101. Clinical Use of XMR in the Diagnosis and Treatment of Congenital Heart Disease
Reza F. Razavi, MD,1 Derek L. G. Hill, PhD,2 Stephen F. Keevil, PhD,1 Vivek Muthurangu, MD,1 Sanjeed Hegde, MD,1 Kawal S. Rhodes, PhD,1 Joop van Vaals, PhD,3 Marc Miquel, PhD,1 Michael Barnett, MD,4 Eric Rosenthal, MD,5 Shakeel Qureshi, MD,6 Robert Tulloh, MD,7 Rado Andriantsimiavona, MSc,1 David J. Hawkes, PhD,1 Edward Baker, MD,1 John Spence, BSc.8
King's College London, London, United Kingdom, Radiological Sciences, King's College London, London, United Kingdom, Philip's Medical Systems, Best, Netherlands, Anaesthetics, Guy's Hospital, London, United Kingdom, Dept. Congential Heart Disease, Guy's Hospital, London, United Kingdom, Congenital Heart Disease, Guy's Hospital, London, United Kingdom, Congenital Heart Disease, Guy's Hospital, London, United Kingdom, Radiology, Guy's Hospital, London, United Kingdom.
Introduction: We are undertaking a programme of MRI guided catheterisation of patients with congenital heart disease. We describe our facility and report on our first 8 clinical cases.
Purpose: We aim to reduce or eliminate X-ray dose, improve visualisation of relevant structures during the procedure, provide 3D imaging and flow quantification, and integrate invasive electrophysiology with MR derived motion.
Methods: Description of facility: We have installed an XMR interventional suite, comprising a 1.5T Philips Intera I/T MRI scanner and Philips Pulsera cardiac x-ray unit in the same RF and x-ray screened room. A movable table top allows patients to be easily moved between modalities in less than 60 seconds. Half of the room is outside the 5 Gauss line, permitting use of traditional instruments and devices, as well as transoesophagael ultrasound and RF ablation equipment when required. The suite includes appropriate anaesthetic and monitoring equipment with invasive pressure monitoring of catheters, and a communications system for the operators. The Philips flexible coil arrays are sufficiently radio-translucent to be left in place during x-ray imaging without any image quality degradation. The XMR suite has positive pressure air handling and filtration appropriate for a catheterisation laboratory. A comprehensive safety protocol has been drawn up to minimize possible hazards.
MR-compatible catheters (ie: non braided, and without guidewires) can be manipulated in the heart and great vessels using direct MRI visualization with the real-time and interactive imaging capabilities of the Intera system, which provide between 10 and 20 frames per second. Swan-Ganz Catheters, which have a carbon-dioxide-filled balloon at their tip can be clearly seen using these fast imaging protocols by means of passive contrast mechanisms.
Following trial runs and phantom studies, clinical studies have commenced with the approval of the Guy's Hospital Research Ethics Committee. All subjects give informed consent to undergo their treatment in this research facility.
Description of procedures: Patients are anaesthetised in the patient preparation area, and transferred to the MR table in the XMR suite, where the ECG electrodes, anaesthetic tubing and RF coils are connected. The patient is then transferred to the x-ray end of the room to gain access by means of femoral artery, femoral vein or jugular vein as appropriate. The patient is then transferred to the MR scanner for pre-intervention imaging. This comprises a 3D volume heart examination (100 slices in 8 chunks at 3 phases of the cardiac cycle), then the required ventricular function and flow studies. The interactive mode of the scanner is used at this point to identify the required image planes for subsequent catheter tracking. Catheters can then be inserted and manipulated within the magnet as required, using real time or interactive imaging. Where braided catheters with more torque or guidewires are needed for catheter manipulation, the patient can be transferred back to the x-ray table. Invasive pressure waveforms and ECG are recorded continuously under both MR and x-ray guidance, and blood gases (for saturation measurements) can be collected under MR as well as x-ray. Device implantation and RF ablation is invariably done at the x-ray end of the room due to lack of device MR compatability. Suitable devices can be imaged in MRI immediately post implantation to ensure correct placement.
Results: Eight patient studies have been performed: six diagnostic cardiac catheterisations, one device closure of atral septal defect (ASD), and one radiofrequency ablation of supraventricular tachycardia. Catheters were manipulated under MR guidance in all subjects. Phase-contrast MR derived pulmonary artery flow measurements were used along with invasive pressure measurements to calculate pulmonary vascular resistance in 5 cases. In the ASD closure case, 3D MRI was used to plan the procedure. In the RF ablation case, tagged MRI was used to assess ventricular motion pre-and post ablation. Figure shows a catheter in the right ventricle. Figure shows the ASD closure device immediately post-implantation.
Conclusions:We have used an XMR facility to carry out cardiac catheterisation on eight patients with congenital heart disease, using a mixture of left heart and right heart procedures. Catheters were manipulated under MR guidance, with the tip of the catheter visualized by means of passive contrast from a carbon dioxide balloon. As we have gained experience, we have found less need for x-ray imaging during the procedure. For the most recent diagnostic procedure, no x-ray imaging was required. Three dimensional imaging, flow quantification and myocardial motion assessment have all provided clinically useful extra information in these procedures that would not otherwise have been available.
