abilities of Neo-PFC and a representative PFC emulsion, the oxidation states of cerebral tissues in substituted animals were measured by near-infrared spectrometry. After the 70% exchange transfusion of whole blood of rats by Neo-PFC and Fluosol-DA®, fractional inspired oxygen (FiO2) was gradually decreased from 100% to 0%. As the control experiments, the blood was substituted by Krebs Ringer bicarbonate buffer containing 3% BSA. When the blood of rats was substituted by Neo-PFC, Cyt. ox., a terminal enzyme in mitochondrial respiratory chain maintained fully oxidized state with FiO2 values between 100 to 40%. By contrast, in the models substituted by Fluosol-DA® and BSA-buffer, Cyt. ox. was gradually reduced with FiO2 values below 60% and 80%, respectively. This specific advantage of Neo-PFC was explained by its higher oxygen solubility in arterial blood. The novel PFC emulsion prepared by the new emulsifying technology is a potential basis for blood substitutes.
INTRODUCTION
Perfluorochemical (PFC) emulsion has been developed as candidate for artificial blood substitutes Citation[1-4], and some of them have been clinically applied Citation[5-7]. Generally, PFC emulsion is blood type free, and can be stored longer than native red cells Citation[[4]]. In addition, PFC emulsion has high performance to deliver oxygen in peripheral circulation because of its lower viscosity than native blood Citation[[2]]. The PFC emulsion has been applied for percutaneous transluminal coronary angioplasty (PTCA) Citation[[5]], radiation therapy Citation[[7]], magnetic resonance imaging Citation[[8]], and exchange transfusion in cat cerebral tissues Citation[[9]]. On the other hand, some in vivo studies reported that half-lives of PFC emulsion in animals are shorter than that of native red cell, and particle size of PFC has to be below 1/10 of native red cells for transit through capillary Citation[[1]]. Perfluorodecalin (PFD), a PFC used in Fluosol-DA® has a faster excretion rate than other PFC such as perfluorotripropylamine (FTPA). However, emulsification of PFD to small and stable particle is difficult Citation[[4]]. In addition, Pluonic F-68, a synthesized surfactant of Fluosol-DA® caused the activation of endothelial factor Citation[[10]]. To settle these issues, we employed a new emulsifying technology for improvement of the above-mentioned problems of PFC emulsion. This study provides the evaluation of oxygen carrying capacity of the developing PFC emulsion in substituted animals by near-infrared spectrometry (NIRS).
MATERIALS AND METHODS
PFC Emulsion
The PFC emulsion (Neo-PFC) was prepared with Controlled High Pressure Process Homogenizer Citation[[11]](). Perfluorodecalin and egg yolk lecithin were employed as the PFC material and the surfactant, respectively. For improving stability and pharmacokinetics, the surface of PFC emulsion was modified by DSPE-PEG. Fluosol-DA® was purchased from Green Cross Co. The physicochemical properties of Neo-PFC were shown in in comparison to Fluosol-DA® and native blood.
Figure 1. The concept for High Pressure Process Homogenizer (DeBEE 2000) developed by TalShechter, Nakano, and Fukushima Citation[[6]] employed for PFC emulsification. The first step of PFC emulsification is performed by dual feed method, and the second step by reverse method repeatedly (n = 3−10).
![Figure 1. The concept for High Pressure Process Homogenizer (DeBEE 2000) developed by TalShechter, Nakano, and Fukushima Citation[[6]] employed for PFC emulsification. The first step of PFC emulsification is performed by dual feed method, and the second step by reverse method repeatedly (n = 3−10).](/cms/asset/0e8beac4-c16d-4f5c-b5d5-887f57e89696/ianb19_a_11116784_uf0001_b.gif)
Table 1. The Properties of Neo-PFC, Fluosol-DA®, and Stored Blood
Animal Preparation
For comparing the ability of oxygen delivery to tissues, the three animal models of exchange transfusion were prepared. The first model was rat whose blood was substituted by Neo-PFC emulsified by DeBEE 2000. The second one was that by Fluosol-DA®, and third one was control model that by Krebs-Ringer buffer (pH 7.5). The male Wistar rats (body weight: 230–280 g) anaesthetized with pentobarbital (50 mg/kg, i.p.) were ventilated by 100% O2 during blood substitution. The blood exchange transfusions was carried out by infusion from a femoral vein and drawing from a femoral artery (1.0 ml/min). After the 70% substitution of whole blood, O2-N2 ratio was changed by stepwise decreasing of fractional inspired oxygen (FiO2). The body temperature of the rats was maintained at 37°C. The PO2 in a femoral artery and a cervical vein, blood pressure in femoral artery, and heart rates were frequently measured during experiments.
