Abstract
The variations of membrane bound total sialic acid (TSA) and lipid peroxidation level dependent on the antioxidant enzyme activities such as Superoxide Dismutase (SOD), Catalase (CAT), Glutathione peroxidase (GSH-Px) have been studied in yeast extract supplemented medium. The maximum SOD and CAT activities of F. equiseti tended to increase with raises of yeast extract concentration up to 25 g/L where they were determined to be 78.6±0.96 and 312.7±5.6 IU/mg. On the other hand, SOD and CAT activities in F. acuminatum significantly increased with the rise of yeast extract concentration up to 10 g/L (p < 0.01) and maximum activities were observed at this concentration as 36.3±0.54 and 115.3±2.19 IU/mg on the 12th day incubation. Other H2O2 scavenger enzyme, GSH-Px activities of F. equiseti and F. acuminatum were reached the maximum at 5 and 25 g/L yeast extract and determined as 5.06±0.04 and 4.74±0.09 IU/mg, respectively. TSA level showed positive correlation with SOD and CAT activities while LPO levels variations negatively correlated. The results may indicate that these antioxidant enzymes also appeared to be involved in protecting membrane bound sialic acids as well as membrane lipid of the fungus from exogenous reactive oxygen species.
INTRODUCTION
Sialic acids are a family of monosaccharaides comprising several derivatives of neuraminic acid including N-glycolyl and N-acetyl groups, as well as O-acetyl and other O-substitutions [Citation[1], Citation[2]]. They are important terminal or subterminal units in oligosaccarides from complex glycoconjugates expressed at the cell surface. Naturally occurring sialic acids are known to mediate many important biological pheneoma involving cell-cell and cell-matrix interactions [Citation[3], Citation[4]]. It is well known that the number and derivative of sialic acids accord strictly with such conditions as growth medium and aging period [Citation[4]]. On the other hand, one of the important reasons for aging is production of reactive oxygen species [Citation[5]]. Reactive oxygen species (ROS) by products of molecular oxygen consumption in the electron transport chain are also generated into the mitochondria [Citation[6]]. These reactive oxygen species, namely superoxide radical, hydroxyl radical and hydrogen peroxide, oxidatively damage lipids, proteins and nucleic acid [Citation[7]]. Polyunsaturated fatty acids are especially sensitive to ROS attack, and this initiates a chain reaction that forms lipid peroxides. Therefore, LPO has been identified as the major biochemical alteration underlying oxidant induced cell injury [Citation[8]]. The biological systems have evolved to develop an effective and complicated network for defence mechanisms to efficiently handle the harmful oxidative environments [Citation[9]]. These defence mechanisms include enzymatic and non enzymatic defences. The enzymatic systems include cytosolic and mitochondrial superoxide dismutases (SODs), catalase and glutathione peroxidases [Citation[10], Citation[11]]. Natural antioxidants include low molecular weight compounds such as ascorbic acid, vitamin E, glutathione and β-carotene. The antioxidant and antioxidant enzymes in an organism may operate within the context integrated systems. The component system may work synergistically to scavenge reactive oxygen species to prevent oxidative damage. During anaerobic growth, some antioxidant defences such as SODs, catalases and peroxidases are present at a very low level or even not present. In fact, carbohydrates and nitrogen sources represses the transcription of a number of genes, including some of the enzymatic antioxidant defences [Citation[12]].
The scope of this study is the survey of the central feature of the variations of membrane bound total sialic acid and LPO levels in Fusarium species depending on the antioxidant and antioxidant enzymes with respect to yeast extract concentrations in the AFM growth medium during 22 days of incubation.
MATERIALS AND METHODS
Media and Growth Conditions
Cultures of F. equiseti and F. acuminatum were maintained on supplemented potato dextrose agar. Spore production in the slants usually requires 5 to 7 days of growth at 28°C. Spore concentration was determined by measuring haemocytometer (5.107 conidia/ml).
Cultures were grown in a synthetic medium, which is called Armstrong Fusarium Medium (AFM) containing: 1.1 g KH2PO4, 0.4 g MgSO4 · 7H2O, 1.6 g KCl, 7.27 g KNO3, 2 ppm FeSO4 · 7H2O, ZnSO4 · 7H2O, MnSO4 · 2H2O, 1.0 mg Thiamine-HCl and 0.05 ml Tween 80 in 1 liter of distilled water [Citation[13]]. The culture medium was adjusted to pH 4.5. Cultures were incubated with agitation at 100 rpm at 28°C in 250 ml shaking flasks containing 100 ml of culture for 18 days. After the cultivation process, the cells were collected by centrifugation followed by washing twice with distilled water and kept at − 20°C.
