Abstract
The problem of interaction of human serum albumin (HSA), unconjugated bilirubin (UB) and high porosity activated HSGD carbons is investigated in this study. The decrease of UB to HSA molecular ratio by more than 300 times was demonstrated while the batch experiments in HSA–UB admixtures after contact with HSGD. HSGD carbons express extremely high activity for the removal of UB from HSA containing solutions (more than 100 mg of UB per 1 g of activated carbon). Ex-tempore albumin-coating of carbon surface decreases adsorbent capacity by bilirubin on 21%. At the same time ex-tempore albumin-coating of HSGD carbon surface as well as blood citratization prevent platelet and leukocytes loss and clotting inside of the column. Pharmacopoeia solution of HSA containing acetyl-tryptophan or octanoate used for albumin-coating of HSGD adsorbents, becomes ligand-free and rather more active in complexing with protein-bound substances. Combination of albumin-coated HSGD carbon as haemosorbent with HSA ligand-free solution as a transfusion media seems a new prospective modality of the extracorporeal removal of protein-bound toxins.
INTRODUCTION
It is known that activated charcoal easily adsorbs human serum albumin (HSA). Adsorptive capacities of charcoals can range from several up to hundreds of mg of protein per gram of carbonic matrix depending on its structure and albumin concentration.Citation[[1]],Citation[[2]] At the same time, activated charcoals of appropriate structure possess the high enough capacity and good kinetic properties in respect to UB mixed with albumin solution.Citation[[3]] UB is a very suitable marker for evaluation of adsorbents capacities to remove albumin-bound substances because of its high association constant (primary site assoc. const≈108 M−1) with this protein. So, if the adsorbent activity by bilirubin is sufficient, other protein-associated markers of hepatic insufficiency like phenols, bile acids, free fatty acids, mercaptans etc. could also be removed easily.Citation[[4]]
In this study in-vitro experiments are used to answer some important questions concerning interaction between albumin and bilirubin adsorption onto the surface of the highly activated carbon HSGD which was developed in our lab especially for removal of strongly protein bound substances. In parallel, problems of adsorbent haemocompatibility and ex-tempore preparation of ligand-free HSA solution are discussed.
MATERIALS AND METHODS
Chemicals
The following analytically pure reagents were used: crystalline human serum albumin (fraction V, Mr 66.500 essentially fatty acid-free, bilirubin, sodium caprylate, salicylic acid, phenol, sodium salt of deoxycholic acid (Sigma Chemical, Poole, UK), HSGD (HemoSorbent Granulated Deliganding, IEPOR, Kiev, Ukraine). All other reagents were of analytical grade and used without additional purification.
Adsorptive Treatment
Albumin solution (30 mg/ml) for batch and perfusion experiments was prepared from pharmaceutical human serum albumin (100 mg/ml) for intravenous infusion. Albumin–bilirubin solutions (0.2 mg/ml) were made using crystallized nonconjugated bilirubin. The desired weight of bilirubin was initially dissolved in a minimal amount of 0.1 M sodium hydroxide and the final volume was adjusted by solution of albumin in sodium phosphate buffer.
Batch experiments were carried out by shaking 50 mg carbon HSGD with 6 ml bilirubin–albumin solution for 120 minutes at room temperature. The assays for initial and equilibrium concentrations of bilirubin solution were done according to the commercial protocol (605-S Sigma Diagnostics) or by protocols described in the literature.Citation[[5]] Solution concentration of albumin was determined by spectrophotometer.Citation[[6]] Albumin and bilirubin concentrations were measured thrice and an average result was calculated.
The perfusion experiments were carried out in three parallel micro-columns with independent micro-pump channels and with a common inlet solution by pumping albumin–bilirubin solution at a rate of 1.25 ml/min through 10 cm3 micro-columns (with a height-to-diameter ration 7:2 cm) packed with HSGD adsorbent. Samples were taken from the outlet of all three columns at 15, 30, 60, 90, 120, 180 and 240 minutes of perfusion.
Albumin coating of HSGD adsorbent was made by perfusion of fatty acids free albumin solution of HSA (100 ml) through the micro column at a rate of 1 ml/min.
