Abstract
Models of emphysema produced by exposing animals to cigarette smoke (CS) have potential for use in testing treatments of this disease. To better characterize development of emphysema in an animal model, male and female mice of the B6C3F1 and A/J strains were exposed to CS at 250 mg total particulate material (TPM)/m3 for 15 weeks. Emphysema was evident in both strains of mice to differing degrees of severity. The CS-induced increase in the mean linear intercept (normalized to BW) of A/J mice was 51% greater than the control value, while CS-exposed B6C3F1 had an increase of 38% in this morphometric measurement of alveolar air space enlargement. In separate experiments, female B6C3F1 mice and male A/J mice were exposed to CS for 32 weeks and 15 weeks, respectively, and were then used to test the efficacy of all trans-retinoic acid (ATRA) treatments to ameliorate emphysema lesions. Following CS exposure, the B6C3F1 mice were treated once daily for 14 days in a 3-week period by nose-only inhalation exposure to aerosols of 180 or 1,800 mg-minutes ATRA/m3. The A/J mice were treated once daily, 4 days/week, for three weeks by either intraperitoneal injection of ATRA (0.5 or 2.5 mg/kg) or inhalation exposure to ATRA (3,600 or 18,000 mg-minutes/m3). Neither the injections nor inhalation exposures of ATRA in either strain of mouse caused reversal of the emphysema. In summary, CS-induced emphysema was more severe in A/J mice than in B6C3F1 mice. Treatment with ATRA did not reverse emphysema in either strain of CS-exposed mice.
Introduction
Animal models of emphysema may provide means of testing treatments and of studying pathogenesis. To date, however, there have been only a few reports of any ameliorating or preventive effects of pharmaceuticals, biologicals, or nutritive factors on the disease process in animals. Particular studies have stemmed from the seminal work of Massaro and Massaro Citation[1] describing the reversal of elastase-induced emphysema in rats by treatment with intraperitoneal injections of ATRA. Several reports followed to support these initial findings Citation[2-7], but other studies have failed to demonstrate any beneficial effect of ATRA on emphysema Citation[8-11]. Here, we sought to treat the disease with ATRA treatment in an animal model of cigarette smoke (CS)-induced emphysema. Further, in order to avoid potential side effects from systemic delivery of the retinoid, we exposed mice with emphysema to aerosols of ATRA in expectation of better targeting of the drug to the lung.
Cigarette smoking is clearly the chief cause of COPD. Producing CS-induced emphysema, as part of the COPD syndrome, in animals represents a logical progression in modeling the disease rather than relying on inducing the disease by intratracheal instillation of elastinolytic proteinases. Moreover, mutant and transgenic animals may not recapitulate the morphogenesis or inflammatory process inherent to that induced by CS exposure. However, development of emphysema in CS-exposed animals is, as in people, a lengthy process, and decreases in disease induction times of the models are desirable for testing therapeutic effects of pharmaceutical compounds. Previously, emphysema was demonstrated in female B6C3F1 mice exposed to CS for 32 to 56 weeks Citation[12&13], but reports of strain differences in mice in susceptibility to CS-induced lung disease Citation[14-16] stimulated an investigation into model improvement by comparing strain-specific susceptibilities. Thus, in order to include results from males and to compare to another reportedly susceptible strain, male and female mice of the B6C3F1 and A/J strains were exposed to CS for a period of 15 weeks. Results herein show that A/J mice develop more severe disease in a relatively short induction period.
Materials and Methods
Animals and CS Exposure
All procedures were conducted under protocols approved by an Institutional Animal Care and Use Committee governed by the U.S. Animal Welfare Act in facilities accredited by the Association for Assessment and Accreditation of Laboratory Animal Care International. For comparing strain and gender differences, 7- to 8-week-old male and female A/J (Jackson Laboratories, Bar Harbor, ME) and B6C3F1 mice (Charles River Laboratories, Wilmington, MA) were exposed whole-body to mainstream CS from 1R3 non-filtered research cigarettes (27.1 mg TPM yield/cigarette; University of Kentucky Tobacco Research and Development Center, Lexington, KY) or to filtered air (FA, control) for 15 weeks as previously described Citation[12]Citation[17-20]. Two experiments were performed for studying the efficacy of ATRA. In the first experiment, 5- to 7-week-old female B6C3F1 mice were exposed for 32 weeks to mainstream CS from 2R1 non-filtered research cigarettes (44.1 mg TPM yield/cigarette; University of Kentucky Tobacco Research and Development Center) or to FA. In the second experiment, 7–8-week-old male A/J mice were exposed to CS from Type 1R3 cigarettes or to FA for 15 weeks. In all experiments, mice were exposed for 6 hours/day and 5 days/week at CS concentrations of approximately 250 mg TPM/m3, except for the first few days of exposures during which the mice were acclimated to CS at 100–125 mg TPM/m3. Animals were weighed periodically and were monitored twice daily for health status.
ATRA Treatment
During the last week of CS exposure, mice designated for ATRA treatment by inhalation were conditioned to nose-only exposure tubes. In the first efficacy experiment, B6C3F1 mice in each of the CS and FA exposure groups were divided into blocks and exposed to ATRA (Sigma St. Louis, MO) at 6 or 60 mg/m3 for 30 minutes/day (180 or 1,800 mg-minutes/m3) or to ethanol aerosols (sham-control, ). Animals were exposed once daily for 5 days/week during the first 2 weeks, for 4 days during the last week of treatment, and were killed on Day 5 of the last week of treatment. In the second experiment, groups of A/J mice were treated with ATRA either by intraperitoneal (i.p.) injection at 2 doses (0.5 or 2.5 mg /kg body weight [BW]) or by nose-only inhalation at 2 concentrations of 120 or 300 mg/m3 for 30 or 60 minutes/day, respectively (3,600 or 18,000 mg-minutes ATRA/m3; ). Mice in the second experiment were treated once daily for 4 days per week for 3 weeks. Two groups served as vehicle controls for ATRA (). Mice were killed approximately 1 hour after the last treatment, and tissues were collected for stereology of emphysema, evaluation of ATRA toxicity, and measurement of tissue levels of ATRA. In the second experiment, ATRA for i.p. injection was suspended in cottonseed oil at a concentration such that each mouse received approximately 0.1 mL i.p. The formulation was stored at 4°C in amber bottles and was prepared weekly. Treatment for FA- and CS-exposed mice began on Monday or Tuesday, respectively, such that individual blocks of animals were treated for only 4 days/week with 3 non-treatment days intervening.
Table 1. Experimental design for ATRA efficacy assessment in CS-exposed mice.
For inhalation exposure, mice were assumed to have a 24 g BW, a 0.2 g lung weight, a minute volume of 40 mL/minute, and fractional depositions of 0.5 to the whole respiratory tract and 0.1 to the deep lung for ATRA particle sizes of 1–2 µm mass median aerodynamic diameter (MMAD). Thus, a 30-minute exposure at 60 mg/m3, for example, was predicted to deposit 72 µg into the whole respiratory tract (1.5 mg ATRA/kg BW) and 7 µg into deep lung (36 µg ATRA/g lung tissue). Inhalation exposure to ATRA aerosols was performed essentially as previously described Citation[11]. Briefly, ATRA was solubilized in 100% ethanol and then aerosolized either with a Collison nebulizer (BGI, Inc., Waltham, MA) or with a HEART nebulizer (Model 100609, WESTMED, Inc., Tucson, AZ), dried along with dilution air to achieve target concentrations, and delivered to a cylindrical 24-port nose-only exposure chamber (In-Tox Products, Inc., Albuquerque, NM). Gravimetrically derived aerosol concentrations were measured by weighing particles collected on 25 mm Zefluor P5PL025 filters (Pall Corporation, East Hills, NY) within holders connected to one of the empty ports of the chamber. Two or more filter samples were collected during each of the daily exposures, and the mean of each day's exposure concentrations was calculated. Cascade impactor samples were collected periodically to provide aerodynamic characteristics. Control groups were exposed to aerosolized and dried ethanol (vehicle) alone for 30 minutes/day.