Preparation of Cell-Free Extracts
Wet Fusarium species cells were frozen at − 20°C and thawed overnight at + 4°C and then suspended in a 20 mM phosphate buffer, pH 7.4 containing polypropylene glycol-1200 in a volume equal to 1.5 times its weight. A 600 µl cell suspension was ground in 1.5 ml plastic vials with 0.8 g of glass beads (0.5 mm Φ) for 10 min. Cell debris was removed by centrifugation at 15000 rpm for 15 min.
Assay Methods in Cell-Free Extracts
SOD activity was measured in cell free extracts by the method of Crosti based on the inhibitory effect of SOD on the spontaneous autoxidation of 6-OHDA at 490 nm [Citation[14]]. One IU is defined as the amount of SOD required to inhibit the initial rate of 6-OHDA autoxidation by 50%.
Catalase activitiy was determined in cell free extracts by the method of Aebi [Citation[15]]. The decomposition of H2O2 followed directly by the decrease in extinction at 240 nm and 37°C.
GSH-Px activity was determined with Ransel kits by using consecutive glutathione reductase reaction. The reaction was monitored by oxidation of NADPH followed at 340 nm and 37°C. This assay, based on the method of Paglia and Valentine, requires cumene hydroperoxide as a substrate [Citation[16]].
Vitamin C concentrations were immediately determined. Vitamin C concentrations were determined by the 2,4-dinitrophenylhydrazine [Citation[17]].
Total sialic acid was determined using a spectrophometric periodate-resorcinol method for the measurement of TSA [Citation[18]].
Lipid peroxidation was estimated based on thiobarbituric acid (TBA) reactivity. Samples were evaluated for malondialdehyde (MDA) production using a spectrophotometric assay for TBA. The extinction coefficient at 532 nm of 153 000 M−1 cm−1 for the chromophore was used to calculate the MDA-like TBA produced [Citation[19]].
The protein content was determined by the method of Lowry et al. using bovine serum albumin as Standard [Citation[20]].
Statistical Analysis
The Tukey test, for multiple comparison, was used for statistical significance analyses. The values are the mean of three separate experiments. Also comparisons were name with Pearson's correlation for each substrate and or enzyme between the five different carbon and nitrogen sources at each given incubation time.
RESULTS
The variations of total sialic acid, LPO level and antioxidant enzyme activities of F. equiseti and F. acuminatum were determined in cell extracts when yeast extract was used as a nitrogen source in the range of 5–35 g/L concentration. However, F. equiseti didn't grow in 35 g/L yeast extract supplemented medium.
As shown in , antioxidant enzymes, SOD and CAT activities in filamentous fungus F. equiseti increased for all yeast extract concentrations used in the initial days of cultivation, reaching a maximum at day 12, after which the activities began to decline (p < 0.01). The maximum SOD and CAT activities tended to increase with raises of yeast extract concentration up to 25 g/L where they were determined to be 78.6±0.96 and 312.7±5.6 IU/mg.
Figure 1 Variations of SOD activity in F. equiseti (a) and F. acuminatum (b) depending on the incubation period in medium containing; 5 g/L (—▪—), 10 g/L (—•—), 15 g/L (—▴—), 25 g/L (—▾—), 35 g/L (—♦—) yeast extract concentrations. The values are the mean±SEM for experiments of three separate experiments.
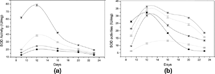
Figure 2 Variations of CAT activity in F. equiseti (a) and F. acuminatum (b) depending on the incubation period in medium containing; 5 g/L (—▪—), 10 g/L (—•—), 15 g/L (—▴—), 25 g/L (—▾—), 35 g/L (—♦—) yeast extract concentrations. The values are the mean±SEM for experiments of three separate experiments.
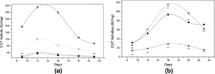
The enzyme activities were qualitatively and quantitatively different in the F. acuminatum for all yeast extract concentrations tested. SOD and CAT activities in F. acuminatum significantly increased with the rise of yeast extract concentration up to 10 g/L (p < 0.01) and maximum activities were observed at this concentration as 36.3±0.54 and 115.3±2.19 IU/mg on the 12th day of incubation (, ). But, higher than 10 g/L yeast extract concentrations had a inhibitory effect on the enzymes in cultures growth medium (p < 0.01). SOD activities were reached a maximum at the 12th day in all yeast extract concentrations used and prolongation of incubation led to a decrease of activity (p < 0.01). However, incubation days in which the maximum CAT activities observed shifted to 16th day. In addition; maximum SOD and CAT activities in F. equiseti were significantly higher in comparison to F. acuminatum had (p < 0.01).
