Abstract
Rabbit liver cytosolic glutathione‐s‐transferase (GSTs; EC: 2.5.1.18) was immobilized in cross‐linked gelatin cylindrical molds which the formaldehyde was used as cross linker. Glutathione‐s‐transferases are the enzymes that catalyze the conjugation of wide variety of electrophilic substrates with glutathione. This process generally leads to the detoxification of the xenobiotics. Characterization of immobilized GST was made by using 1‐chloro‐2,4‐dinitrobenzene(CDNB) as substrate. Immobilization efficiency of the enzyme was calculated in terms of activity yield. Optimal enzyme, gelatin and formaldehyde amounts, optimum pH and temperature, Km[CDNB], Km[GSH] and thermal stability was searched and compared with the free enzyme. Furthermore, for studying the detoxification by conjugation in vitro, immobilized GST was tested using stirred batch reactor system and found suitable with a high yield of 2,4‐dinitrophenyl‐GSH conjugate formation.
Introduction
Glutathione‐s‐transferases (GSTs; EC:2.5.1.18) are a family of multifunctional enzymes involved in the cellular detoxification and excretion of many physiological and xenobiotic substances. They catalyse the nucleophilic addition of the thiol of reduced glutathione (γ‐glutamyl‐cysteinyl‐glycine) to electrophilic centers in organic compounds (Mannervik and Danielson, [Citation1987]; Warholm et al., [Citation1981]; Wilce and Parker, [Citation1994]). The glutathione conjugates so‐formed are rendered were water soluble, thus facilating their eventual elimination. This reaction is one of the early steps along the mercapturic acid pathway in which hydrophobic xenobiotics are inactivated and eliminated from the organism (Habig et al., [Citation1974]). The hepatic GSTs have been found to be both microsomal and cytosolic with differences in substrate specificity (Mannervik and Jensson, [Citation1982]; Morgenstein et al., [Citation1983]).
Detoxification plays an important part in the treatment of hepatic failure. An approach to eliminate lipophile toxins is the applications of enzymes. The immobilization of detoxification enzymes offers several advantages over use of conventional microsomal, cytosolic or soluble enzyme preparations (Brugmann et al., [Citation1990]; Dulik and Fenselau, [Citation1988]). These include ease of handling, facile separation of products from enzyme incubation mixtures, stabilization of the enzyme toward thermal or oxidative degradation and the opportunity to recover and reuse the enzyme catalyst. These advantages have been of particular value for enzymes and enzyme systems that are difficult to isolate, are unstable, have intrinsically low reaction rates and require expensive cofactors. The enzymes that metabolize xenobiotics to polar conjugates fall into this category.
Immobilization of detoxification enzymes have found applications such as; for synthesis of drug and xenobiotic metabolites, to understand both mechanistic and kinetic aspects of conjugation reactions and to assist in artificial liver support for detoxification.
Glutathione‐s‐transferase is a soluble enzyme which has been investigated for fixation and detoxification. It is a carrier protein for many toxic substances, having a higher binding constant in most cases to albumin. Therefore, the advantages of immobilization of glutathione‐s‐transferase are three fold; the enzyme is not bound to cell particles and thus does not have to be detached from organelles, the enzyme in accordance with its importance is present in large amounts in the cytosol of liver and kidney and the necessary cofactor glutathione is very cheap and easy to prepare.
Gelatin has been shown to be useful and very versatile support matrix for the immobilization of biologically active macromolecules (Bigi et al., [Citation1998]; Grodzinski, [Citation1999]). Gelatin offers a number of important advantages in its use as a carrier matrix in that, it is abundant in nature, inexpensive and safe material. The protein nature of gelatin, its high hydrophilicity and consequently its strong swelling power provide environmental conditions very favorable for immobilized enzymes and reduce the mass transfer resistance to the diffusion of substrate and reaction product. On the other hand, at temperatures below 40°C, gelatin gels form gelatin sols that they are thermally reversible and also mechanically unstable. However, thermostability and mechanical strength can be achieved by treating gelatin gels with cross linkers especially formaldehyde and glutaraldehyde among others (Bayramoğlu et al., [Citation1992]).
The aim of this study was to provide versatile method for the immobilization of glutathione‐s‐transferase within cross‐linked gelatin cylindrical molds by means of using formaldehyde as hardener.