Studies were performed to show that the nose-only exposures were not noxious or toxic. In the first experiment, groups of 6 to 8, 12- to 14-week-old, female B6C3F1 mice were exposed to ATRA once daily for 30 minutes/day by nose-only inhalation, as described above, for 14 days out of a 3-week treatment period. The ATRA concentrations were 0 (ethanol-sham), 2, 6, 20, and 60 mg/m3 (0, 60, 180, 600, and 1,800 mg-minutes/m3, respectively). Animals were killed approximately 24 hours after the last exposure, and tissues were collected as outlined below and examined histopathologically. In the second toxicity study, a total of 20, 7–8-week-old male A/J mice were used. Eight mice each were exposed for 30 minutes to 120 mg ATRA/m3 or for 60 minutes to 300 mg ATRA/m3 (3,600 and 18,000 mg-min/m3, respectively). Four of the A/J mice were exposed for 30 minutes to dried aerosols of ethanol. At approximately 1 hour post-exposure, the ethanol-exposed A/J mice and 4 each of the A/J mice exposed to 3,600 and 18,000 mg-minutes ATRA/m3 were killed and necropsied as outlined below for measurement of ATRA concentrations in lung tissue and plasma and for histopathology. At 24 hours post-exposure, the remaining ATRA-exposed animals were killed for the same analyses.
Necropsy, Tissue Analysis, and ATRA Measurements
Mice were killed with i.p. injections of a pentobarbital-based euthanasia solution, blood was collected, and plasma was prepared. The heart and mediastinal tissues were removed from the thoracic viscera, and lungs with attached trachea were weighed. The right bronchus was ligated, and the right lung lobes were removed and frozen. Concentrations of ATRA in plasma and lung tissue from mice of the second efficacy and toxicity experiments were measured by a liquid chromatography-triple quadrupole mass spectrometry (LC/MS3) technique as previously described Citation[11]. Briefly, ether/ethyl acetate extracts of tissue and plasma homogenates were diluted with methanol/10 mM ammonium acetate and then separated on a C18 polar analysis column with an isocratic mobile phase of 90:10 methanol/water followed by MS3 analysis. Extraction efficiency from lung and plasma was approximately 100%.
The left lungs from toxicity animals were inflated with 10% neutral buffered formalin (NBF) via a tracheal cannula until the pleura was tense, while other lungs were fixed with NBF by inflation at a constant hydrostatic pressure of 25 cm for 6 hours (whole lungs for the strain-gender experiment, left lungs for the ATRA efficacy experiments). Tracheas were ligated, and lungs were fixed further by immersion in NBF for >48 hours. Samples of liver, kidney, spleen, trachea, esophagus, thyroid gland, larynx, and nasal passages were collected from some of the mice, fixed in NBF, processed, embedded in paraffin, sectioned, and stained with hematoxylin and eosin (H&E) for evaluation of ATRA toxicity. The volume of constant pressure-fixed left lungs (VL) was determined Citation[21], and left lungs were sliced in a dorsoventral-transverse direction as previously described Citation[12]. The tissues were processed, and 3 µm-thick sections from the caudal faces of the paraffin-embedded tissue blocks were made and stained with H&E. The amount of tissue shrinkage due to histoprocessing was determined as previously described Citation[11] and was used for correcting stereology parameters (below).
Sections of lungs were viewed by light microscopy to subjectively assess the degree of emphysema and any ATRA-induced reversal of the lesion. Stereology/morphometry was used to assess emphysema in the strain-gender effects experiment and to assess the effect of ATRA in a limited number of animal groups of the efficacy experiments (see Results). Fields in a systematic random sampling Citation[12] were chosen at 100 × magnification (every third field for the first efficacy experiment and every fourth field for the strain-gender comparison and second efficacy experiments). Fields were evaluated at 200 × magnification. The most commonly used morphometric indicator of alveolar air space enlargement in emphysema, the mean linear intercept (Lm, in µm), was calculated Citation[12]. The Lm was corrected for histoprocessing shrinkage by dividing it by the individual animal's linear shrinkage factor (first ATRA efficacy experiment) or the group-average linear shrinkage factor (strain-gender and second ATRA efficacy experiment). Using the VL and Lm, the total alveolar surface area (Sa, in cm2) was calculated Citation[12]. From point counting, the fractional volume densities of alveolar septa (VVspt) and alveolar air space (VVair) were also measured and multiplied by VL to yield total volume of a particular component for the lung Citation[12].
Data Analysis
Data were analyzed with SAS software (SAS Institute, Inc., Cary, NC) primarily using the general linear models version of analysis of variance (ANOVA). In the gender-strain experiment, differences in morphometry/stereology parameters were tested for interactive effects of three independent variables (strain, gender, CS exposure), and pairwise comparisons between groups were made using the least squares means procedure with the Tukey-Kramer adjustment for multiple comparisons when the ANOVA produced significant effects (p ≤ 0.05). Similarly, ANOVA and Tukey-Kramer least square means tests were performed for 2 independent variables (CS or FA exposure, ATRA or vehicle treatment) for the first ATRA efficacy experiment on B6C3F1 mice. For the second efficacy experiment on A/J mice, one-way ANOVA with exposure-treatment groups (CS-ATRA, CS-vehicle, and FA-vehicle) as the independent variables was performed for each of the inhalation and injection-treated categories. Student-Newman-Keuls (SNK) tests were used to discern pairwise differences.
Lung ATRA concentrations from groups of A/J mice in the second toxicity test were tested for differences by one-way ANOVA and SNK tests. Because of unequal variances and non-normal distribution, median plasma ATRA concentrations from the same mice were tested with the Kruskal-Wallis one-way ANOVA and Dunn's pairwise tests. Lung and plasma ATRA concentrations from the efficacy study with A/J mice had non-normal distributions and/or unequal variances. These data were first transformed by ranking and then tested using three independent variables (CS exposure, ATRA route of administration, and ATRA dose level) in a general linear models ANOVA procedure and Tukey-Kramer least squares means tests.
Results
CS Exposure Parameters
Mice used to study strain and gender influences were exposed to CS from 1R3 non-filtered cigarettes for 15 weeks at 5 days/week and 6 hours/day. The mean of average daily concentrations was 244.0 mg TPM/m3 (SD = 36.2, n = 72 days) or 97.6 ± 14.5% of the target concentration. The B6C3F1 mice for ATRA treatment were exposed for 32 weeks, 5 days/week and 6 hours/day, to CS from 2R1 non-filtered cigarettes. The mean CS concentration was 244.1 mg TPM/m3 (SD = 30.5, n = 154 days) or 97.6 ± 12.2% of the target concentration. Exposure of A/J mice used for ATRA treatments was also to CS from 1R3 cigarettes (15 weeks per group), and the mean CS concentration was 250.0 mg TPM/m3 (SD = 26.9, n = 77 days) or 100.0 ± 10.8% of the target concentration.
Effect of Mouse Strain and Gender on CS-Induced Emphysema
As seen previously Citation[11&12], CS exposure slowed the rate of BW gain in comparison to FA-exposed controls (), such that BW of CS-exposed animals at necropsy were significantly lower than controls (). Lung weights and VL were significantly greater in response to CS exposure and were also significantly affected by strain. There was no effect of an interaction between exposure and strain on these two parameters (), suggesting that CS exposure effects on lung weight and volume were similar among the strains.