Other H2O2 scavenger enzyme, GSH-Px activities in F. equiseti were decreased significantly with respect to increase in yeast extract concentration of the culture medium (r = − 0.442, p < 0.05) (, ). However, GSH-Px activity in F. acuminatum tended to increase as the yeast extract concentration increased (r = 0.814, p < 0.01). The maximum GSH-Px activities of F. equiseti and F. acuminatum were determined to be 5.06±0.04 and 4.74±0.09 IU/mg, respectively.
Figure 3 Variations of GSH-Px activity in F. equiseti (a) and F. acuminatum (b) depending on the incubation period in medium containing; 5 g/L (—▪—), 10 g/L (—•—), 15 g/L (—▴—), 25 g/L (—▾—), 35 g/L (—♦—) yeast extract concentrations. The values are the mean±SEM for experiments of three separate experiments.
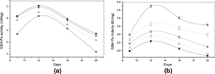
Figure 4 Variations of ascorbate level in F. equiseti (a) and F. acuminatum (b) depending on the incubation period in medium containing; 5 g/L (—▪—), 10 g/L (—•—), 15 g/L (—▴—), 25 g/L (—▾—), 35 g/L (—♦—) yeast extract concentrations. The values are the mean±SEM for experiments of three separate experiments.
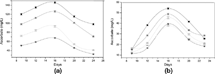
Biosynthesizes of antioxidant ascorbic acid in F. equiseti and F. acuminatum with respect to raised yeast extract concentrations were decreased markedly (r = −0.826 and −0.450) and reached the maximum points in 16 incubation days as 146.2 ± 2.48 and 53.9 ± 0.81 mg/L, respectively (p < 0.01).
As shown in , the increase of membrane bounded sialic acid level in F. equiseti and F. acuminatum, depending on the yeast extract concentration, showed positive correlation with the significant rise of SOD (r = 0.839 and 0. 7.55; p < 0.01 ) and CAT activities (r = 0.808 and 0. 417; p < 0.01). The maximum sialic acid levels activities of F. equiseti and F. acuminatum have been determined in medium containing 25 g/L and 10 g/L yeast extract as 8.53 ± 0.14 and 3.95 ± 0.06, respectively.
Figure 5 Variations of TSA level in F. equiseti (a) and F. acuminatum (b) depending on the incubation period in medium containing; 5 g/L (—▪—), 10 g/L (—•—), 15 g/L (—▴—), 25 g/L (—▾—), 35 g/L (—♦—) yeast extract concentrations. The values are the mean±SEM for experiments of three separate experiments.
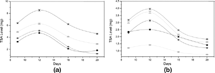
– shows that, as an indicative marker of oxidative damage, LPO levels in F. equiseti decreased during the 12 days of incubation for all used concentrations of the nitrogen source and then markedly increased (p < 0.01). On the other hand, LPO levels of F. acuminatum reached a minimum on the 12th day in all concentration used except for 5 g/L, which was increased continuously during the incubation period.
Figure 6 Variations of LPO level in F. equiseti (a) and F. acuminatum (b) depending on the incubation period in medium containing; 5 g/L (—▪—), 10 g/L (—•—), 15 g/L (—▴—), 25 g/L (—▾—), 35 g/L (—♦—) yeast extract concentrations. The values are the mean±SEM for experiments of three separate experiments.
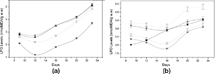
DISCUSSION
General functions of sialic acid residues at the cell surface are attributed to their physico-chemical properties such as electronegatively, hydrophilicity and relatively large size of the hydrated molecules [Citation[2], Citation[21]]. They can either mask recognition sites or serve as recognition determinants [Citation[3], Citation[22]]. That's why surface molecules of fungal cells seemingly play an important role in their membrane protection with respect to antioxidant enzyme.
In the current investigation, the highest increases in MnSOD activity value of F. equiseti was detected in medium containing 25 g/L yeast extract. In addition, besides the SOD activity, maximum CAT activity was also determined at 25 g/L yeast extract, whereas maximum GSH-Px activity was determined at 5 g/L yeast extract. However, SOD and CAT activities of F. acuminatum were increased to only up to 10–15 g/l yeast extract, whereas maximum GSH-Px activity was observed at 25 g/L yeast extract. The results show that the toxic effect of increased H2O2 by the raised MnSOD activity in F. equiseti is overcome by CAT for high levels of H2O2 and GSH-Px for low levels of H2O2.