Measurements of NIRS
For the measurements of oxidation states of cytochrome oxidase (Cyt. ox.), a terminal enzyme in mitochondrial respiratory chain, a spectrophotometer with a rotating disc with four band-pass filters Citation[[12]] was employed. The light source contained four wavelengths (700, 730, 750, and 805 nm), and was guided onto the rat calvaria by the use of an optical fiber. Light transmitted through the cerebral tissues was detected by another optical fiber in the mouth and guided to a photo multiplier. The changes in reduced Cyt. ox. were calculated by the following formulas Citation[[12]].
The concentration of oxidized Cyt. ox. calculated from the maximum change caused by aerobic (100% O2)—anaerobic (100% N2) transition.
RESULTS
shows the expected solubility of oxygen in circulated blood of the 70%-substituted rats. Each curve was calculated from oxygen dissociation curve of native blood and oxygen solubility of Neo-PFC and Fluosol-DA®. The difference of solubility between Neo-PFC and Fluosol-DA® comes from the PFC concentration of them. The DO2 (5.5%) presents the amount of oxygen delivered to tissues calculated from PaO2 and PvO2 in normal rats. At 600 Torr of PO2 value, oxygen solubility in Neo-PFC substituted and Fluosol-DA® substituted rats were 12 and 10 (vol./%), respectively. From 600 to 100 Torr, each oxygen solubility linearly decreased with PO2 changes. By contrast, the values of oxygen solubility in control model (BSA-buffer) were much smaller than both PFCs-exchanged models, because oxygen content in BSA-buffer is almost equal to water.
Figure 2. The expected solubility of oxygen in circulated blood of the 70%-substituted rats. Each curve was calculated from oxygen dissociation curve of native blood and oxygen solubility of the PFCs. The DO2 presents the amount of oxygen delivered to tissues calculated from PaO2 and PvO2 in normal rats.
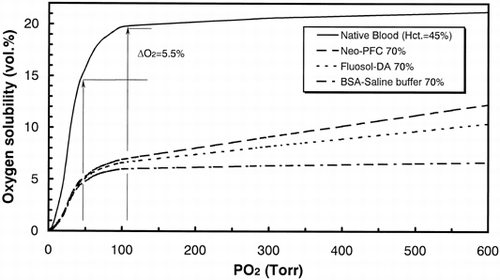
shows the oxidation states of Cyt. ox. in rat cerebral tissues plotted against the fractional inspired oxygen (FiO2). In Neo-PFC substituted model, Cyt. ox. was maintained in fully oxidized state with FiO2 values from 100 to 40%, and was gradually reduced with FiO2 values below 40%. In Fluosol-DA® substituted model, Cyt. ox. was gradually reduced with FiO2 values below 60%. In the control model, Cyt. ox. was gradually reduced with FiO2 values below 80%. In each three model, Cyt. ox. was linearly reduced with FiO2 values below 10%.
Figure 3. The oxidized states of Cyt. ox. in rat cerebral tissues plotted against the fractional inspired oxygen (FiO2). The error bars mean standard deviations (n = 7). Each percentage value was plotted using those after the exchange transfusion (100% FiO2) as references.
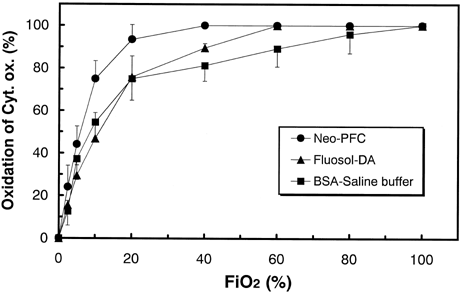
shows the oxygen partial pressure in arterial and venous blood plotted against FiO2. The PaO2 values (open symbols) strongly depend on the FiO2 value, and there were no significant changes between three models. The inset shows the same data enlarged below 60 Torr of PO2. The PvO2 values (closed symbols) depend on the characters of each blood substitute. The rank order of PvO2 values at each FiO2 was that of Neo-PFC, Fluosol-DA®, and control model (p < 0.01).
Figure 4. The oxygen partial pressure in arterial and venous blood plotted against FiO2. The error bars mean standard deviations (n = 7). Each concentration was measured from a small amount of blood drawn from a femoral artery and a cervical vein.
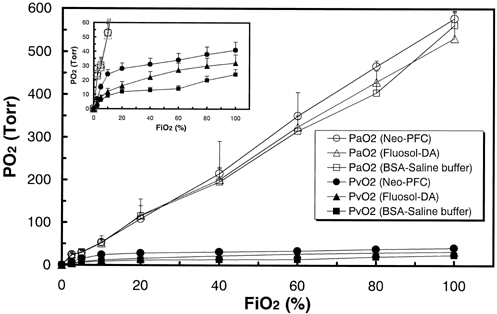
shows the oxygen carrying abilities calculated from the values of PaO2, PvO2, and oxygen solubility. At FiO2 values from 100 to 60%, the oxygen delivery by Neo-PFC and Fluosol-DA® were almost identical, and significantly larger than that by control model (p < 0.01). At FiO2 values from 40 at 20%, the oxygen delivery by Neo-PFC was significantly larger than those of Fluosol-DA® and control model (p < 0.05). At FiO2 values below 20%, no significant difference was observed in the three models.