The working protocol included 10 healthy donors (5 males and 5 females) aged 35–53 years. The whole blood was obtained in the amount of 410 ml per person and divided into 135 ml and 275 ml portions. The first portion was mixed with 27 ml of standard ACD solution, and the second one was mixed with 50 ml of 0.9% sodium chloride solution containing 2000 IU of Fraxiparine. The heparinized fresh blood divided in 2 equal portions was immediately pumped through micro-columns containing 500 mg of albumin-coated and uncoated HSGD. The perfusion was carried out at a rate of 1.25 ml/min for 2 hours. The portion of blood stabilised by ACD solution was pumped through uncoated HSGD at a similar rate for 2 hours. The drop of pressure at the inlet and outlet of micro-column was measured by an EMT-035 electromanometer (Elema-Shonander, Sweden).
Leukocyte and platelet counts were performed by standard microscopic methods described elsewhere.Citation[[9]]
Differential Scanning Microcalorimetry (DSMC)
The melting thermograms of defatted albumin preloaded by bilirubin and purified albumin were recorded before and after adsorptive treatment on a DASM-4 microcalorimeter (Biopribor, Puschino, Russia) at a scanning rate of 1°C/min. The working concentration of albumin was 5.0 mg/ml. The temperature maximum T1 and T2 of the components were obtained by mathematical deconvolutionCitation[[7]] of the melting curve. Preliminary experiments with threefold repeated measurements of albumin thermal denaturation demonstrated that a deviation of temperature maximum of more than 0.5°C coincided with a significant level of p<0.01.
Flow Microcalorimetry (FMC)
The temperature effects of albumin coupling with different marker ligands (sodium octanoate, salicylic acid, phenol red, deoxycholic acid (sodium salt) in equimolar concentrations were measured by a flow microcalorimeter 2277 Thermal Activity Monitor (LKB, Bromma, Sweden) at ambient temperature. The enthalpies of complexing were calculated.Citation[[8]]
Scanning Electron Microscopy (SEM)
Due to the well defined shape of HSGD granules, the electron microscopy of the granules was relatively straightforward. Some number of granules were placed into a plastic Petri dish. The support plate, with double-sided sticky tape, was lightly pressed into granules crowd by taped side from above. Later, to granules which stuck on the tape the special conductive silver paste was applied from the side in order to provide better mechanical stability and high electron beam delivered charge removal rate. In the result the sides of granules available for electron microscopy examination were not covered by any agent or metal or subjected to any extra processing after rinsing and drying. Thus it is highly likely that observed surface properties such as microporous structure are not artefact of preparation method. The scanning electron microscope used was a Hitachi S-5200 in-lens field emission; accelerating voltage was 4 kV. Images were recorded using the secondary electron signal.
RESULTS
describes an SEM picture of the adsorbent external surface. Under the small magnification spherical adsorbent granules look solid and smooth enough. But under the higher magnifications one can see that the adsorbent surface consists of a great number of open pores. Calculations (omitted) give the part of volume of these granules occupied with carbon itself not exceeding 5–10% of granules total volume.
Figure 1. First two pictures (A, B) were done in low resolution mode to demonstrate shape parameters of granules of HSGD carbons. Granule in the middle of the first picture was taken for high magnification examination. Three last pictures (C, D, E) are consequent magnification zooms of the same area on the granule. Scale bars are provided in the bottom right corner of each picture. Numbers correspond to the length of the entire scale bars.
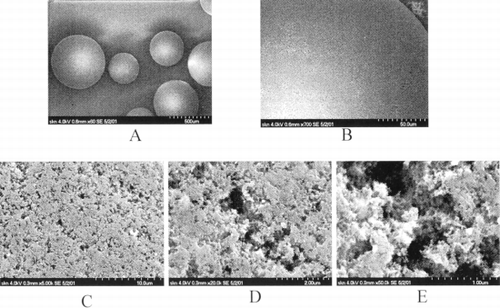
reflects the fact that HSGD carbon activity adsorbs both UB and albumin from its mixture. Under the particular conditions used here the bilirubin concentration drop was 97.8% and the albumin concentration drop was 34.9% with adsorbent capacity for albumin 1147 mg/g and for bilirubin—20.7 mg/g. The bilirubin–albumin molecular ratio after adsorptive treatment was diminished from 0.74 to 0.0024, or by approximately 310 times. A high level of purification of residual (unadsorbed) albumin was also demonstrated by marked changes in HSA melting curves, which after treatment approached the melting curve of analytically pure defatted albumin ().