Ascorbic acid is produced by plant and yeast cells as an important secondary metabolite [Citation[23]]. Therefore, we suspected ascorbic acid to have been formed under different culture conditions as employed in the filamentous fungus, Fusarium species. In this study, the production of ascorbic acid of F. equiseti and F. acuminatum decreased significantly with the increasing of yeast concentration from 5 to 25 g/L. The decreasing of ascorbate production probably reflects that increase in yeast extract concentration as a nitrogen source depressed the ascorbate biosynthesis.
On the other hand, the most significant decrease of membrane LPO level of F. equiseti and F. acuminatum were determined in medium containing 25 and 10 g/L yeast extract, which have maximum SOD and CAT activities. These results may indicate that both SOD and CAT enzymes are essential in protection the membrane damage [Citation[24-26]]. Moreover; in comparison to the 10 g/L yeast extract, the LPO level of F. equiseti growth in 5 g/L yeast extract decreased with increased GSH-Px activity and ascorbate production. The decreasing of membrane LPO levels may be explained by the result that the increase in peroxide radicals produced in the mitochondria escape to the cytosol where they are neutralized by a number of antioxidants including GSH-Px enzyme and ascorbate [Citation[27], Citation[29]].
In addition, besides antioxidant enzymes, TSA level in yeast extract containing medium was markedly increased opposed to the significant decrease which occurred in LPO levels. The results may indicate that these antioxidant enzymes also appeared to be involved in protecting membrane–bound sialic acids as well as membrane lipid of the fungus from exogenous ROS [Citation[30]].
According to our results, the decrease in ascorbate production, SOD, CAT, GSH-Px activities and increase in LPO levels were observed during 12–19th days. These might be explained by the fact that the aging process of the Fusarium species suppressed the antioxidant defence system due to diminished activities of these enzymes and might cause exposure of cells to more damage of radicals [Citation[31], Citation[32]]. In conclusion, antioxidant enzymes have a combined effect on the protection of biomembranes against deleterious effects of the reactive oxygen species. In addition, surface molecule level such as sialic acids of fungal cells is also indicative marker of cell membrane damage.
REFERENCES
- Iwersen, M., Vandamme-Feldhaus, V., Schauer, R. (2003). Enzymatic 4-O-acetylneuraminic acid in guinea-pig liver. Glycoconjugate Journal 15: 895–904. [CSA]
- Schauer, R. (2000). Achievements and challenges of sialic acid research. Glycoconjugate Journal 17: 485–499. [PUBMED], [INFOTRIEVE], [CSA], [CROSSREF]
- Varki, A. (1992). Diversity in the sialic acid. Glycobiology 2: 25–40. [PUBMED], [INFOTRIEVE], [CSA]
- Schauer, R. (1982). Chemistry, metabolism and biological functions of sialic acids. Adv. Carbohydr. Chem. Biochem. 40: 131–234. [PUBMED], [INFOTRIEVE], [CSA]
- Camougrand, N., Rigoulet, M. (2001). Aging and oxidative stress: studies of some genes involved both in aging and in response to oxidative stress. Respiration Physiology 128: 393–401. [PUBMED], [INFOTRIEVE], [CSA], [CROSSREF]
- Hassan, H.M., Moody, C.S. (1986). Regulation of the biosynthesis of superoxide dismutase in prokaryotes, in Superoxide and Superoxide Dismutase in Chemistry, Biology and Medicine, G. Rotilio, Ed., Elsevier Science Publishers: Amsterdam, New York, Oxford, pp. 274–279.
- Cadenas, E. (1989). Biochemistry of oxygen toxicity. Ann. Rev. Biochem. 58: 79–110. [PUBMED], [INFOTRIEVE], [CSA], [CROSSREF]
- Oberley, L.W., Oberley, T.D. (1986). Free radicals, cancer and aging, in J.E. Johnson, Jr., Ed., New York, pp. 352–371.
- Sies, H. (1986). Biochemie des oxidative stree. Angew. Chem. 98: 1061–1075. [CSA]
- Fridovich, I. (1997). Superoxide anion radical, superoxide dismutases, and related matters. J. Biol. Chem. 272: 18515–18517. [PUBMED], [INFOTRIEVE], [CSA], [CROSSREF]
- Halliwell, B., Gutteridge J.M.C. (1999). In Free Radicals in Biology and Medicine, Oxford Science Publications: Oxford, pp. 105–245.