102. MR Delivery and Tracking of Magnetically-Labeled Mesenchymal Stem Cells in Myocardial Infarction
Dara L. Kraitchman, VMD, Ph.D.,1 Parag Karmarkar, Ph.D.,1 Lawrence Hofmann, M.D.,1 Ergin Atalar, Ph.D.,1 Yuji Nakamoto,1 Richard Wahl, M.D.,1 Bennett Chin, M.D.,1 Bradley J. Martin, Ph.D.,2 Mark F. Pittenger, Ph.D.,2 Jeff W. M. Bulte, Ph.D.1
Radiology, Johns Hopkins University, School of Medicine, Baltimore, MD, USA, Osiris Therapeutics, Inc., Baltimore, MD, USA.
Introduction: Because of the limited regenerative capacity of the heart muscle, there is enormous interest in using cellular myoplasty techniques, such as mesenchymal stem cell transplantation, to limit infarct size and restore cardiac function after myocardial infarction. At present, most cellular transplantation techniques in animal models require histological analysis to determine the fate and migration of cells [1–3]. Thus, the number and location of cells delivered can be estimated only post-mortem. The recent ability to label MSCs [4] with MR-visible contrast agents and nuclear tracers should enable serial tracking and quantification of MSC transplantation non-invasively with high spatial resolution.
Purpose: We investigated the potential to deliver and track Feridex-labeled MSCs (MR-MSCs) in an animal model of myocardial infarction (MI).
Methods: Five farm pigs (20–25 kgs) were subjected to a 60-minute closed-chest left anterior descending coronary artery occlusion using cardiac catheterization techniques to create myocardial infarction followed by reperfusion. MR-MSCs were injected intramyocardially (1–20×107 MSCs, n=3) or intravenously (1–2×108 MSCs, n=2) within the first hours of reperfusion. Peripherally injected MR-MSCs were co-labeled with 111-Indium oxine and serial whole body SPECT scans were performed over the first 48 h post-injection to track redistribution with follow-up MRI (1.5 T CV/I, General Electric) and SPECT at 1 week. In addition, mongrel dogs were used for pilot studies of MR delivery of intramyocardial MSCs. Intramyocardial deliver was performed using a custom needle tipped catheter via x-ray fluoroscopy (Biocardia, Inc) and a custom active MR catheter coil equipped with a needle. MR-MSCs were detected using a fast gradient echo (FGRE) pulse sequence (6.0 ms TR; 1.6 ms TE; 20° FA; 512×512 image matrix; 5 mm slice thickness; 32 kHz BW; 28 cm FOV; and 4 NSA). Infarct size and location were determined using a delayed contrast-enhanced MRI (CE-MRI, 0.2 mmol/kg Gd-DTPA, 7.8 ms TR; 3.4 ms TE; 25° FA; 256×192 image matrix; 5 mm slice thickness; 32 kHz BW; 28 cm FOV; 2 slice averages NSA; and 250 ms TI). MR-guided delivery was performed using a FIESTA pulse sequence (4.4 ms TR; 1.3 ms TE; 45° FA; 128×128 image matrix; 8-10 mm slice thickness; 125 kHz BW; and 30 cm FOV) in combination with an interactive scan plan acquisition (i-drive, GE).
The heart was excised post-mortem and sectioned for histological staining (i.e., Prussian Blue) to detect Feridex-labeled MSCs and gamma counting for 111-In oxine validation.
Results: A total of 26 intramyocardial injections were performed with ∼2/3rds of the injections visualized by MRI. Based on CE-MRI, successful MR-MSC injections in the infarction were demonstrated (Fig. ). MR-MSCs could be detected at 1 week post-injection after intramyocardial delivery but not after peripheral delivery, which was confirmed by post-mortem analysis. Peripheral delivery resulted in a predominant pulmonary distribution of MSCs immediately after injection with redistribution to the kidney, liver, spleen, and bone marrow over 48 hrs.
Conclusions: MR tracking and delivery of MR-MSCs is feasible and represents a method for non-invasively tracking the quantity and location of MSCs after MI. In this animal model, peripheral delivery was unable to produce detectable quantities of MSCs necessary for cellular regeneration of infarcted tissue.
Figure 1. MR-MSCs lesions (arrow) in CE-MRI (left) and FGRE images (right) in the long-axis plane demonstrating successful transplantation in MI.
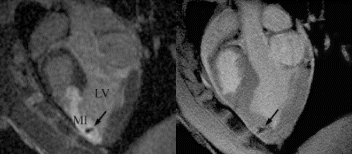
References
1. Jackson, K.A. et al., J. Clin. Investig. 2001, 107, 1395–402.
2. Wang, J.S. et al., J. Thorac. Cardiovasc. Surg. 2000, 120, 999–1005.
3. Orlic, D., et al., Nature 2001, 410, 701–705.
4. Bulte, J.W., et al., Natl Biotechnol 2001, 19, 1141–1147.
103. Serial Contrast-Enhanced MRI of Myocardial Infarction in Mice Early After Reperfusion. Importance of Early Treatment in Strategies to Control Reperfusion Injury
Zequan Yang, M.D./Ph.D.,1 Stuart S. Berr, Ph.D.,2 Marie-Claire Toufektsian, Ph.D.,1 Joel M. Linden, Ph.D.,3 Brent A. French, Ph.D.1
Biomedical Engineering, University of Virginia, Charlottesville, VA, USA, Radiology, University of Virginia, Charlottesville, VA, USA, Cardiovascular Division, University of Virginia, Charlottesville, VA, USA.
Introduction: The temporal evolution of myocardial infarction (MI) after reperfusion and its effect on left ventricular (LV) function are not well-defined. However, this information is critical in designing therapeutic strategies to reduce the effects of reperfusion injury in the setting of acute MI.