Table 2. Comparison of emphysema response in B6C3F1 and A/J mice after 15 weeks of CS exposure.
Figure 1 Body weights (BW) during cigarette smoke (closed symbols) and filtered air (open symbols) exposure of male and female B6C3F1 (circles) and A/J mice (squares). Mice exposed to cigarette smoke gained BW more slowly than filtered air-exposed controls such that BW of cigarette smoke-exposed mice at the termination were significantly less than BW of filtered air-exposed mice (see ).
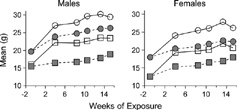
The lungs from CS-exposed mice were mottled gray-brown and pink, and some did not deflate completely upon thoracotomy. As seen previously Citation[12], lungs from CS-exposed mice had irregular enlargement of alveolar and alveolar ductal air spaces (). This “non-uniformity in the pattern of respiratory air space enlargement” has been typified as the hallmark lesion of emphysema in animal models Citation[22] and is consistent with patchy tissue destruction. Attenuation, fragmentation, blunting, and folding of alveolar septa was noted in several foci (). Neutrophils and macrophages with abundant pigmented and vacuolated cytoplasm were numerous in some foci of alveolar air spaces. Occasionally, alveolar septa were slightly thickened by infiltrates of neutrophils and mononuclear leukocytes. The interstitium around small, thin-walled blood vessels deep in the parenchyma and around parabronchiolar blood vessels and lymphatics was sometimes mildly infiltrated by lymphocytes and macrophages.
Figure 2 Left lung parenchyma from female B6C3F1 (A, B) and A/J mice (C, D) exposed to filtered air (A, C) or cigarette smoke (B, D) for 15 weeks. Note that cigarette smoke exposure in both strains is associated with widespread but irregular alveolar and alveolar ductal air space expansion. Severity of the change is qualitatively less in the cigarette smoke-exposed B6C3F1 mice. Similar changes are present in the male animals (not shown). Bars = 200 µm.
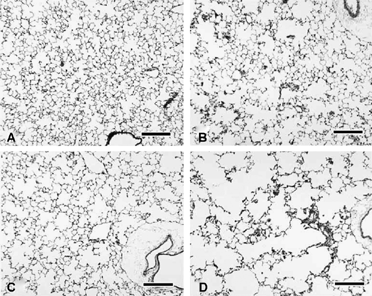
Figure 3 Alveolar septal damage (A) and inflammation (B) in the parenchyma of female A/J mice exposed to cigarette smoke for 15 weeks. Note the alveolar septal attenuation or breaks (arrowheads) and alveolar septal fragments (black arrow, A). Also present are neutrophils and vacuolated macrophages (white arrow, A). A focus of relatively severe inflammation (B) is composed of exudates of neutrophils (black arrow) and macrophages (white arrows) within alveolar air spaces. Macrophages are often vacuolated and contain pigmented cytoplasm. Bars = 20 µm.
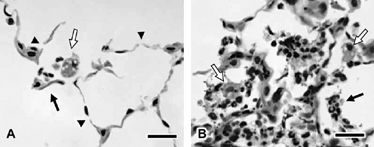
For stereologic comparison of gender and strain effects, group average numbers of microscopic fields evaluated ranged from 20.1 to 25.3. Several stereologic parameters corroborated the histopathologic evidence of emphysema in both strains and genders of CS-exposed mice following the 15-week exposure (). There was a statistically significant effect of exposure on the mean linear intercept (Lm), a crude measure of alveolar diameter, where parenchymal air spaces were enlarged in response to CS. Mouse strain also significantly affected Lm, where air spaces in A/J mice were larger regardless of CS exposure. The CS exposure also caused a significant increase in the total volume of parenchymal air space (VVair× VL) and a significant decrease in the total alveolar surface area normalized to lung volume (Sa:VL). Again, there was a significant effect of strain on both of these parameters, as might be expected in differently sized mouse strains, but the interaction between strain and exposure was not significant (). When Lm was normalized to BW (Lm:BW), however, the effect of an interaction between strain and exposure was statistically significant (). The CS-exposed A/J mice had an average increase of 51% in the Lm:BW ratio compared to the control average, while the value for CS-exposed B6C3F1 mice was only 37% greater (p < 0.05, Tukey-Kramer pairwise comparison). There was no statistically significant effect of gender on Lm or Lm:BW. The effect of gender was also absent for the remaining parameters (). Last, there was no increase in the amount of parenchymal gas-exchange tissue (total alveolar surface area [Sa] or total volume of alveolar septa [VVspt× VL]) in response to CS exposure despite the significant CS-induced increases in VL and VVair× VL (). This was consistent with increased amounts of parenchymal air space, most often in a disrupted structural arrangement (i.e., emphysema), being the primary reason for the enlarged VL, rather than parenchymal hyperplasia and/or hypertrophy causing lung enlargement as might be expected of rodent lungs in response to some inhaled toxins Citation[23].
Toxicity of Inhaled ATRA
In the first toxicity experiment, female B6C3F1 mice were exposed nose-only to ethanol (sham) or to ATRA at 60, 180, 600, or 1,800 mg-minutes/m3/day for 14 days out of a 3-week treatment period and killed approximately 24 hours later. Average concentrations of the aerosols from filter samplings were 1.99, 5.56, 20.89, and 61.81 mg ATRA/m3 (SD = 1.05, 1.23, 4.28, and 14.33, respectively from n = 14 daily mean values) for the respective target concentrations of 2, 6, 20, and 60 mg ATRA/m3. The MMAD of ATRA particles for each concentration was 3.86, 0.69, 0.45, and 0.42 µm, respectively (SD = 4.81, 0.23, 0.04, and 0.11, respectively; n = 2 each), with geometric standard deviations (σg) of 9.73, 5.06, 2.41, and 4.39 (SD = 5.76, 3.14, 0.11, and 1.36, respectively; n = 2). The mice showed no overt irritation in response to the exposure, there were no significant BW changes during the exposure period, and lung weights at necropsy did not vary significantly (data not shown). Six, 5, and 7 mice out of 8 mice per group exposed to 180, 600, and 1,800 mg-minutes ATRA/m3, respectively, had minimally mottled lungs. Histopathologic lesions in the lung consisted primarily of slight to minimal interstitial infiltrates of inflammatory cells and minimal multifocal airway epithelial degeneration with regeneration.
In the second toxicity experiment, male A/J mice were exposed once to 3,600 or 18,000 mg-minutes ATRA/m3 or to dried aerosols of ethanol and were killed approximately 1 hour or 24 hours post-exposure. Average concentrations of the aerosols were 153.16 and 333.08 mg ATRA/m3 (SD = 44.15 and 22.28, n = 3 and 6 filter samples, respectively) at the respective target concentrations at 120 and 300 mg ATRA/m3. The MMAD of ATRA particles of the high-concentration exposure was 0.37 µm with a σg of 2.61. The animals showed no irritation during the exposure, and there were no histologic lesions noted in the nasal passages, the upper and lower respiratory tracts, the liver, or the kidneys of these animals. At approximately 1 hour post-exposure, ATRA levels in the lung and plasma were measurable by LC/MS3 (). The results were variable, but the 18,000 mg-minute/m3 dose of ATRA caused significant elevation of plasma ATRA at the 1-hour time point (p = 0.0009, Kruskal-Wallis ANOVA; p < 0.05, Dunn's test). By 24 hours, plasma ATRA was not detectable above levels of control animals. Likewise, only the high-dose exposure resulted in a statistically significant elevation of lung ATRA above control values at 1 hour post-exposure (p = 0.03, 1-way ANOVA; p < 0.05, SNK test). By 24 hours, lung ATRA levels were not significantly different from the control values ().