Table 1. Changes in the Concentrations of Nonconjugated Bilirubin and Albumin After 2 Hours of Contact with Activated Carbon in Batch Experiment (Bilirubin–Albumin Solution—6 mL, HSGD—0.05 g; n=5; M±SD)
Figure 2. Melting curves of HSA: (A) defatted HSA; (B) loaded with unconjugated bilirubin up to bilirubin–albumin molecular ratio 0.74; (C) purified onto HSGD carbons up to molecular ratio 0.0024.
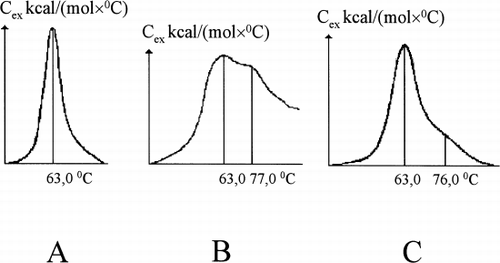
In one can see that during albumin-coating the outlet concentration of HSA becomes equal to the inlet concentration after 45 min of perfusion (adsorbent saturation). At this time the column retains approximately 7.9% of pumped albumin, or 1186 mg of protein per gram of adsorbent. During the rinsing of the column with saline a zero outlet concentration of albumin could not be reached by 75 min of perfusion but, from a comparison of the outlet and pool concentration data, one can conclude that the main albumin release takes place during the first 15 minutes. Total protein release consisted of 3.28% of previously pumped albumin or 492.4 mg per gram of adsorbent. Consequently, the HSA portion, more or less strongly fixed onto the HSGD carbon surface amounted to nearly 5.6% of the perfused protein, or 693 mg per gram.
Table 2. Average Results of 3 Microcolumn Experiments with Albumin-Coated Activated Carbon and Saline Rinsing of 0.5 g HSGD Charcoal (HSA Inlet Concentration—81 g/L, Perfusion Rate—1.25 ml/min)
shows the curves of outlet concentrations of UB for columns containing uncoated or albumin-coated HSGD carbon. Both columns exhibit extremely high activity for removal of UB from the albumin–bilirubin mixture, but adsorptive capacity of albumin-coated adsorbent is 21.4% less than that of uncoated one (80 mg of UB per gram of adsorbent versus 63 mg/g, respectively). These data are actually for 240 min of perfusion time but at the end of the experiment both columns still preserved essential adsorptive capacity. Linear approximation of the outlet curves up to the inlet level of bilirubin concentration (dotted lines) gave estimates of the complete capacity of uncoated and albumin-coated carbon as 113 mg/g and 88 mg/g, respectively.
Figure 3. Average outlet bilirubin concentration curves for uncoated (□) and albumin-coated (•) HSGD carbon.
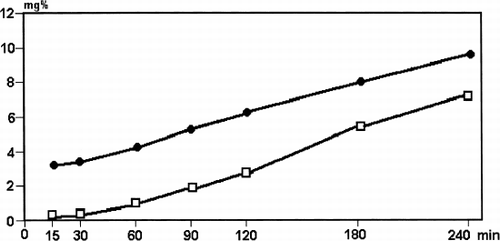
Difference in the outlet HSA concentration for albumin-coated and uncoated HSGD () was evident at the beginning of the experiment and became negligible with the increase of the amount of perfused protein as the dry weight of latter exceeds the weight of sorbent in 257 times at the end of perfusion. At the same time, albumin-coating strongly influenced the microcolumn flow resistance in the case of the heparinized human blood in-vitro perfusion. The rapid increase of flow resistance and “caking” of uncoated HSGD carbon () coincided with the remarkable consumption of platelets and leukocytes inside the microcolumn ().