Purpose: The purpose of the current study was to use contrast-enhanced cardiac MRI to test the hypothesis that infarct size and/or cardiac function may not necessarily remain constant between 1 and 24hr post-MI.
Methods: In the MRI study, 8 mice underwent baseline scans at 3–4d prior to 45min of LAD occlusion and 24hr of reperfusion. Infarct size was serially assessed by delayed Gd-DTPA hyperenhancement at 1 and 24hr post-reperfusion. ECG-gated cardiac MRI was performed on a Varian INOVA 4.7T scanner with Magnex gradients using a birdcage quad coil (RF Design Consulting, Newberry, FL). For imaging at baseline, a 2D-cine FLASH pulse sequence was used with a flip angle=30°. For contrast-enhanced imaging after MI, heavily T1-weighted cine FLASH images (flip angle=60°) were acquired 15–20 minutes after the infusion of Gd-DTPA (0.3–0.6 mmol/kg, IP) for infarct size determination. During each session, twelve cardiac phases were acquired using. TE=3.9 ms, TR=8–10 ms, FOV=30 mm, matrix=128×128 and slice thickness=1 mm. In a second study, 16 mice were similarly infarcted to test the hypothesis that administration of a potent, anti-inflammatory agent at reperfusion might limit reperfusion injury and thus reduce the size of MI. Eight of these were untreated while 8 were treated with anti-inflammatory agent (ATL146e. a highly-selective agonist of the A2a-adenosine receptor administered at 5ug/kg, IV, 2 min before reperfusion). Infarct size in this study was assessed by TTC staining 24hr post-reperfusion.
Results: The size of MI as assessed by MRI at 1 and 24hr post-reperfusion was 32±1 and 34±1% of LV mass, respectively (p<0.05 by 2-tailed T-test, Fig. ). LV ejection fraction (EF) was dramatically reduced at 1hr and declined even further at 24hr post-reperfusion (p<0.05 for both baseline vs. 1hr and 1 vs. 24hr). Bolus injection of ATL146e significantly reduced MI size (15±2 vs. 27±1% of LV mass, p<0.05).
Conclusions: Serial assessment by contrast-enhanced cardiac MRI reveals the infarct size attains 94% of its final (24hr) volume within 1hr of reperfusion. However, the loss in EF between 1 and 24hr post-reperfusion is much greater, and cannot fully be explained by this marginal increase in infarct size. In the second study, the administration of a potent anti-inflammatory agent just prior to reperfusion caused a substantial (44%) reduction in infarct size. Taken together, the results of these two studies indicate that any therapy administered >1hr after reperfusion has the potential to reduce infarct size by no more than 6%; whereas, therapies initiated just prior to reperfusion can reduce infarct size to a far greater extent, yielding up to a 44% reduction in infarct size.
104. Molecular Imaging of Angiogenesis Associated with Athrosclerosis In Vivo with Paramagnetic Nanoparticles at 1.5T
Patrick M. Winter,1 Shelton D. Caruthers,2 Anne H. Schmieder,1 John S. Allen,1 Ralph W. Fuhrhop,1 Thomas D. Harris,3 Samuel A. Wickline,1 Gregory M. Lanza.1
Cardiology, Washington University, St. Louis, MO, USA, Philips Medical Systems, Best, Netherlands, Bristol-Myers Squibb Medical Imaging, New York, NY, USA.
Introduction: Angiogenesis is integral to the development and progression of atherosclerotic disease. αvβ3-integrin is a selective molecular epitope expressed by angiogenic endothelium (1). Conventional imaging methods can identify the late stages of atherosclerosis, i.e. lumenal stenosis, allowing the most severe narrowings to be treated surgically. The development of atherosclerotic plaques, however, occurs over a very long time providing ample opportunities for therapeutic interventions. A non-invasive imaging method that allows detection of the early development of plaques would allow monitoring of disease and possibly treatment progression.
In comparison to conventional MRI contrast agents, our site-targeted agent is designed to detect the specific biochemical markers of angiogenesis. By targeting these markers, not only can diseased and normal tissue be distinguished, but earlier detection of disease may be possible. Our targeted contrast system consists of a lipid-encapsulated, liquid perfluorocarbon nanoparticle (2) directly coupled to a selective αvβ3 ligand. This research demonstrates the feasibility of αvβ3-targeted paramagnetic nanoparticles for identification of angiogenesis in developing atherosclerotic plaques in vivo.
Purpose: To demonstrate sensitive identification of developing plaques in a rabbit model of atherosclerosis with T1-weighted signal enhancement after systemic injection of αvβ3-targeted paramagnetic nanoparticles.
Methods: Nanoparticle Preparation: The αvβ3-targeted paramagnetic nanoparticle contrast agent was produced by incorporating a paramagnetic ligand (Gd-DTPA-BOA) into the outer lipid membrane of a perflurocarbon nanoparticle covalently-complexed to an αvβ3-integrin ligand. Non-targeted nanoparticles were produced in an identical fashion, but without the αvβ3-integrin ligand.