Figure 4 Plasma and lung levels of ATRA in A/J mice of a toxicity assessment as determined by LC/MS3 after a single nose-only inhalation exposure to vehicle (ethanol sham), low-concentration (3,600 mg-min/m3) or high-concentration ATRA (18,000 mg-min/m3). Circles represent ATRA measurements for individual animals approximately 1 hour after exposure (ethanol-sham controls, low-, and high-ATRA, n = 3–5/group). Squares represent measurements at 24 hours after exposure (low- and high-ATRA, n = 4/group). Both plasma and lung values from the high-concentration exposure group (18,000 mg-minutes/m3) at the 1 hour time-point were significantly different from the ethanol sham values (p < 0.05, Dunn's and Student-Newman–Keuls pairwise tests, respectively).
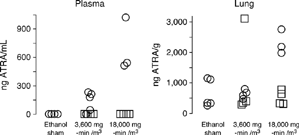
Effect of ATRA Treatment on CS-Induced Emphysema
Inhalation doses of 180 and 1,800 mg-minutes ATRA/m3 (6 and 60 mg/m3 for 30 minutes) were chosen for evaluating efficacy of ATRA for treating emphysema in CS-exposed B6C3F1 mice (). Out of a total of 15 treatment days, the mean concentrations achieved for ATRA were 6.41 and 59.90 mg /m3, or 106.8 and 99.8% of target levels, for the low and high exposure levels, respectively (SD = 0.36 and 4.51). The MMAD ranged from 0.60 to 1.03 µm for ATRA aerosol particles (low and high level, respectively; SD = 0.18 and 0.37). Based on a BW of 27 g (average terminal BW of mice from all groups), a minute-volume at 40 mL/minute, and a respiratory tract deposition fraction of 0.5, the achieved aerosol concentrations delivered an estimated 0.14 and 1.33 mg ATRA/kg BW daily to the mice at the low and high aerosol concentrations, respectively.
The B6C3F1 mice were killed 1 day after their last ATRA exposure. Lungs at necropsy and histopathologically were similar to those of the of CS-exposed mice in the gender-strain experiment. All CS-exposed mice had irregular alveolar and alveolar duct air space expansion regardless of ATRA treatment (). A morphometric/stereologic assessment was performed but limited to randomly selected animals of the CS-high-dose ATRA group, the CS-sham ethanol group, and FA-control groups of mice that were exposed either to ATRA or an aerosol of ethanol (). The CS-exposed mice had significantly decreased BW, increased lung weights, and increased VL; ATRA treatment did not significantly affect these values (). The average number of microscopic fields stereologically evaluated for emphysema was 30.2 for CS-exposed animals (SD = 3.5, n = 10) and 25.4 for FA-exposed animals (SD = 3.1, n = 10). An increase in Lm and in the total volume of parenchymal air space (VVair× VL) in both CS-exposed groups corroborated the histopathologic assessment of irregularly enlarged parenchymal air spaces in all CS-exposed groups indicating that ATRA treatment had no effect.
Table 3. Effects of CS and ATRA treatment on stereology of emphysema in female B6C3F1 mice.
Figure 5 Left lung parenchyma from female B6C3F1 mice exposed to filtered air (control; A, B, and C) or to cigarette smoke (D, E, and F) for 32 weeks followed by nose-only inhalation treatment with ethanol vehicle (A, D), 180 mg-minutes ATRA/m3 (B, E), or 1,800 mg-minutes ATRA/m3. Note irregular alveolar air space expansion in all cigarette smoke-exposed groups without any improvement following 3 weeks of ATRA treatment. Bars = 200 µm.
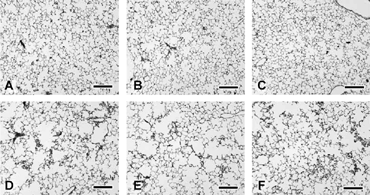
Because ATRA had no effect on reversing emphysema in the B6C3F1 mice, a second study was performed using A/J mice and injected ATRA as well as higher doses of inhaled ATRA (). The A/J mice developed more severe emphysema than B6C3F1 mice after relatively short CS-exposure periods (above) and thus allowed relatively quick assessment. Gain of BW of the A/J mice during the 15-week CS exposure period was similar to what had been observed previously with CS-exposed mice (data not shown). Relatively dramatic gains in BW during the ATRA treatment phase of previously CS-exposed mice were limited to those mice treated by injection and by nose-only exposure to aerosols of ethanol (). Mice treated with the high dose level of 18,000 mg-minutes ATRA/m3 lost an average of 2.4 g (approximately 11–12%) in BW during the treatment phase regardless of prior CS or FA exposure (). This was attributed to the development of erosive and ulcerative dermatitis on the muzzles of the high-level ATRA-exposed animals (not shown) and subsequent anorexia. Other than these findings, there were no histopathologic lesions attributable to ATRA exposure in the respiratory tracts (including nasal passages), livers, kidneys, or spleens of mice exposed to the high-dose level of ATRA.
Figure 6 Mean BW of ATRA-treated male A/J mice following 15 weeks of filtered air (open symbols) or cigarette smoke exposure (closed symbols) during inhalation exposure to (left) or injection treatment with (right) ATRA. Circles represent means for ethanol-exposed or cottonseed oil-injected sham-control mice. Triangles are for mice exposed to or injected with low concentrations of ATRA (3,600 mg-min/m3 or 0.5 mg/kg, respectively). Squares are for mice exposed to or injected with high concentrations of ATRA (18,000 mg-min/m3 or 2.5 mg/kg, respectively). As seen previously (), the BW of cigarette smoke-exposed mice is much less than that of filtered air-controls at the end of cigarette smoke exposure. Note that inhalation exposure to the high concentration of ATRA is associated with a decrease in BW of both filtered air- and cigarette smoke-exposed mice. The BW of injection-treated, cigarette smoke-exposed mice approaches that of the filtered air-controls by the end of the ATRA treatment period.
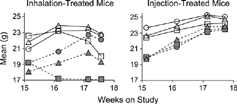
Weekly averages of daily ATRA mean concentrations as determined gravimetrically are depicted in . For the low dose-level, average ATRA aerosol concentrations were 93 to 110% of the 3,600 mg-minutes/m3 target dose. Based on an average terminal BW of approximately 21 g, minute volumes of 40 mL/minute, and a deposition fraction of 0.5, mice in the low level group likely received 3.4 mg ATRA/kg BW. Because of the development of cutaneous lesions and BW loss in the high-dose group (see above), the target concentration of aerosolized ATRA was halved mid-week during the second week of exposure by reducing the exposure time from 60 minutes to 30 minutes. Thus, achieved aerosol concentrations ranged from 92 to 95% of the target. At the halved target dose of 9,000 mg-minutes ATRA/m3, an estimate of dose for the high-level group with an average terminal BW of approximately 19 g was 9.5 mg ATRA/kg BW.
Table 4. Inhalation doses, particle sizes, and particle size distribution of aerosols for nose-only exposure of male A/J mice to ATRA.
Lung and plasma content of ATRA from five randomly selected animals per group in the aerosol-exposed and injection-treated A/J mice is depicted in . Lung tissue concentrations varied considerably in both aerosol-exposed and injection-treated mice and ranged from 167 to 2,432 ng ATRA/g. Although there was no statistically significant effect of dose, inhalation treatment resulted in significantly higher concentrations of lung ATRA than did injection treatments (p < 0.0001, Tukey-Kramer test). Interestingly, lungs from CS-exposed mice had significantly less ATRA following inhalation exposures or injection treatments than did FA-exposed control mice (p = 0.004, Tukey-Kramer test; ). Plasma concentrations were also variable and ranged from non-detectable to 317 ng ATRA/mL. Only the effect of dose was statistically significant, where mice that were treated with ATRA at both levels of aerosol exposure and injection treatment had greater plasma concentrations than sham-treated controls (p < 0.0001, ANOVA and Tukey-Kramer test; ).