Table 3. Albumin Consumption with Uncoated and Albumin-Coated HSGD Carbon (Average Results from 3 Microcolumn Experiments; HSA Inlet Concentration—34.3 g/L)
Table 4. Evaluation of Statistical Significance of the Difference in Leukocytes and Thrombocytes Count Between Inlet and Outlet of the Microcolumns HSGD Carbon Perfusing with the Fresh Donor Blood for 120 Min
In the case of albumin-coating of the adsorbent or citratization of blood there were no platelets and white blood cells loss observed and an increase of microcolumn flow resistance with time was very slow (, ).
If an official (pharmacopeial) solution of HSA for i.v. transfusion was pumped through the column the melting curve of HSA at the outlet looks quite different from its initial shape and very similar to the melting curve of the pure defatted HSA ().
Figure 5. Melting curves of officinal HSA solution for transfusions before (A) and after (B) its purification onto HSGD carbons in the regimen, similar to the adsorbent coating with albumin. (C) melting curves of defatted HSA, “Sigma,” USA.
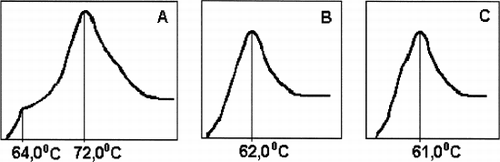
describes the difference in transport abilities of the official and purified HSA in relation to four marker ligands for the main binding sites of albumin. shows that pharmacopeial albumin after exposure to HSGD carbon exhibited enhanced binding towards each of the ligands. This is demonstrated by the enhancement of the appropriate microcalorimetric parameter Hc (enthalpy of complexing). This purified preparation in its complex-forming abilities was very close to analytically pure “Sigma” fatty free HSA ().
Table 5. Comparation of Flow Microcalorimetry Data (ΔHc, kcal/mole) Reflecting Complex-Forming Abilities of Main Binding Sites of Different Samples of HAS
DISCUSSION
Batch experiments with HSGD carbon demonstrated very good adsorption of UB from defatted HSA solution. (). In such experiments it is difficult to compare different capacities of adsorbents because of different levels of residual (equilibrium) concentration of adsorbed substances. However, despite a very low final concentration of UB, capacity of HSGD adsorbent (20.1 mg/g) is definitely higher than that of both conventional synthetic hemosorbent (SCN, 0.8–1.1 mg/g) and of previous version of HSGD carbon (5–15 mg/g).Citation[[4]]
Because of relatively high amount of adsorbent in comparison with the amount of protein (50 mg of carbon against 164 mg of albumin) albumin loss was also apparent (35%). Anyway, the ligand-carrier molecular ratio was diminished by more than 300 times, which reflects the high quality of purification of residual 65% of albumin from tightly (association constants of the order of 108 M−1)protein-bound ligand. The results of differential scanning microcalorimetry also demonstrated that the molecular conformation of this purified albumin was very similar to that of a Sigma fatty acid-free standard.Citation[[10]]
In a dynamic experiment with microcolumns and high (81 g/l) concentration of HSA the saturation of HSGD carbon occurred after 30–45 minutes of perfusion. The protein–sorbent ratio weight in these experiments was 152 or 46 times higher than in the previous case. So, the proportion of protein retained with the adsorbent consisted of only 7.9%. After rinsing with saline solution the weakly bound fraction of albumin molecules was washed out mainly in the first 15 minutes and the residual amount of protein consisted of 693 mg per gram of HSGD adsorbent. This was a significant amount in relation to the weight of adsorbent, so such an adsorptive system could be defined as protein-carbonic, but not purely carbonic.