MRI Protocol: Male New Zealand White rabbits were fed either 1% cholesterol (n=8) or standard rabbit chow (n=4) for ∼80 days. All control diet animals received αvβ3-targeted nanoparticles, while the high-cholesterol rabbits received either αvβ3-targeted (n=5) or non-targeted (n=3) nanoparticles. Transverse black-blood MRI (TR/TE=380/11 ms) of the aorta from the diaphragm to the renals was performed with a clinical 1.5T magnet (Gyroscan NT, Powertrak 6000, Philips Medical Systems, Best, Netherlands) using a quadrature birdcage neck coil. The image resolution was 250 by 250 μm in-plane with a 5 mm slice thickness. Images were collected before and after (0, 30, 60, 90 and 120 minutes) peripheral injection (ear vein) of 0.5 ml/kg body weight of nanoparticles. Expression of αvβ3 in the aortic wall was confirmed by immunohistochemistry (LM609). Regions of interest were manually drawn around the aortic wall and skeletal muscle at three levels (renals, mid-abdominal and diaphragm). The signal intensity was calculated before and after injection, normalized relative to the signal from a fiduciary marker placed within the field of view. The percent change in signal intensity after nanoparticle injection was calculated.
Results: The T1-weighted black-blood images collected before nanoparticle injection showed no gross evidence of plaque development in the aortic walls of rabbits fed either high-cholesterol or control diets (Fig. ). Two hours after αvβ3-targeted nanoparticle injection, the signal from the aortic wall of high-cholesterol rabbits was enhanced by 93±15% (Fig. ). The signal from the aortic wall of control diet rabbits showed significantly less enhancement, 30±6% (Fig. ) (p<0.005) as did the aortic wall of high-cholesterol rabbits that received non-targeted nanoparticles, 34±4% (p<0.01). No signal enhancement was observed in the muscle of any group (Cholesterol-Fed: 22±2%, Control Diet: 12±1%, Non-Targeted: 19±2%). Histology revealed endothelial αvβ3 expression in the aortic adventitia of cholesterol-fed rabbits.
Conclusion: Molecular imaging of angiogenesis associated with the early development of atherosclerosis was demonstrated noninvasively in cholesterol-fed rabbits using a clinical MRI scanner (1.5 T). These results suggest that MR molecular imaging may provide a unique tool to monitor the onset, progression or responsiveness to therapy of atherosclerotic angiogenesis. Expansion of the vasa vasorum in the aortic adventitia represented the sites of angiogenesis responsible for specific binding of targeted contrast agents, as has been suggested by Wilson, et al (3).
These experiments suggest that αvβ3-targeted paramagnetic nanoparticles can identify developing atherosclerotic plaques in vivo. The enormous paramagnetic payload of our nanoparticle (approximately 88,000 Gd3+ ions per particle) provides significant signal enhancement despite the partial volume effects inherent in site-targeted contrast agents. We can detect the effects of nanoparticles bound to relatively diffuse epitopes in an imaging voxel measuring 250 by 250 μm and 5 mm thick. These experiments utilized high resolution MRI in order to maximize the signal enhancement in the arterial wall, but even with typical clinical resolutions (∼1 mm in-plane), the nanoparticles create significant signal enhancement (2).
This novel, targeted MRI agent may allow sensitive early detection and characterization of unrecognized vascular pathology in high-risk patients.
Figure 1. T1-weighted black-blood images of aortas from rabbits fed high-cholesterol or control diets before and 2 hrs. after αvβ3-targeted nanoparticle injection.
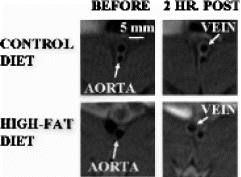
Figure 2. MRI signal intensity of aortic wall and muscle from rabbits fed high-cholesterol or control diets before and up to 2 hrs. after αvβ3-targeted nanoparticle injection.
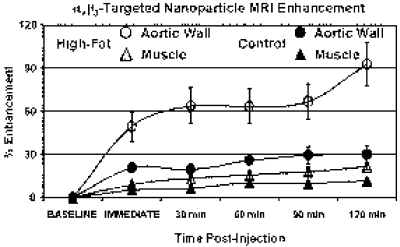
References
1). Liaw L, et al. J Clin Investig 95:713, 1995.
2). Flacke S, et al. Circulation 104:1280, 2001.
3). Wilson SH, et al. Circulation 105:415, 2002.
105. Relation of Myocardial Scarring to Clinical Risk Factors for Sudden Cardiac Death in Hypertrophic Cardiomyopathy
Heiko Mahrholdt, MD,1 Anja Wagner, MD,1 Lubna Choudhury, MD,2 Marcus Honold, MD,3 Robert O. Bonow, MD,2 Udo Sechtem, MD,3 Robert M. Judd, PhD,1 Raymond J. Kim, MD.1
Duke Cardiovascular MR Center, Duke Medical Center, Durham, NC, USA, Northwestern Medical School, Chicago, IL, USA, Robert Bosch Medical Center, Stuttgart, Germany.
The risk of sudden cardiac death (SCD) in patients with hypertrophic cardiomyopathy (HCM) is commonly estimated using established clinical predictors. Although myocardial scarring is thought to be an important anatomic component of the arrhythmogenic substrate that leads to SCD, the relation between scarring and clinical risk factors for SCD is unknown.
Cine and gadolinium contrast-enhanced MRI (ceMRI) was performed in 35 HCM patients (48+-16 yrs, 21 male) on a clinical 1.5T scanner. For each patient, the clinical risk of SCD was assessed using the following additive scale. history of cardiac arrest=4 points, sustained VT=3 points, extreme hypertrophy (max wall thickness≥30mm)=2 points, family history of SCD (≥1 first degree relative, <50 yrs)=1 point, unexplained syncope=1 point, repetitive nonsustained VT (≥2 episodes)=1 point. Scar volume was assessed by planimetry of hyperenhanced regions on ceMRI.