Table 5. Lung and Plasma ATRA Concentrations After 3 Weeks of Inhalation or Injection Treatment in A/J Mice.
Similar to the previous experiment with B6C3F1 mice, CS-exposed A/J mice had histopathologic evidence of mild to moderate irregular alveolar air space enlargement, parenchymal damage, and alveolar inflammation (). Treatment with ATRA, whether administered by inhalation or injection, did not reverse the lesions. Morphometry and stereology was performed on a limited set of animals in order to discern any subtle effect (). Because exposure to high level ATRA (initially, 18,000 mg-minutes/m3) caused localized erosive dermatitis and subsequent BW loss () and because severe BW loss associated with caloric restriction can cause emphysema Citation[24], only the lower concentration inhalation group (CS + 3,600 mg-min ATRA/m3) was included for stereologic evaluation. In the injection-treated category, the high-dose group (CS + 2.5 mg ATRA/kg) was compared to vehicle-treated control groups (FA + cottonseed oil and CS + cottonseed oil).
Table 6. Effects of ATRA Treatment on Stereology of Emphysema in Selected Male A/J Mice Exposed to CS or FA for 15 Weeks.
Figure 7 Left lung parenchyma from male A/J mice after 15 weeks of filtered air (A, C–E) or cigarette smoke exposure (B, F–G) followed by either no treatment (A, B), sham-treatment by nose-only inhalation exposure to ethanol vehicle (C, F), nose-only exposure to low-level ATRA (3,600 mg-minutes/m3; D, G), or nose-only exposure to high level ATRA (18,000 mg-minutes/m3; E, H). Note moderate irregular alveolar air space expansion in all cigarette smoke-exposed mice without any reversal of the lesion by the 3-week ATRA treatment. Emphysema is qualitatively similar in lungs from cigarette smoke-exposed mice treated with ATRA by i.p. injection (not shown). Bars = 200 µm.
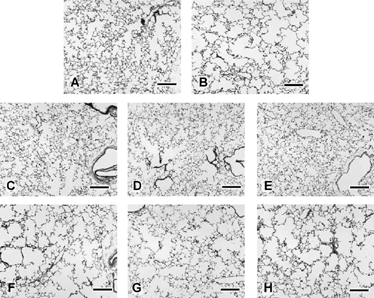
The mean number of microscopic fields evaluated during stereology of the A/J mice was 21.0 for CS-exposed animals (SD = 5.5, n = 29) and 15.5 for FA-exposed animals (SD = 2.3, n = 16). Because mice were killed at different times and their lungs were assessed by different operators (THM for the no-treatment and aerosol-treated categories; BJW for the injection-treated category), statistical analysis was divided by category (). In general, CS-exposed mice, regardless of ATRA treatment, had significantly enlarged VL in comparison to FA-exposed control mice (). With regard to alveolar air space enlargement, CS-exposed mice without any following treatment had significantly increased Lm and VVair× VL (p < 0.05 by t-test; ) in consistency to what had been demonstrated previously (). Exposure to CS caused a significant increase in Lm in all ATRA-treated animals regardless of inhalation or injection administration of the drug (). There were significant reductions in the Sa:VL ratios in mice having no treatment following CS exposure and in CS-exposed mice treated afterward with aerosols of either ATRA or ethanol. There was a reduction of the same parameter in the CS-exposed, injection-treated mice. For the most part, there were no changes in Sa or VVspt× VL in CS-exposed mice suggesting that hypertrophy or hyperplasia of parenchymal tissue did not occur to any overt extent.
Discussion
One study presented here corroborated previous reports Citation[14-16] that A/J mice are relatively more sensitive to emphysema induction by CS exposure. The reasons for this strain effect are unknown, but some of the phenotypic expressions of A/J mice related to inflammatory processes, innate and adaptive immunity, and pulmonary function may have a role in the pathogenesis. A/J mice normally have greater lung compliance than other strains of mice Citation[25]. The A/J strain also displays greater airway hyperresponsiveness to challenges with cholinergic drugs Citation[26-28] and exposure to ozone Citation[29] or ozonated products Citation[30]. Allergic airway hyperresponsiveness is also greater in A/J mice and has been linked in part to preferential activation of a nuclear factor of activated T cells (NFATc) Citation[31] and to a deficiency in the C5 component of complement Citation[32]. A/J mice also have a relatively greater pulmonary inflammatory response to nitrogen dioxide exposure Citation[33] and are more sensitive than other strains to exposure to nickel sulfate Citation[34]. A/J mice also have a deficiency in the production of type 1 inflammatory cytokines (gamma interferon, interleukin 2) in response to ectromelia virus infection which makes the strain susceptible to mousepox Citation[35]. On the other hand, gamma interferon-induced production of nitric oxide by macrophages is believed to be partly responsible for the resistance of A/J mice to mouse hepatitis virus infection Citation[36]. Whether or not any of these known phenotypes of inflammatory response would also contribute to emphysema susceptibility requires further investigation. As with any complex disease process, susceptibility to developing emphysema is likely under the control of many different genes.
The present study was also designed to discern any effect of gender on emphysema induction; however, there were no statistically significant gender influences, even though there appeared to be a greater enlargement of alveolar air space size in CS-exposed female B6C3F1 mice in comparison to their male counterparts. Unpublished results from our institution have demonstrated that females of a variety of mouse strains are indeed more susceptible than males in response to the same level of CS exposure, i.e., CS-exposed female mice develop alveolar air space enlargement in less time than males. Although pulmonary function was not measured in the present study, female mice may have had greater deposition of CS components because of comparably larger ventilatory minute-volumes per unit BW, as has been suggested, in somewhat of a dose-response effect, for greater lung cancer incidence observed in female rats exposed to CS for lifetime in comparison to males Citation[37]. Further study of gender influences is warranted. Overall, the capability to produce emphysema in mice after a relatively short CS exposure creates opportunities to study disease pathogenesis and treatment in animals within more reasonable time frames. Moreover, as has been seen recently Citation[38], various strains of mice with differing susceptibilities to CS-induced emphysema can be used to deduce some of the pathogenic mechanisms of the disease.
Emphysema induction is not dependent on the type of cigarette used for generating CS, as mice in separate experiments of the present report developed emphysema after exposure to CS from two different types of standardized research cigarettes (the 1R3 yielding ~ 27 mg TPM/cigarette and the 2R1 yielding ~ 44 mg TPM/cigarette). Likely, as has been shown in CS-exposed rats Citation[39], the particulate material within CS is responsible more than vapor components for emphysema induction. While the type of cigarette is immaterial, the amount of particulate material delivered to the pulmonary parenchyma may dictate part of an emphysema outcome. Using the same type of estimation process for determining doses of inhaled drugs such as ATRA (see Materials and Methods), mice of the present study (CS at 250 mg TPM/m3, minute volume of 40 mL/minute, fractional particle deposition of, say, 50%) would have had 1.8 mg of TPM deposited in the respiratory tract per day or approximately 90 mg/kg BW for a 20 g mouse. A person smoking 1 pack (20 cigarettes) per day of, say, 1R3 cigarettes with an estimated particle deposition fraction of 50% would have approximately 270 mg TPM deposited in the respiratory tract per day or approximately 4.2 mg/kg BW for a 65 kg person. Thus, mice of the present study may have developed emphysema in a relatively short time period because of the comparatively massive pulmonary deposition of particulate material from CS. Indeed, preliminary work at our institution indicates that lower particulate concentrations of CS will produce emphysema in exposed mice, but the time needed for lesion development is greater than that required when the high level of 250 mg TPM/m3 is used.