- Kreiner, M., Harvey, L.M., Mcneil, B. (2002). Oxidative stress response of a recombinant Aspergillus niger to exogenous menadione and H2O2 addition. Enzyme and Microbial Technology 30: 346–353. [CSA], [CROSSREF]
- Scheffer, R.P., Walker, J.C. (1953). Fusarium culture media formulas. in Phytopathology 43: 116–125. [CSA]
- Crosti, N., Serudei, T., Bajer, J., Serra, A. (1987). Modification of the 6-hydroxydopamine technique for the correct determination of superoxide dismutase. J. Clin. Chem. Clin. Biochem. 25(7): 265–272. [PUBMED], [INFOTRIEVE], [CSA]
- Aebi, H. (1983). Enzymes-oxidoreductases, transferases, in Methods of Enzymatic Analysis, H.U. Bergmeyer, Ed., Verlag Chemie: Deerfield Beach, FL, Vol. 3, pp. 273–286.
- Paglia, D.E., Valentine, W.N. (1967). Studies on the quantative and qualative characterization of erythrocyte glutathione peroxidase. J. Lab. Clin. Med. 70: 158–165. [PUBMED], [INFOTRIEVE], [CSA]
- Nino, H.V.,Shaw, W. (1976). In Vitamins in Fundamental of Clinical Chemistry, N.W. Tietz, Ed., pp. 542–550.
- Spyridaki, M.H.E., Siskos, P.A. (1996). An improved spectrophotometric method for the determination of free, bound and total N-acetyneuraminic acid in biological fluids. Anal. Chim. Acta. 237: 277–285. [CSA], [CROSSREF]
- Buege, J.A., Aust, S.D. (1976). Lactoperoxidase-catalyzed lipid peroxidation of microsomal and artificial membranes. Biochimica et Biophysica Acta (BBA) – General Subjects. 444(1): 24, 192–201. [CSA], [CROSSREF]
- Lowry, O.H., Rosebrough, N.J., Farr, A.L., Randal, R.J. (1951). Protein measurement with the folin phenol reagent. J. Biol. Chem. 193: 265. [PUBMED], [INFOTRIEVE], [CSA]
- Achyuthan, K.E., Achyuthan, A.M. (2001). Comparative enzymology, biochemistry and pathophysiology of human exo-α -sialidases. Comparative Biochemistry and Physiology Part B 129: 29–64. [CSA], [CROSSREF]
- Schauer, R. (2000). Biochemistry of sialic acid diversity, in Carbohydrates in Chemistry and Biology, B. Ernst, G.W. Hart, R. Sinay, Eds., Wiley-VCH: Weinheim, Vol. 3, pp. 227–243.
- Rose, R.C., Bode, A.M. (1993). Biology of free radical scavengers; on evoluation of ascorbate. FASEB, J. 7: 1135–1139. [CSA]
- Ayar-Kayali, H., Tarhan, L. (2003). Influence of zinc and copper ions on metals transport, antioxidant system responses and membrane LPO levels of F. equiseti and F. acuminatum. Enzyme and Microbial Techno. 33: 828–835. [CSA], [CROSSREF]
- Blinski, T., Litwinska, J., Bloszcynski, M., Bajus, A. (1989). SOD deficiency and the toxicity of the products of autooxidation of polyunsaturated fatty acids in yeast. Biochim. Biyophys. Acta. 1001: 102–106. [CSA]
- Chevari, S., Andial, J., Banke, K. (1992). Free radical reactions and concern. Vopr Med. Chim. 38: 4–5. [CSA]
- Sharma, R., Morgan, P.D. (2001). Ascorbate reduces superoxide production and improves mitochondrial respiratory chain function in human fibroplasts with electron transport chain deficiencies. Mitochondrion 1: 191–198. [PUBMED], [INFOTRIEVE], [CSA], [CROSSREF]
- Haliwell, B., Gutteridge, J.M.C. (1989). Free Radicals in Biology and Medicine, in Clarendon Press: Oxford, England.
- Ayar-Kayali, H., Ozer, N., Tarhan, L. (2002). Intracellular superoxide dismutase, catalase and glutathione peroxidase activities and membrane lipid peroxide levels in Fusarium acuminatum upon environmental changes in a defined medium. Arc. Biochem. Biophys. 400: 265–272. [CSA], [CROSSREF]
- Sato, Y., Endo, T. (1999). Differential expression of sialoglycoproteins in the rat hippocampus and its changes during aging. Neurosci. Lett. 262: 49–52. [PUBMED], [INFOTRIEVE], [CSA], [CROSSREF]
- Osiewacz, H.D., Stumpferl, S. (2001). Metabolism and aging in filamentous fungus Podospora anserina. Arc. Gerontol. Geriatr. 32: 185–197. [CSA], [CROSSREF]
- Blum, J., Fridovich, I. (1985). Inactivation of glutathione peroxidase by superoxide radical. Arch. Biochem. Biophys. 240: 500–508. [PUBMED], [INFOTRIEVE], [CSA], [CROSSREF]