Scarring was present in 27 patients (77%). The average amount of scar was 10+−9% LV. Dividing patients into 5 subgroups according to scar volume (Fig. ), we found a progressive stepwise relation between scar volume and clinical risk for SCD (P<0.001 for trend). For example, in patients with scar volume >30% LV the average risk score was 5+−2 points (range 3–7) whereas in patients without scar, all 8 had a risk score of zero. Interestingly, of the 21 patients with a risk score of zero, a third (33%) had scar volume greater than 10% LV, suggesting that tissue substrate for SCD may be present in some patients without clinical risk factors.
The extent of myocardial scarring on MRI relates in a progressive stepwise fashion to the clinical risk of SCD. Whereas patients without scar do not have clinical risk factors for SCD, the absence of risk factors does not rule out substantiative amounts of scarring.
106. Assessment of Pulmonary Vein Diameters After Radiofrequency Catheter Ablation in Patients with Atrial Fibrillation Using Magnetic Resonance Imaging and Conventional X-Ray Angiography
Thorsten Dill,1 Okan Ekinci,2 Thomas Neumann,2 Heinz-Friedrich Pitschner,2 Christian W. Hamm.2
Cardiology, Kerckhoff Heart Center, Bad Nauheim, Germany, Kerckhoff Heart Center, Bad Nauheim, Germany.
Introduction: Radiofrequency catheter ablation (RFCA) of atrial myofibrils at the orifice of pulmonary veins (PV) for treating atrial fibrillation (AF) causes frequently diameter reduction (DR) due to reversible edema or permanent stenosis.
Purpose: In this study, magnetic resonance imaging (MRI) as a noninvasive technique was compared to standard x-ray angiography (angio) for quantification of pulmonary vein diameters (dia).
Method: 138 PV of 46 patients (52±10y) with AF were examined before, one day after and three months after RFCA with MRI (1.5T, Sonata, Siemens, Erlangen). A non-gated contrast enhanced FISP-3D sequence followed by a 3D reconstruction (MPR) was used. The ostium and the proximal 20 mm of all PV were evaluated and measured. Quantitative vessel analysis (Quantcor, Siemens, Erlangen) was done for the angio of 45 PVs before RFCA. These data were compared to MRI data. The dia of untreated PV were used as reference.
Results: All MRI examinations were free of complications. Mean duration: 6±2 min. The comparison of dia measured by MRI and x-ray angiography revealed a high correlation (r=0.934, p<0.001). There was no significant difference in MRI and angio dia (15.3 vs 15.0 mm). MRI measurements showed no significant change of dia at any time in untreated PV, whereas a significant DR was found in PV after RFCA (before/one day after/3 mo. follow-up (fup). 15.2±2/14.2±3/13.8 mm±3 mm, p<0.001). One day after RFCA 18 PV (13%) showed a DR >25%, 7 (5%) even >50%. On follow up 8 additional PVs showed a DR. In 6 PVs a regression of DR was found.
Conclusion: There is a high correlation of PV diameters measured by MRI and x-ray angiography. MRI proved to be accurate and reproducible for the measurement of PV diameters and allows non-invasive follow-up after RFCA for detection of pulmonary vein stenoses without the biological risk of x-ray. Diameter changes in treated PVs may occur even weeks after RFCA. Long term follow-up appears to be imperatively indicated.
107. A Comparison of Dobutamine Stress Function and Stress Perfusion MRI in 260 Patients
James F. London, Gauri Tilak, Srirama V. Swaminathan.
The Heart and Vascular Institute of Florida, Safety Harbor, FL, USA.
Introduction: Cardiac magnetic resonance is emerging as an important imaging modality for pharmacological stress testing. Using this technique one can aquire information regarding stress function, stress perfusion, or both.
Purpose: The purpose of this study was to compare the diagnostic accuracy of stress function to stress perfusion in a large group of patients undergoing dobutamine MRI stress testing.
Methods: The study population consisted of 260 patients (160 men and 100 women; aged 61.2 ± 12.2) referred for MRI stress testing. The MR imaging studies were performed on a Philips 1.5 T Intera (Philips Medical Systems, Nederland, BV). After obtaining the scouts and initial functional information the wall motion protocol was employed. The wall motion studies were performed at rest and also increased dosage of dobutamine administered in steps of 10 mcg/kg/min to a maximum of 50 mcg/kg/min. Up to 1 mg of atropine was administered if needed to achieve a target heart rate of 85% of the age predicted maximal heart rate. The wall motion protocol was a 6 slice steady state sequence called Balanced Fast Field Echo (B-FFE). This protocol was performed under breath hold with an average breath hold time of 6 sec. Slice thickness 8 mm with an inter-slice gap of less than 14 mm. Field of view (FOV) varied with patient size and was less than 450 mm. Acquisition matrix of 128×154 along frequency and phase direction respectively with a flip angle of 55° and TR/TE of 3.3/1.45 ms.
After reaching the peak target heart rate, stress perfusion imaging was performed with gadolinium (0.1 mmol/kg) using a six slice, multiple phase, Turbo Field Echo-Echo Planar Imaging (TFE-EPI) sequence. The slices were all 8 mm in thickness with an inter-slice gap of 4 mm covering the heart from apex to the base providing short axis views. EPI factor of 11 with a flip angle of 30° and a TR/TE of 12/3.5 ms were used. Bandwidth 70.4 Khz. 120 degree sat pre pulse with a delay of 200 ms. Studies were read prospectively by one experienced reader blinded to cardiac catheterization results. Patients were followed by phone conversation and chart review to see if they underwent cardiac catheterization or had cardiac events within 6 months following the cardiac MRI stress test. Stress test results were compared to cardiac catheterization data to obtain sensitivity, specificity, and diagnostic accuracy for the two modalities. Significant coronary artery disease was defined as a stenosis of greater than 50% in a major epicardial vessel.