After the effect of ATRA treatment on elastase-induced emphysema in rats was described Citation[1], treatment of CS-exposed mice with ATRA became an intriguing concept. Initially, we used a model of emphysema in CS-exposed female B6C3F1 mice Citation[12&13] to attempt treatment of the disease by nose-only exposure to ATRA at relatively low aerosol concentrations. After this treatment regimen failed to reverse emphysema (, ) and following the comparison study of CS exposure effects on A/J and B6C3F1 mice (, ), we attempted treatment with higher doses of inhaled ATRA in CS-exposed male A/J mice. Reasoning that inhaled ATRA doses may have been inadequate during the first efficacy study, the inhalation dose at the highest aerosol concentration in the second efficacy study with the A/J mice was increased by 10-fold. Further, emphysematous mice were also treated with injections of ATRA where the low dose of 0.5 mg/kg BW was matched to that used for treating emphysematous rats Citation[1]. As in the first experiment, this injection dose, the 5-fold higher injection dose of 2.5 mg/kg, and both doses of aerosolized ATRA failed to reverse emphysema in the CS-exposed A/J mice (, ). The same study design and similar dosing was used to treat rats made emphysematous by intratracheal instillation of pancreatic elastase, and the same negative results were obtained Citation[11].
The reason for the failure is unknown. Lack of a sufficient dose was suspected in the first efficacy study with aerosolized ATRA and emphysematous B6C3F1 mice, but ATRA levels in the lung tissue and plasma of A/J mice in the second study were measured to confirm sufficient delivery to the respiratory tract. Lung tissue and plasma ATRA levels in the treated A/J mice were markedly variable (), and analytical results were not as conclusive as those seen previously in emphysematous rats treated with ATRA aerosols and injections Citation[11]. Statistical analysis revealed that aerosol administration delivered more ATRA to the lung than did i.p. injection. This was not unexpected, given that a single exposure of mice to ATRA at 9,000 mg-minutes/m3 (the adjusted dose of the high-level group after development of epidermal toxicity) was estimated to deposit 9.5 mg ATRA/kg BW into the respiratory tract, while the injected high-dose of ATRA was only 2.5 mg/kg. However, the design of the present study was not optimized for evaluation of pharmacokinetics, and although measurement of ATRA in lung and plasma within 15 minutes of treatment would have likely given more definitive results Citation[7], we have demonstrated here and previously Citation[11] that inhalation administration is an effective means of delivery of ATRA to lung tissue. Notably, the levels of ATRA achieved in plasma and lungs of mice of the present study are comparable to those reported by others Citation[7].
Interestingly, there was a statistically significant effect of prior CS exposure on lung ATRA concentrations, where CS-exposed animals had less ATRA in their lungs than ATRA-exposed FA control animals (). Cigarette smoke-induced changes in pulmonary function or structure may have caused changes in the kinetics of ATRA absorption, distribution, or biotransformation resulting in decreased lung levels in comparison to FA-exposed controls. This might be of concern when using inhaled retinoids or other compounds in humans with compromised lung function or pulmonary inflammation. However, lower quantities of ATRA in the lung were not seen in ATRA aerosol-treated rats with elastase-induced emphysema Citation[11]. Qualitative differences between the morphology of CS-induced emphysema of mice of this report and elastase-induced emphysema in rats of the previous study Citation[11] were not readily apparent, except that elastase caused more severe damage with less residual inflammation. Perhaps the inflammatory component of CS-induced emphysema was partly responsible for the difference between the emphysematous mice and rats in lung levels of inhaled ATRA.
Further investigation of the pharmacokinetics and pharmacodynamics of inhaled retinoids is warranted. Injection treatments at the high dose of 2.5 mg ATRA/kg BW also failed to ameliorate emphysema (), so it is only speculative that aerosol delivery to inflamed and emphysematous lungs resulted in enhanced clearance or biotransformation of the drug to ineffective metabolites. Interestingly, retinoic acids and other retinoids have proinflammatory activity including induction of cytokines and chemokines Citation[40-43] especially in emphysematous lungs Citation[10]. Retinoids also can induce metalloproteinase activity Citation[44]. On the other hand, retinoids also have antiinflammatory activity, such as inhibition of degranulation of polymorphonuclear leukocytes Citation[45]. The complex inflammatory milieu within emphysematous lungs may not be conducive for regenerative effects of ATRA treatment, if such treatment exacerbates inflammatory cell infiltration, synthesis and release of proinflammatory cytokines, and release of proteolytic enzymes. A better understanding of the effects of ATRA in inflamed lung tissue is necessary.
Animal models of CS-induced emphysema are increasingly being used to test efficacy of pharmaceuticals to prevent or treat COPD. Examples include prevention of emphysema development or pulmonary inflammation in guinea pigs and mice treated with phosphodiesterase-4 inhibitors Citation[46-50], metalloproteinase inhibitors Citation[15]Citation[51-53], human α1-proteinase inhibitor/antitrypsin Citation[54&55], and a synthetic serine proteinase inhibitor Citation[56]. Any of these treatments have some probability of successfully ameliorating ongoing pulmonary inflammation and preventing further tissue damage in people, whether or not such damage results from exacerbation of the disease process, and prevention of such processes continues to be a noble goal. However, reversal of previous damage to lung parenchyma, i.e., a truly regenerative process, remains to be seen with any of these compounds and also, arguably, with retinoid therapies. Models of emphysema in CS-exposed animals will likely continue to be a good way to discover therapies that could regenerate lung tissue.
Acknowledgments
The authors wish to thank the excellent technical staff for production of this manuscript including D. Esparza, S. Lucas, K. Breese-Bennett, D. Kracko, W. Blackwell, and the Aerosol Science and Technical Communications Units. The authors are also indebted to Dr. J. McDonald for insight on ATRA chemical analysis.
References
- Massaro G D, Massaro D. Retinoic acid treatment abrogates elastase-induced pulmonary emphysema in rats.Nat Med 1997; 3(6):675–677. [PUBMED], [INFOTRIEVE], [CSA]
- Belloni P N, Garvin L, Mao C P, Bailey-Healy I, Leaffer D. Effects of all-trans-retinoic acid in promoting alveolar repair.Chest 2000; 117(5 suppl 1):235S–2341S. [PUBMED], [INFOTRIEVE], [CSA], [CROSSREF]
- Belloni P (2001) . In Effects of retinoid agonists in experimental animals, Abstracts of Presentations, First Siena International Conference on Animal Models of Chronic Obstructive Pulmonary Disease, SienaItaly, Sep. 30–Oct. 2, 2001; Università di Siena: Certosa di Pontignano, .