The above-described ex-tempore albumin-coating diminished the adsorptive capacity of HSGD carbons by UB by at least 20%. It is a typical situation for all kinds of coating, regardless of its chemical nature and origin of the polymers used, and derives from polymer-induced enhancement of diffusion resistance on the border between liquid and solid phases.Citation[[11]] At the same time, a decrease in the capacity of HSGD adsorbents by bilirubin could not be explained by the competitive adsorption of another ligand, because the protein used for the preparation of the bilirubin–HSA mixture was highly purified and fatty-acid-free. These results contradict some previous dataCitation[[12]] where the authors found that pretreatment of adsorbents by albumin enhanced the adsorptive capacity of activated carbons towards direct and indirect bilirubin. This disagreement could be explained by the difference between the adsorbents that were used: if adsorbent has a small capacity for bilirubin removal from albumin solutions, adsorption of pure albumin onto the surface of a less-effective carbonic adsorbent can overcome the negative effect of the coating by this biopolymer. Nevertheless in our experiment HSGD carbon demonstrated an impressive adsorptive capacity of 113 mg/g for uncoated and 80 mg/g for albumin-coated adsorbent. Extrapolation of these data to real plasmaperfusion conditions, would mean that 10 grams of HSGD carbon would be enough for removal of 600–800 mg of bilirubin per session. This amount of adsorbent could be compared with the 875 g of adsorptive materials, recently used by Italian authors to achieve the similar bilirubin removal rate.Citation[[13]]
However, plasmoperfusion is not the only use for HSGD adsorbent. One can see that (, ) albumin-coated grains of this carbon demonstrate stable hydrodynamics and good haemocompatibility properties during in vitro contact with whole human blood. Uncoated carbon starts to initiate the clotting of heparinized blood and catch blood cells after 30 minutes of perfusion, which is quite expectable taking into account the rough microstructure of the granules' external surface (). Thus microcolumn flow resistance rapidly goes up and plugging occurs between 40 and 45 minutes of perfusion. In total, these findings are in good agreement with the classical data of Chang,Citation[[14]] who demonstrated first the remarkable role of albumin-coating of charcoal surface in the prevention of blood cell trauma and column plugging. At the same time, citratization of blood gives pressure drop results similar to albumin-coated adsorbent, and enhances some other parameters of adsorbent haemocompatibility. This coincides with result ofCitation[[15]] also obtained much earlier.
Nevertheless, albumin-coating plus heparinization remains very attractive treatment modality, especially taking account the expanding role of massive albumin transfusion in the treatment of hepatic coma and some complications of ascites.Citation[[16]] The use of conventional pharmacopeial solution of HSA for intravenous transfusion instead of analytically pure defatted albumin for ex-tempore coating of HSGD carbon leads to drastic changes in properties of pharmacopeial HSA solution because of the effective removal of thermostabilizers (octonoate, n-acetyl-tryptophan) and some natural hydrophobic ligands by adsorbent. The molecular conformation of this albumin and its complex-forming abilities come close to those of defatted analytically pure protein or liquid protein adsorbent Albomax.Citation[[17]]
Transfusion of such “activated” HSA before or during extracorporeal sessions could be useful for attracting hydrophobic toxins from the tissue compartment into the blood stream and to make adsorptive treatment more effective.Citation[[18]] If HSA transfusion is used in encephalopathic patients, one should remember that the caprylate (octanoate) anion is traditionally described as an important encephalotoxinCitation[[19]] and consequently octanoate-free albumin solution is preferable for the treatment of hepatic coma and precoma. Nothing will be lost, however, if a part of the adsorptive capacity of adsorbent is used for octanoate removal from the HSA transfusion solution, because this small deficit of column efficacy should be compensated for by the enhancement of complex-forming activity of transfused HSA. So, a combination of albumin-coated HSGD adsorbent with the transfusion of purified albumin onto this adsorbent looks to be an attractive new modality of the treatment of some end-stage hepatic diseases and its complications.
CONCLUSIONS
Deligandizating granulated hemosorbents HSGD express extremely high activity (100 mg/g) for the removal of unconjugated bilirubin from albumin solution.
Ex-tempore albumin-coating of carbon surface decreases adsorbent capacity by bilirubin on 21%.
Ex-tempore albumin-coating of HSGD carbon surface as well as blood citratization prevent platelet and leukocytes loss and clotting inside of the column.
Conventional (pharmacopeial) solution of HSA used for albumin-coating of HSGD sorbents, becomes octanoate-free and rather more active in complexing with protein-bound ligands.
Combination of albumin-coated HSGD carbon with ligand-free solution of HSA seems to be a new prospective modality of extracorporeal biochemical correction in patients with hepatic insufficiency.