Materials and Methods
Glutathione(GSH), 1‐chloro‐2,4‐dinitrobenzene(CDNB), Gelatin Type II, Folin Cioucalteu Reagent, Bovine Serum Albumin(BSA) were obtained from Sigma Chemical Co., St. Louis. All other chemicals were purchased from local suppliers and were analytical grade or better.
New‐Zealand White rabbits were sacrificed by a sharp blow to the head followed by cervical dislocation.
Preparation of Cytosolic Glutathione‐s‐transferase
Livers were removed and carefully perfused with cold saline. All subsequent manipulations were performed at 4°C. Livers were homogenized in 3 volumes of ice‐cold 0.1 M phosphate buffer(pH 7.4) containing 1 mM EDTA. This homogenate was centrifuged for 20 minutes at 10000 g. Resulting supernatant was decanted through glass wool to trap fat particles and further centrifuged at 100000 g for 1 hour. The supernatant was lyophilized, stored at − 20°C and used for immobilization.
Cytosolic protein concentration was assayed by the method of Lowry et al. using Bovine Serum Albumin as standard (Lowry et al., [Citation1951]).
Enzyme Immobilization
A solution of gelatin (10% and various) in water maintained at 40°C. To 9.5 ml of this dispersion, 0.5 ml of a hardening solution consisting of formaldehyde (20% and various) in 50% v/v ethanol is added and within 1 minute appropriate volume of enzyme solution was added. This has the advantage of reducing the exposure of enzyme to formaldehyde rendering it a more active preparation. Cylindrical molds were constructed from a disposable hypodermic syringe with the needle end of the barrel cut off squarely. The mixture is quickly poured into cylindrical mold (0.4–5 cm) and allowed to gel in a deep freezer(about − 20°C). After at least 5 hours, the mold is brought to room temperature and the gelatin cylinder obtained is thoroughly rinsed with distilled water and kept wet overnight at 4°C and then cut into thin disks (0.1 cm).
Assay of Glutathione‐s‐transferase Activity
Glutathione‐s‐transferase activity was assayed according to Habig et al., [Citation1974] with slight modifications using 1‐chloro‐2,4‐dinitrobenzene(CDNB) as substrate. The reaction mixture contained 1mM GSH, 1mM CDNB in 0.1M phosphate buffer; (pH 7.0) and the enzyme source (free and immobilized form with similar protein amount) in a total volume of 3 ml. The reaction mixtures were preincubated for 2 minutes and the reaction was started by adding CDNB. Incubation took place in a shaking water bath for 5 minutes. Under these conditions, product formation was linear with respect to time and protein concentration. The reaction was stopped by the addition of 0.2 ml of 33% TCA and absorbance was read at 340 nm. The enzyme activity was expressed as units/mg protein (μmol/min/mg protein). The enzyme unit was calculated using the molar extinction coefficient of 9.6 mM− 1cm− 1 for CDNB.
Characterization of Immobilized Cytosolic GST Preparation
In order to make comparison of the immobilized and free enzyme activities, it is necessary to know the content of enzyme which was entrapped in molds. For this aim, activity and protein amounts of initial enzyme preparation and washing solutions were estimated and the immobilization efficiency was calculated in terms of activity yield. To achieve the best results, the optimal enzyme‐to‐gelatin ratio (as mg protein / % gelatin) in relation to the particular type of the enzyme and the effect of formaldehyde on the enzyme was evaluated by incubating at 37°C for 5 minutes a fixed amount of enzyme with increasing amount of formaldehyde.
The GST activity as a function of pH and temperature was also determined. To establish the kinetic parameters such as Km, series of experiments were carried out by using differing concentrations of CDNB (0.1–2.0 mM) and glutathione (0.1–2.0 mM) as a second substrate.
Thermal stability of immobilized GST via free enzyme was determined as follows; immobilized enzyme and free enzyme which has the same amount of protein concentration were first incubated for half an hour in various temperatures (0–60°C) and then the activities were measured in standard conditions of GST assay.
Furthermore, the conjugation reaction for the investigation of in vitro detoxification was also studied towards CDNB in stirred batch reactor system which the reservoir contained 30 μmol GSH, 30 μmol CDNB in phosphate buffer pH 7.0 and magnetically stirred at 37°C. Assays were performed on 50 μl aliquots of the reservoir content at zero time and periodically for different times during the course of reaction.