Results: 252 of the 260 patients were able to complete the protocol. There were no serious complications. Eight patients had transient arrythmias, 2 patients had contrast extravasation, and 1 patient had a mild contrast reaction. The mean peak heart rate was 135±15.8 BPM. The mean peak SBP was 183.6±27.6 mm Hg. The mean peak rate pressure product was 24,812±4,812. 39 patients underwent cardiac catheterization within 6 months following their cardiac MRI stress test. Three of 128 (2.3) patients with normal MRI stress tests underwent percutaneous revascularization within the six month follow-up perior. There were no other cardiac events in patients with normal cardiac MRI stress tests. The sensitivity and specificity for stress wall motion was 48% and 89% respectively. The sensitivity and specificity for stress perfusion was 74% and 74% respectively. Diagnostic accuracy was 74% for stress perfusion versus 67% for stress function.
Conclusions: Dobutamine MRI stress perfusion is more accurate than dobutamine MRI stress function for predicting significant coronary artery disease.
108. Myocardial First-Pass Perfusion Imaging: A Multicenter Dose Ranging Study
Steven D. Wolff,1 Juerg Schwitter,2 Richard Coulden,3 Matthias Friedrich,4 David A. Bluemke,5 Robert W. Biederman,6 Edward T. Martin,7 Fran Kashanian,8 Thomas K. F. Foo,9 Paul E. Licato,9 Cindy R. Comeau.1
Advanced Cardiovascular Imaging, Cardiovascular Research Foundation, and Lenox Hill Hospital, New York, NY, USA, University Hospital of Zurich, Zurich, Switzerland, Papworth and Addenbrooke's Hospitals, Cambridge, United Kingdom, Franz-Volhard Klinik, Berlin-Buch, Germany, Johns Hopkins University School of Medicine, Baltimore, MD, USA, Allegheny General Hospital, Pittsburgh, PA, USA, Oklahoma Heart Institute, Tulsa, OK, USA, Berlex Laboratories, Montville, NJ, USA, GE Medical Systems, Milwaukee, WI, USA.
Introduction: Myocardial perfusion imaging is an integral part of the assessment of patients with ischemic heart disease. A number of published studies have shown that first-pass contrast-enhanced cardiac MRI is efficacious in this regard.1 Many of the initial studies were performed with low doses of gadolinium contrast (e.g. ≤0.05 mmol/kg) and gradient-recalled-echo pulse sequences. In later studies, some investigators have used higher doses of contrast in an attempt to increase the signal-to-noise ratio of the images and perhaps increase the sensitivity for detecting perfusion defects.2,3,4 More recently, a newer EPI-based perfusion pulse sequence was developed to maximize sensitivity to differences in T1 without compromising the rate of image acquisition.5 Because this sequence allows for a relatively long time between the saturation pulse and image acquisition (TI), it would be expected to have increased sensitivity to low doses of gadolinium contrast (e.g. ≤0.05 mmol/kg) as compared to sequences with shorter TI times.
Purpose: The purpose of this study is to determine the minimal efficacious dose of Magnevist for detecting coronary artery disease using an EPI-based perfusion sequence with a notched-saturation pulse.
Methods: This study was performed under IRB approval. Patients were eligible to enroll in the study if they were scheduled for cardiac catheterization, had not previously undergone cardiac by-pass graft surgery, and had no contraindications to adenosine, gadolinium contrast, or MRI. Three clinical sites administered Magnevist (gadopentate dimeglumine, Berlex Laboratories, Wayne, NJ) to 94 patients of whom 75 were evaluable. Reasons for patients not being evaluable are listed as follows. incorrect MRI protocol (6), no resting perfusion images (4), off-resonance (4), images lost before being archived (2), no QCA (1), presumed contrast extravasation (1), bad gating (1). Site 1 enrolled 40 patients of whom 34 (85%) were evaluable, Site 2 enrolled 27 patients of whom 23 (85%) were evaluable, and Site 3 enrolled 32 patients of whom 18 (56%) were evaluable. No serious adverse events were reported.
First-pass perfusion imaging was performed on a 1.5 T Signa CV/i scanner (GE Medical Systems, Milwaukee, WI) using a segmented EPI pulse sequence with a notched saturation pulse.5 Perfusion pulse sequence parameters were as follows. TR 6.6–15.8 ms, TE 1.3–2.2 ms, TI 158–211 msec, echo train length 4-8, FOV34–37×25–27 cm, matrix 128×128, and slice thickness 10 mm. Depending on the heart rate, typically 6–8 slice locations were acquired every 2 R-R intervals. Subjects were randomized to one of three doses of Magnevist. 0.05 mmol/kg (27 patients), 0.1 mmol/kg (24 patients), and 0.15 mmol/kg (24 patients). For stress perfusion imaging, contrast injection and image acquisition commenced 3 minutes following the initiation of adenosine infusion (Adenoscan, Fujisawa, Deerfield, IL. 140 ug/kg/min). Contrast was injected at 5 ml/sec and was followed with 25 ml saline flush at the same injection rate using an MRI compatible power injector. Rest perfusion images were acquired 20 minutes later with identical parameters (but with no adenosine infusion). The MR perfusion images were analysed by 4 independent blinded readers who graded the likelihood of significant coronary artery disease on a 1–5 scale. An ROC analysis was performed for each reader at each dose level.