HSGD: | = | haemosorbent granulated deliganding, new generation of activated carbons |
HAS: | = | human serum albumin |
UB: | = | unconjugated bilirubin |
REFERENCES
- Eretskaya E. V., Nikolaev V. G., Sergeev V. P., Stephanov A. V., Vovianko S. I. Study of adsorption of blood plasma proteins with carbonic activated fibers. Khim.-Farm. Zh. 1985; 3: 360–365
- Eretskaya E. V., Shulepov Y. V., Grebennikov S. F., Korneeva L. N., Pilipenko S. V., Kinin A. T., Eretsky E. L. Study of equilibrium and kinetic charakteristics of adsorption of serum albumin with activated charcoal. Dokl. Akad. Nauk Ukr., Biochemistry 1994; 12: 134–139
- Dunlop E. H., Hughes R. D., Williams R. Physico-chemical aspects of removal of protein bound substances by charcoal and other adsorbents of potential value in systems of artificial liver support. Med. Biol. Eng. 1978; 16: 343–349
- Nikolaev V. G., Sarnatskaya V. V., Sigal V. L., Klevtsov V. N., Makhorin K. E., Yushko L. A. High-porosity activated carbons for bilirubin removal. Int. J. Artif. Organs 1991; 14(3)179–185
- Doumas B. T., Watson W. A., Biggs H. G. Albumin standards and measurement of serum albumin with bromcresol green. Clin. Chim. Acta 1971; 31: 87–96
- Iendrassik L., C'ieghorn R. Biochem. Z. 1937; 289: 1
- Privalov P. L., Potekhin S. A. Scanning microcalorimetry in studying temperature-induced changes in proteins. Methods Enzymol. 1986; 131: 4–51
- Coassolo P., Sarrazin M., Sari J. S., Briand C. Microcalorimetric studies on the binding of some benzodiazepin derivatives to human serum albumin. Biochem. Pharmacol. 1978; 27: 2787–2792
- Men'shikov V. V. Laboratory Methods of Investigation in Clinic. Meditsina, Moscow 1987; 122–137
- Nikolaev V. G., Sarnatskaya V. V., Ivanyuk A. A., Yushko L. A. Thermodynamic Criteria for the Removal of Certain Hepatic Insufficiency Markers from Protein-Containig Solutions. Artificial Liver Support, G. Brunner, M. Mito. Springer Verlag, Berlin 1992; 197–210
- Nikolaev V. G. Hemocarboperfusion in Experiment and Clinic. Naukova Dumka, Kiev 1984; 129–135
- Fesenko E. A., Gaylor J.D. S., Smith E. M., Mikhalovsky S. V., Courtney J. M. Adsorption of bilirubin on active carbons. Int. J. Artif. Organs 1998; 21: 642
- Pazji P., Scagliarini R., Puviani A. C., Lodi G., Moisiani E., Gullini S. Biochemical assessment and clinical evaluation of non-ionic adsorbent using in patients with intractable jaundice. Int. J. Artif. Organs 2000; 23: 312–318
- Chang T.M. S. Artificial Cells. Springfield (Ill)USA 1972
- Scharschmidt B., Marfine J., Shapiro L., et al. The use of calcium chelatiny agents and prostoglandine E to eliminate platelet and white blood cells losses resulting from hemoperfusion through uncoated albumin–agarose gel and neutral and cation-exchange resines. J. Lab. Clin. Med. 1977; 89: 110–119
- Chormomyz V., Sarnatskaya V., Zemskov V., Yushko L., Maslenny V., Nikolaev V. Cirrhosis: Adsorptive Purification and Reinfusion of Ascitic Fluid. European Congress I.H.P.B.A. Budapest 99, L. Flautner, P. K. Kupcsulik, I. Rozsa. Monduzzi Editore S.P.A., Bologna 1999; 179–183
- Sarnatskaya V. V., Nikolaev V. G., Lobunets K. A., Yushko L. A., Ivanuk A. A., Nikolaev A. V. Albomax as a promising liquid adsorbent for binding hydrophobic markers. Artif. Organs 1993; 17: 487
- Sorf P., Navasa M., Arroyo V., Aldeguer X., et al. Effect of intravenous albumin on renal impairment and mortality in patients with cirrhosis and spontanous lactorial peritocitis. New Eng. J. Med. 1999; 341: 403–409
- Zieve L. Metabolic Abnormalities in Hepatic Coma and Potential Toxines to be Removed. Artificial Hepatic Support, R. Williams, J. M. Murray. Pitman Medical, Lion, London 1975; 11–26