Results and Discussion
By means of activity determinations in the washings and the immobilization medium indicated that approximately 82–88% of the added GST enzyme activity remained in the cross‐linked gelatin spherical molds. This discrepancy indicates that enzyme escapes from the molds during washings. This has also been an experience with other enzyme entrapment systems reported before (Zihnioğlu and Telefoncu, [Citation1995]), such as chitosan, alginate, carragenean etc. Therefore, for comparisons between free and immobilized GST preparations, the assays were normalized on the basis of mg cytosolic protein and GST activity initially used per assay. To observe the effect of enzyme concentrations (1–10 mg protein corresponding 0.4–4.0 Units) were tested. Optimal enzyme‐to‐gelatin ratio (as mg protein / ml gelatin) and the effect of cross linker formaldehyde were represented in . There is a more or less restricted limit to the amount of enzyme (mg or units) that can be immobilized per ml of 10% gelatin without changes in the apparent specific activity which is given as activity yield per set of immobilization. As is seen in , the activity yield was best obtained at 8 mg (3.2 Units) per milliliters of 10% gelatin. Since gelatin and cross linker (formaldehyde) concentrations kept constant, increasing the gelatin and enzyme concentration may possibly cause enzyme‐enzyme cross linking. The other proposed explanation of the results is that, with increased loading, the enzyme becomes over saturated within the matrix pore and thus restricts the product and the substrate diffusion. The effect of ethanolic formaldehyde of cytosolic GST preparation was investigated in order to select the most suitable amount of cross linking (formaldehyde) agent to be used in the rest of the work. The gel that produced maximum enzyme activity yield was determined as 1.0% (). The results showed that, as the formaldehyde concentration in the gelatin mixture increased, the leakage of the enzyme decreased. However, increasing the formaldehyde concentration caused activity lost. This may be responsible of either denaturation of the enzyme or co‐cross linking the latter to gelatin chains in the stabilized gel network, thus causing steric hinderance. So that for cytosolic GST immobilization in gelatin matrix up to 1.0% of formaldehyde ratio as a hardener seemed appropriate.
Table 1. Effect of enzyme, gelatin and formaldehyde concentration on the immobilization efficiency of cytosolic GST preparation by means of activity yield
The optimum pH of the free enzyme and immobilized enzyme was found to be at pH 7.0 (). The optimum temperature was at 37°C () meaning immobilization has no effect both on optimum pH and temperature.
Figure 1. The effect of pH on the activity of free(▪) and immobilized(•) GST activity (sodium acetate; pH 3.0–6.0, phosphate; pH 6.5–7.5, borate; pH 8.0–9.0).
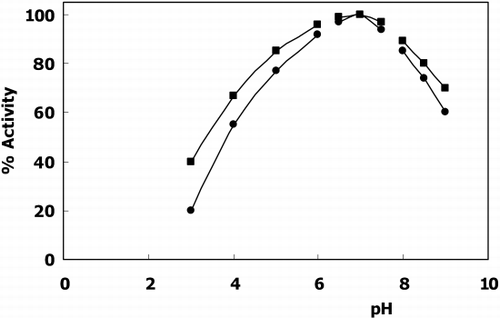
Although the interpretation of kinetic data obtained for enzyme such as glutathione‐s‐transferase which exists as multiple isoforms with overlapping substrate specificity is generally difficult. However, some useful information could be obtained from a basic kinetic analysis of GST by using general substrate CDNB. Km for both CDNB and the co‐substrate GSH were calculated by the use of Lineweaver‐burk plots with either [CDNB] or [GSH] held constant at approximately saturating levels, while the reactants was varied over a constant range of concentrations (0.1–2.0 mM CDNB or 0.1–2.0 mM GSH) Km[CDNB] for free and immobilized GSTs were found as 6.25 × 10− 4M and 10 × 10− 4M, respectively and Km[GSH] was determined as 3.7 × 10− 4M and 6 × 10− 4M for free and immobilized enzyme. Only a little lack of substrate affinity can be seen in immobilized enzyme which could be explained by diffusion as a character of the immobilization method.
Thermal stability of immobilized glutathione‐s‐transferase within formaldehyde cross linked gelatin cylindrical molds via free enzyme is shown in .
The figure clearly indicated that the thermal stability of the GST was increased after immobilization. In principle thermal stability of an enzyme can increase, decrease or unchangeable after immobilization process. The results confirm that immobilization supports the thermal stability of the enzyme.