X-ray coronary angiography was performed within 30 days of the perfusion study and served as the standard of reference. Coronary angiograms were quantitatively analyzed by a core laboratory. For this study a 70% or greater stenosis of a main epicardial coronary artery or of a moderate or large sized branch vessel was considered positive for coronary artery disease.
Results: Figure shows an example of a stress (top row) and rest (bottom row) perfusion study. The arrows point to regions of decreased signal intensity in the subendocardium, indicating the presence of flow limiting stenoses in this patient who had significant coronary artery disease on cardiac catheterization. Figure shows an ROC analysis for each of the four readers at each of the three doses of Magnevist. The average area under the ROC curve is 0.90±0.04, 0.72±0.09, and 0.83±0.06 at doses of 0.05, 0.10, and 0.15 mmol/kg, respectively. Among the three doses, there is a statistically significant difference between the ROC areas of the low and medium doses (p<0.02). However, there is no statistically significant difference between the low and high or medium and high doses.
Conclusions: For first-pass adenosine stress myocardial perfusion imaging, a dose of 0.05 mmol/kg is sufficient to detect the presence of coronary artery disease with a high degree of accuracy. Although the number of patients enrolled in this study is small, there appears to be a trend toward improved accuracy and inter-reader agreement with the lowest dose. This suggests the possibility that higher doses of contrast may be less efficacious. While the precise mechanism is unclear, one could speculate that perhaps higher doses of contrast cause more prominent susceptibility artifacts, which could mimic and/or mask real perfusion defects
References
1. Wilke NM, Jerosh-Herold M et al. 1999; 10:676–685
2. Wolff SD, Comeau CR et al. Proc. ISMRM 2000; 8:1558
3. Arai AE, Epstein FH et al. Proc. ISMRM 1999; 7:304
4. Schwitter J, Nanz D et al. Circulation 2001 May 8; 103(18):2230–2235
5. Slavin GS, Wolff SD et al. Radiology 2001; 219:258–263
Acknowledgment
This study was supported in part by Berlex Laboratories and GE Medical Systems.
109. A Survey of Patient Tolerance of MRI and SPECT Myocardial Perfusion Studies
Sven Plein, MD,1 Tim R. Jones,1 Penelope Thorley,2 F Cheater,3 C Hale,3 Mohan U. Sivananthan.1
Cardiac MRI Unit, Leeds General Infirmary, Leeds, United Kingdom, Nuclear Cardiology, Leeds General Infirmary, Leeds, United Kingdom, School of Healthcare studies, University of Leeds, Leeds, United Kingdom.
Introduction: The most commonly used imaging modality for myocardial perfusion imaging in clinical routine is Single Photon Emission Tomography (SPECT). Magnetic Resonance Imaging (MRI) assessment of myocardial perfusion offers some potential technical advantages over SPECT and could soon become a clinical alternative to SPECT. However, there are important hardware and procedural differences between MRI and SPECT, which could affect the way patients experience the two tests. For example, current MRI scanners obviously present a more confined environment, which may be uncomfortable or intolerable for patients. On the other hand, MRI perfusion scans are generally completed in one imaging session, whereas SPECT protocols conventionally require two days. In order to be clinically accepted, the tolerability of a test is as important as its technical proficiency. The development of cardiac MRI so far has focused on the technical developments and questions of patient perception of MRI versus SPECT have not yet been addressed.
Purpose: To compare the patient tolerance of MRI and SPECT myocardial perfusion studies.
Methods: We designed a patient questionnaire in collaboration with the School of Health Care studies at the local University. The questionnaire is divided into three sections, one for SPECT and MRI separately and one section for direct comparison of the two tests. The questions include overall ratings for the scans, scan comfort, space on the scanner, immediate and delayed after-effects of the scans, scan duration and perceived safety of the procedure. Patients are asked to state a preference for one test with further questions exploring the reasons for their preference.
Questionnaires were sent to 41 patients (21 male, mean age 65.4) who had both SPECT and MRI myocardial perfusion scans at our institution. Less than 3 months separated the two studies and both included an Adenosine stress examination. Nuclear scans were clinically requested and performed using a standard two-day protocol, with rest and stress studies performed on separate days. MRI scans were performed as part of a research protocol in a single session, with resting perfusion followed by stress perfusion. The MRI study also included functional and coronary artery imaging.
Results: Thirty-two of 41 patients returned questionnaires; 31 of these were completed. On direct comparison, 13 patients stated that overall they preferred the MRI examination, 9 preferred the SPECT scan and 9 expressed no preference (difference not significant). The main reason for preferring the MRI scan was the shorter scan duration (mean score 2.1 vs 1.8 on a scale from 1–4, p<0.05). Two patients preferred MRI because they developed shoulder pain during the SPECT scan, which is performed with the left arm raised above the head. Exposure to radiation was not regarded as an important disadvantage of SPECT. The main specific preference in favour of SPECT was the space on the scanner (mean 1.4 vs 1.9, p<0.5). One patient stated he would not have an MRI scan again, while no patients gave this answer for the SPECT scan. Ratings for the overall comfort of the scans were similar with a score of 3.75 for SPECT and 3.78 for MRI (scale from 1–10).
Conclusions: To our knowledge, this is the first reported assessment of patient tolerance of MRI myocardial perfusion imaging compared with the standard SPECT technique. In this study, patients rated the two tests similar, when giving an overall assessment. This indicates that MRI could be a clinically accepted alternative test to SPECT. There were some important differences between the two tests. The shorter scan duration was seen as a specific advantage of MRI. Interestingly, exposure to radiation did not affect patient's preference. The main disadvantage of MRI was the confined space on the scanner. This should be considered in future magnet design.