DISCUSSION
From and , the values of oxygen delivery over 5 (vol %) are necessary for retention of oxidation states of Cyt. ox. It is conceivable that both PaO2 and PvO2 reflect the oxidation of cerebral tissues in . From , the PaO2 values did not depend on the kinds of transfusion solutions, because the PaO2 values are reflected by oxygen partial pressure in lung, and can be controlled by FiO2. By contrast, PvO2 values were regulated by venous hypoxia. From the inset of , even at 100% FiO2, oxygen delivery in control model was 4.6%, and venous hypoxia occurred (PvO2=24 Torr). This quicker response of venous hypoxia in control model was assigned to lower oxygen solubility in artery (). With 70% exchange transfusion by BSA-saline buffer, the changes in blood pressure (120.8 ± 6.8 to 83.3 ± 12.6 Torr) and heart rates (345 ± 35 to 405 ± 5/min) were coincident to complement oxygen in tissue level. The significant decrease in blood pressure (p < 0.01) was also observed in both PFC-substituted models because of their lower viscosity compared with native blood. However, significant increase in heart rates (p < 0.1) was observed only in control model. From , both in PFC-substituted models, Cyt. ox. sustains its oxidized state between 100 to 60% of FiO2. At 40% of FiO2, reduction of Cyt. ox. started in Fluosol-DA® model, because oxygen delivery decreased to 4.7%(). This advantage of Neo-PFC over Fluosol-DA® is assigned to larger concentration of PFC material (30%) than that of Fluosol-DA® (20%) ().In consequence, oxygen solubility in arterial blood in Neo-PFC is larger than that in Fluosol-DA® (), and the retardation of venous hypoxia in Neo-PFC occurred (, inset). Below the 20% of FiO2, Cyt. ox. in Neo-PFC linearly decreased to 0%, because at lower level of FiO2, the remainder of hemoglobin in blood is critical element, and quickly deoxygenated.
By the employing of new emulsifying technology, a novel PFC emulsion with a higher ability for oxygen delivery in vivo compared with others in the past was obtained, and is thought to be a candidate for blood substitute. In addition, novel PFC emulsion with a small particle size is dominant for PTCA or radiation therapy of tumor. To obtain PFC emulsion with a longer lifetime in circulation, we are now improving surface modification of PFC emulsion by stealth-type substances. The PFC emulsion that is further improved may be available in the near future.
REFERENCES
- Fujita T., Suyama T., Yokoyama K. Fluorocarbon emulsion as a candidate for artificial blood. Euro. Surg. Res. 1971; 3: 436–453
- Lowe K. Synthetic oxygen transport fluids based on perfluorochemicals: application in medicine and biology. Vox. Sang. 1991; 60: 129–140
- Sloviter H. A., Kamimoto T. Erythrocyte Substitute for Perfusion of Brain. Nature 1967; 216(4)458–460
- Yokoyama K., Yamanouchi K., Suyama T. Recent advances in a perfluorochemical blood substitute and its biomedical application. Life Chem. Rep. 1983; 2: 35–93
- Cleman M., Jaffee C. C., Wohlgelernter D. Prevention of ischemia during percutaneous transluminal coronary angioplasty by transcatheter infusion of oxygenated Fluosol DA 20%. Circulation 1986; 74: 555–562
- Doctor A., Mazzoni M. C., DelBalzo U., DiCanzio J., Arnold J. H. High-frequency oscillatory ventilation of the perfluorocarbon-filled lung: Preliminary results in an animal model of acute lung injury. Crit. Care Med. 1971; 27: 2500–2507
- Teicher B. A., Rose C. M. Perfluorochemical emulsion can increase tumor radio sensitivity. Nature 1984; 223: 934–936
- Boudewijn P. J., van der Sanden B. P., Heerschap A., Hoofd L., Simonetti A. W., Nicolay K., van der Toorn A, Colier W. N.J.M., van der Kogel A. J. Effect of carbogen breathing on the physiological profile of human glioma xenografts. Magn. Reson. Med. 1999; 42(3)490–499
- Ferrari M., Williams M. A., Wilson D. A., Traystman R. J., Hanley D. F. Cat brain cytochrome-c oxidase redox changes induced by hypoxia after blood-fluorocarbon exchange transfusion. Am. J. Physiol. 1995; 269: H417–424
- Riess J. The design and development of improved fluorocarbon-based products for use in medicine and biology. Artif. Cells Blood Substit. Immobil. 1994; 22: 215–234
- Fukushima S., Yamashita S., Otsuka K., Nakamura T., Takeuchi Shechter T. J. Pharm. Sci. Technol. Jap. 1997; 57(Suppl)70–71
- Hoshi Y., Hazeki O., Kakihana Y., Tamura M. Redox behavior of cytochrome oxidase in the rat brain measured by near-infrared spectroscopy. J. Appl. Physiol. 1997; 83(6)1842–1848