- Mao C P, Bailey-Healy I, Gater P R, Belloni P. Effects of RO444753, a synthetic retinoid agonist, on morphologic and lung function changes in a rat model of emphysema.Am J Respir Crit Care Med 2002; 165(8):A825. [CSA]
- Tepper J, Pfeiffer J, Aldrich M, Tumas D, Kern J, Hoffman E, McLennan G, Hyde D. Can retinoic acid ameliorate the physiologic and morphologic effects of elastase instillation in the rat?Chest 2000; 117(5 suppl 1):242S–244S. [PUBMED], [INFOTRIEVE], [CSA], [CROSSREF]
- Umbehr J J, Li T, Rosales C, Purewall D K, Castellani W, Latkovich P, Baybutt R, Molteni A. Treatment with dietary retinoic acid protects cigarette smoke-exposed rats from the development of pulmonary emphysema and of chronic bronchitis.FASEB J 2002; 16(4–5):A626. [CSA]
- Hind M, Maden M. Retinoic acid induces alveolar regeneration in the adult mouse lung.Eur Respir J 2004; 23:20–27. [PUBMED], [INFOTRIEVE], [CSA], [CROSSREF]
- Lucey E C, Goldstein R H, Breuer R, Rexer B N, Ong D E, Snider G L. Retinoic acid does not affect alveolar septation in adult FVB mice with elastase-induced emphysema.Respiration 2003; 70(2):200–205. [PUBMED], [INFOTRIEVE], [CSA], [CROSSREF]
- Nishi Y, Boswell V, Ansari T, Piprawala F, Satchi S, Page C P. Elastase-induced changes in lung function: relationship to morphometry and effect of drugs.Pulm Pharmacol Ther 2003; 16(4):221–229. [PUBMED], [INFOTRIEVE], [CSA], [CROSSREF]
- Fujita M, Ye Q, Ouchi H, Nakashima N, Hamada N, Hagimoto N, Kuwano K, Mason R J, Nakanishi Y. Retinoic acid fails to reverse emphysema in adult mouse models.Thorax 2004; 59(3):224–230. [PUBMED], [INFOTRIEVE], [CSA], [CROSSREF]
- March T H, Cossey P Y, Esparza D C, Dix K J, McDonald J D, Bowen L E. Inhalation administration of all-trans-retinoic acid for treatment of elastase-induced pulmonary emphysema in Fischer 344 rats.Exp Lung Res 2004; 30(5):383–404. [PUBMED], [INFOTRIEVE], [CSA], [CROSSREF]
- March T H, Barr E B, Finch G L, Hahn F F, Hobbs C H, Ménache M G, Nikula K J. Cigarette smoke exposure produces more evidence of emphysema in B6C3F1 mice than in F344 rats.Toxicol Sci 1999; 51(2):289–299. [PUBMED], [INFOTRIEVE], [CSA], [CROSSREF]
- March T H, Barr E B, Finch G L, Nikula K J, Seagrave J C. Effects of concurrent ozone exposure on the pathogenesis of cigarette smoke-induced emphysema in B6C3F1 mice.Inhal Toxicol 2002; 14:1187–1213. [PUBMED], [INFOTRIEVE], [CSA], [CROSSREF]
- Shapiro S D. Animal models for COPD.Chest 2000; 117(5, suppl 1):223S–227S. [PUBMED], [INFOTRIEVE], [CSA], [CROSSREF]
- Shapiro S D. Animal models for chronic obstructive pulmonary disease.Am J Respir Cell Mol Biol 2000; 22:4–7. [PUBMED], [INFOTRIEVE], [CSA]
- Fitzgerald M F, Spicer D, Fox C, Kobayashi D K, Shapiro S. Effect of acute and chronic exposures to cigarette smoke in two strains of mice. Efficacy of a matrix metalloproteinase (MMP) inhibitor, BAY 15-7496, on emphysema development.Am J Respir Crit Care Med 2002; 165(8):A824. [CSA]
- Finch G L, Nikula K J, Chen B T, Barr E B, Chang I Y, Hobbs C H. Effect of chronic cigarette smoke exposure on lung clearance of tracer particles inhaled by rats.Fundam Appl Toxicol 1995; 24:76–85. [PUBMED], [INFOTRIEVE], [CSA], [CROSSREF]
- Finch G L, Lundgren D L, Barr E B, Chen B T, Griffith W C, Hobbs C H, Hoover M D, Nikula K J, Mauderly J L. Chronic cigarette smoke exposure increases the pulmonary retention and radiation dose of 239Pu inhaled as 239PuO2 by F344 rats.Health Phys 1998; 75(6):597–609. [PUBMED], [INFOTRIEVE], [CSA]
- Chen B T, Bechtold W E, Barr E B, Cheng Y S, Mauderly J L, Cuddihy R G. Comparison of cigarette smoke exposure atmospheres in different exposure and puffing modes.Inhal Toxicol 1989; 1:331–347. [CSA]
- Chen B T, Bechtold W E, Mauderly J L. Description and evaluation of a cigarette smoke generation system for inhalation studies.J Aerosol Med 1992; 5:19–30. [CSA]
- Scherle W. A simple method for volumetry of organs in quantitative stereology.Mikroskopic 1970; 26:57–60. [CSA]
- Snider G L, Kleinerman J, Thurlbeck W M, Bengali Z H. The definition of emphysema.Am Rev Respir Dis 1985; 132:181–185. [CSA]
- Kodavanti U P, Costa D L, Bromberg P A. Rodent models of cardiopulmonary disease: their potential applicability in studies of air pollutant susceptibility.Environ Health Perspect 1998; 106(suppl 1):111–130. [PUBMED], [INFOTRIEVE], [CSA]
- Sahebjami H. Nutrition and lung structure and function.Exp Lung Res 1993; 19(2):105–124. [PUBMED], [INFOTRIEVE], [CSA]
- Reinhard C, Eder G, Fuchs H, Ziesenis A, Heyder J, Schulz H. Inbred strain variation in lung function.Mamm Genome 2002; 13:429–437. [PUBMED], [INFOTRIEVE], [CSA], [CROSSREF]
- De Sanctis G T, Merchant M, Beier D R, Dredge R D, Grobholz J K, Martin T R, Lander E S, Drazen J M. Quantitative locus analysis of airway hyperresponsiveness in A/J and C57BL/6J mice.Nat Genet 1995; 11:150–154. [PUBMED], [INFOTRIEVE], [CSA], [CROSSREF]
- De Sanctis G T, Singer J B, Jiao A, Yandava C N, Lee Y H, Haynes T C, Lander E S, Beier D R, Drazen J M. Quantitative trait locus mapping of airway responsiveness to chromosomes 6 and 7 in inbred mice.Am J Physiol 1999; 277:L1118–L1123 ( Lung Cell Mol Physiol 21). [PUBMED], [INFOTRIEVE], [CSA]
- Ewart S L, Kuperman D, Schadt E, Tankersley C, Grupe A, Shubitowski D M, Peltz G, Wills-Karp M. Quantitative trait loci controlling allergen-induced airway hyperresponsiveness in inbred mice.Am J Respir Cell Mol Biol 2000; 23(4):537–545. [PUBMED], [INFOTRIEVE], [CSA]
- Zhang L-Y, Levitt R C, Kleeberger S R. Differential susceptibility to ozone-induced airways hyperreactivity in inbred strains of mice.Exp Lung Res 1995; 21:503–518. [PUBMED], [INFOTRIEVE], [CSA]
- Rohr A C, Shore S A, Spengler J D. Repeated exposure to isoprene oxidation products causes enhanced respiratory tract effects in multiple murine strains.Inhal Toxicol 2003; 15:1191–1207. [PUBMED], [INFOTRIEVE], [CSA]
- Keen J C, Sholl L, Wills-Karp M, Georas S N. Preferential activation of nuclear factor of activated T cells C correlates with mouse strain susceptibility to allergic responses and interleukin-4 gene expression.Am J Respir Cell Mol Biol 2001; 24:58–65. [PUBMED], [INFOTRIEVE], [CSA]