The conjugation rate of CDNB with glutathione to form GSH‐conjugate of immobilized cytosolic glutathione‐s‐transferase for achieving the detoxification ability was tested by determining the product concentration in the reservoir for the stirred batch reactor. The quantities of 2,4‐dinitrophenyl glutathione in a batch reactor system of conjugation is shown in .
Figure 4. Quantities of 2,4‐dinitrophenyl‐GSH in a stirred batch reaction of glutathione conjugation.
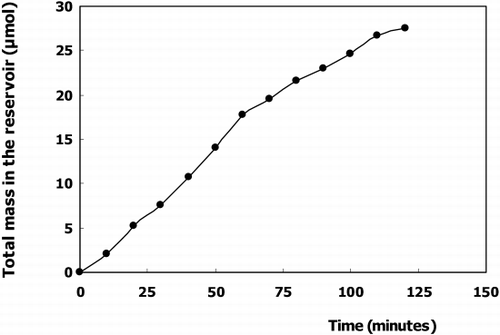
indicates that by using stirred batch reactor system, a continuous production of 2,4‐dinitrophenyl‐GSH conjugate occurs up to 27.5 μmol which the reaction was terminated after 2 hours. This can be mainly explained by the saturation of the reaction product (initial substrate amount 30 μmol) in the reservoir meaning most of the CDNB was converted to its product.
The conversion of CDNB(x) is defined as the proportion of substrate consumed at time t[x = (So − St)/So] where So and St are the concentrations of CDNB in the reservoir and time, t, respectively. The product concentration corresponds to the difference So − St. Thus the variables x and t may be plotted on a conversion curve ().
The conversion curve of CDNB shows that in 2 hours, 91.7% of CDNB converted to 2,4‐dinitrophenyl‐GSH conjugate. In conclusion the results of these experiments in vitro seems to be advisable for extrocorporal application.
The future of immobilized enzymes as important tools for drug metabolism studies appears promising. However, support of failing liver functions (primarily detoxification) requires more than one enzyme system. The combination of enzymes such for hydroxylation, glucuronidation and glutathione binding will cover the detoxification of a large range of materials. In the case of immobilization of these enzymatic systems possibly should have applications in clinical medicine.
References
- Bayramoğlu Z., Akbulut U., Sungur S. Immobilization of α‐amylase into gelatin films with various cross linkers. Biorg. Med. Chem. Lett. 1992; 2: 427–439
- Bigi A., Bracci B., Cojazzi G., Panzavolta S., Roveri N. Drawn gelatin films with improved mechanical properties. Biomaterials 1998; 19: 2335–2340
- Brugmann E., Stange J., Falkenhagen D., Dummler W., Ernst B., Dautszenberg H. Biomat., Art. Cells, Art. Org. 1990; 18(4)513–515
- Dulik D. M., Fenselau C. Use of immobilized enzymes in drug metabolism studies. FASEB J. 1988; 2: 2235–2240
- Grodzinski J. J. Biomedical application of functional polymers. React. Funct. Polym. 1999; 39: 99–138
- Habig W. H., Pabst M. J., Jacoby W. B. Glutathione‐s‐transferases: the first enzymatic step in mercapturic acid formation. J. Biochem. 1974; 249: 7130–7139
- Lowry O. H., Rosenbrough N. T., Farr A. L., Randall R. J. Protein measurement with the folin phenol reagent. J. Biol. Chem. 1951; 93: 265–275
- Mannervik B., Danielson U. H. Glutathione‐s‐transferases: structure and catalytic activity. Crit. Rev. Biochem. 1987; 23: 283–337
- Mannervik B., Jensson H. Binary combinations of four protein subunits with different catalytic specificities explain the relationship between six basic glutathione‐s‐transferases in rat liver cytosol. J. Biol. Chem. 1982; 257: 9909–9912
- Morgenstein R., DePierre J. W., Jörnvall H. Microsomal glutathione transferase; primary structure. J. Biol. Chem. 1983; 260: 13976–13983
- Warholm M., Gutenberg C., Mannervick B., VonBahr C. Glutathione‐s‐transferases from human liver. Biochem. Biophys. Commun. 1981; 98: 512–517
- Wilce M. C.J., Parker M. W. Structure and function of glutathione‐s‐transferases. Biochem. Biophys. Acta 1994; 120:51–120:18
- Zihnioğlu F., Telefoncu A. Preparation and characterization of chitosan‐entrapped microsomal UDP‐glucuronyl transferase. Art. Cells, Blood Subs. Immob. Biotech. 1995; 23(4)545–552