- Karp C L, Grupe A, Schadt E, Ewart S L, Keane-Moore M, Cuomo P J, Kohl J, Wahl L, Kuperman D, Germer S, Aud D, Peltz G, Wills-Karp M. Identification of complement factor 5 as a susceptibility locus for experimental allergic asthma.Nat Immunol 2000; 1(3):221–226. [PUBMED], [INFOTRIEVE], [CSA], [CROSSREF]
- Kleeberger S R, Zhang L-Y, Jakab G J. Differential susceptibility to oxidant exposure in inbred strains of mice: nitrogen dioxide versus ozone.Inhal Toxicol 1997; 9:601–621. [CSA], [CROSSREF]
- Wesselkamper S C, Prows D R, Biswas P, Willeke K, Bingham E, Leikauf G D. Genetic susceptibility to irritant-induced acute lung injury in mice.Am J Physiol 2000; 279:L575–L582 ( Lung Cell Mol Physiol 3)., , [CSA]
- Karupiah G. Type 1 and type 2 cytokines in antiviral defense.Vet Immunol Immunopathol 1998; 63(1–2):105–109. [PUBMED], [INFOTRIEVE], [CSA], [CROSSREF]
- Pope M, Marsden P A, Cole E, Sloan S, Fung L S, Ning Q, Ding J W, Leibowitz J L, Phillips M J, Levy G A. Resistance to murine hepatitis virus strain 3 is dependent on production of nitric oxide.J Virol 1998; 72(9):7084–7090. [PUBMED], [INFOTRIEVE], [CSA]
- Mauderly J L, Gigliotti A P, Barr E B, Bechtold W E, Belinsky S A, Hahn F F, Hobbs C A, March T H, Seilkop S K, Finch G L. Chronic inhalation exposure to mainstream cigarette smoke increases lung and nasal tumor incidence in rats.Toxicol Sci 2004; 81:280–292. [PUBMED], [INFOTRIEVE], [CSA], [CROSSREF]
- Guerassimov A, Hoshino Y, Takubo Y, Turcotte A, Yamamoto M, Ghezzo H, Triantafillopoulos A, Whittaker K, Hoidal J R, Cosio M G. The development of emphysema in cigarette smoke exposed mice is strain dependent.Am J Respir Crit Care Med 2004; 170(9):929–931. [CSA], [CROSSREF]
- Huber G L, Davies P, Zwilling G R, Pochay V E, Hinds W C, Nicholas H A, Mahajan V K, Hayashi M, First M W. A morphologic and physiologic bioassay for quantifying alterations in the lung following experimental chronic inhalation of tobacco smoke.Bull Eur Physiopathol Respir 1981; 17:269–327. [PUBMED], [INFOTRIEVE], [CSA]
- Harant H, de Martin R, Andrew P J, Foglar E, Dittrich C, Lindley I J. Synergistic activation of interleukin-8 gene transcription by all-trans-retinoic acid and tumor necrosis factor-alpha involves the transcription factor NF-kappaB.J Biol Chem 1996; 271(43):26954–26961. [PUBMED], [INFOTRIEVE], [CSA], [CROSSREF]
- Zhu L, Bisgaier C L, Aviram M, Newton R S. 9-cis retinoic acid induces monocyte chemoattractant protein-1 secretion in human monocytic THP-1 cells.Arterioscler Thromb Vasc Biol 1999; 19(9):2105–2111. [PUBMED], [INFOTRIEVE], [CSA]
- Sarkar S A, Sharma R P. Expression of selected apoptosis related genes, MIF, IGIF and TNF alpha, during retinoic acid-induced neural differentiation in murine embryonic stem cells.Cell Struct Funct 2002; 27(2):99–107. [PUBMED], [INFOTRIEVE], [CSA], [CROSSREF]
- Ninomiya M, Kiyoi H, Ito M, Hirose Y, Ito M, Naoe T. Retinoic acid syndrome in NOD/scid mice induced by injecting an acute promyelocytic leukemia cell line.Leukemia 2004; 18(3):442–448. [PUBMED], [INFOTRIEVE], [CSA], [CROSSREF]
- Shibakura M, Niiya K, Kiguchi T, Shinagawa K, Ishimaru F, Ikeda K, Namba M, Nakata Y, Harada M, Tanimoto M. Simultaneous induction of matrix metalloproteinase-9 and interleukin 8 by all-trans retinoic acid in human PL-21 and NB4 myeloid leukaemia cells.Br J Haematol 2002; 118(2):419–425. [PUBMED], [INFOTRIEVE], [CSA], [CROSSREF]
- Fumarulo R, Conese M, Riccardi S, Giordano D, Montemurro P, Colucci M, Semeraro N. Retinoids inhibit the respiratory burst and degranulation of stimulated human polymorphonuclear leukocytes.Agents Actions 1991; 34(3–4):339–344. [PUBMED], [INFOTRIEVE], [CSA], [CROSSREF]
- Fitzgerald M F, Spicer D, Lisle H, Bowyer N, Sturton R G, Minshall D, Meshi B, Ionescu D, Shrad M T, Hogg J C. Efficacy of the PDE 4 inhibitor, BAY 19-8004, in a smoke-induced model of pulmonary inflammation in the guinea pig.Am J Respir Crit Care Med 2001; 163(5):A905. [CSA]
- Sturton G, Fitzgerald M. Phosphodiesterase 4 inhibitors for the treatment of COPD.Chest 2002; 121:192S–196S. [PUBMED], [INFOTRIEVE], [CSA], [CROSSREF]
- Fitzgerald M F, Spicer D, Henning R. Efficacy of the PDE4 inhibitor, BAY 19-8004 and a steroid in tobacco smoke-induced models of pulmonary inflammation in the mouse.Am J Respir Crit Care Med 2003; 167(7):A91. [CSA]
- Fox J C, Spicer D, Henning R, Meshi B, Shrad M T, Hogg J C, Fitzgerald M F. Efficacy of the PDE4 inhibitor, BAY 19-8004, in tobacco smoke models of COPD in the guinea pig.Am J Respir Crit Care Med 2003; 167(7):A91. [CSA]
- Pruniaux M P, Xu J, Bertrand C P, Shapiro S D. Efficacy of a selective phosphodiesterase 4 inhibitor, CI-1044, on cigarette smoke-induced emphysema development in mice.Am J Respir Crit Care Med 2003; 167(7):A874. [CSA]
- Churg A, Zay K, Shay S, Xie C, Shapiro S D, Hendricks R, Wright J L. Acute cigarette smoke-induced tissue breakdown requires both neutrophils and macrophage metalloelastase in mice.Am J Respir Cell Mol Biol 2002; 27:368–374. [PUBMED], [INFOTRIEVE], [CSA]
- Selman M, Cisneros-Lira J, Gaxiola M, Ramírez R, Kudlacz E M, Mitchell P G, Pardo A. Matrix metalloproteinases inhibition attenuates tobacco smoke-induced emphysema in guinea pigs.Chest 2003; 123(5):1633–1641. [PUBMED], [INFOTRIEVE], [CSA]
- Fitzgerald M F, Spicer D, Woodman P, Weedon J, Fox J C. A comparison of the effects of the MMP inhibitor, BAY 15-7496 on pulmonary inflammation induced by multiple exposures to tobacco smoke in mice and guinea pigs.Am J Respir Crit Care Med 2004; 169(7):A470. [CSA]
- Dhami R, Gilks B, Xie C, Zay K, Wright J L, Churg A. Acute cigarette smoke-induced connective tissue breakdown is mediated by neutrophils and prevented by α1-antitrypsin.Am J Respir Cell Mol Biol 2000; 22:244–252. [PUBMED], [INFOTRIEVE], [CSA]
- Churg A, Wang R D, Xie C, Wright J L. α-1-Antitrypsin ameliorates cigarette smoke-induced emphysema in the mouse.Am J Respir Crit Care Med 2003; 168:199–207. [PUBMED], [INFOTRIEVE], [CSA], [CROSSREF]
- Wright J L, Farmer S G, Churg A. Synthetic serine elastase inhibitor reduces cigarette smoke-induced emphysema in guinea pigs.Am J Respir Crit Care Med 2002; 166:954–960. [PUBMED], [INFOTRIEVE], [CSA], [CROSSREF]