110. Comparison of First Pass Magnetic Resonance Perfusion and Contrast Echocardiography for the Detection of Ischemia in Patients with Coronary Artery Disease
Nidal Al-Saadi,1 Andreas Hagendorff, MD,2 Hassan Abdel-Aty, MD,1 Rainer Dietz, MD,1 Matthias G. Friedrich, MD.1
Cardiology, Franz-Volhard-Klinik, Charite, Campus Berlin-Buch, Humboldt University, Berlin, Germany, Cardiology, University of Leipzig, Leipzig, Germany.
Introduction: Myocardial perfusion can be evaluated by new techniques such as contrast echocardiography (MCE) or Magnetic Resonance (MR).
Purpose: We aimed to compare MR perfusion with MCE power Doppler harmonic imaging applying similar protocols for the first pass kinetics of a contrast agent bolus
Methods: 29 patients with coronary artery disease were examined before coronary angiography. In both, MR and MCE three similar long axes were acquired at rest and during adenosine stress (140μg/kg/min). In a cardiovascular MR system (Signa, CV/i 1.5T, GE Medical Systems) a GRE-EPI hybrid sequence was used during the first pass of a Gd-DTPA bolus (0.1 mmol/kg). MCE examinations were performed on a GE Vivid Five with three consecutive bolus injections (each 0,3 ml) of Optison@. Analyses were performed visually and semiquantitatively (upslope and maximal signal intensity in MR, DI kinetics in MCE). Both techniques were compared with coronary angiography.
Results: MR image quality was good in all segments but the very apical of the left ventricle (segment 17). Hypoperfusion could be detected in the territory of the LAD in 13 of 15 patients, in the RCA in 11 of 12 patients, and in the LCX in 9 of 10 patients. Two of 6 patients without coronary stenosis showed hypoperfusion (both were basal segments). MCE image quality was insufficient in all basal segments and posterior and lateral segments. In the territory of the LAD, MCE showed a good agreement with MR (92%) and angiography (84%)
Conclusion: MR and MCE can be used for the evaluation of myocardial perfusion. Whereas both techniques are comparable in the evaluation of the territory of the LAD, MR seems to be superior in the territories of the RCA and LCX.
111. A Combined Single-Session Analysis of Myocardial Perfusion During Adenosine Stress and of Wall Motion During High-Dose Dobutamine-Atropine Stress MRI Improves Diagnosis of Inducible Ischemia
Andreas Wahl, MD,1 Stefan Roethemeyer, MD,2 Ingo Paetsch, MD,3 Kristof Graf, MD,3 Rolf Gebker, MD,3 Holger Langreck, MD,3 Christoph Klein, MD,3 Eckart Fleck, MD,3 Eike Nagel, MD.3
Cardiology, Swiss Cardiovascular Center Bern, Bern, Switzerland, Cardiology, Heart Center Osnabrueck-Bad Rothenfelde, Bad-Rothenfelde, Germany, Cardiology, German Heart Institute Berlin, Berlin, Germany.
Introduction: With MR, both the analysis of myocardial perfusion during adenosine stress and of wall motion during dobutamine stress (DSMR) were shown highly accurate for the diagnosis of inducible myocardial ischemia. A combined single session stress protocol has not been reported so far.
Purpose: To compare the diagnostic accuracy of myocardial perfusion and of wall motion analysis in an unselected population, and to determine whether a combined single session assessment of myocardial perfusion and wall motion improves diagnosis of inducible myocardial ischemia, with conventional quantitative invasive coronary angiography serving as reference standard.
Methods: 65 consecutive patients (63±8 years; prior myocardial infarction 31%; prior percutabeous or surgical revascularization in 43% and 31%, respectively) with suspected (n=28; 43%) or known (n=37; 57%) coronary artery disease underwent a combined adenosine and dobutamine stress protocol (1.5 T Philips), prior to invasive coronary angiography. DSMR images were acquired at rest and during a standardized high-dose dobutamine-atropine protocol in 3 short-axis, a 4-, 3- and 2-chamber view (single-slice steady state free precession technique; TR/TE/flip 3.0/1.5/55). Regional wall motion was assessed using a multiple screen format, a 16 segment model, and a 4-point scoring system. A new or worsening wall motion abnormality in ≥1 segment was considered positive for ischemia. In the absence of ischemia, failure to attain 85% of age-predicted maximal heart rate was defined as non-diagnostic result. Myocardial perfusion was visually assessed during the first pass of a contrast agent bolus (0.05 mmol kg−1), from 60 dynamics (3 short axis images acquired every heartbeat; TFE-EPI hybrid sequence; pp-delay 200 ms; TR/TE/flip 3.6/12/30) during adenosine infusion (140 ug kg−1 min−1) and at rest.
Results: Significant coronary artery disease (≥50% diameter stenoses by quantitative invasive coronary angiography) was found in 77% of patients. No significant adverse effects were observed during stress testing. Sensitivity, specificity and diagnostic accuracy of wall motion analysis were 94%, 80% and 91%, respectively, with one (2%) non-diagnostic test (target heart rate not reached). These values were 86%, 73% and 83% for perfusion analysis, with one non-diagnostic test (poor image quality) and 94%, 80% and 91% for a combined approach, with 100% diagnostic tests.
Conclusions: A combined single session assessment of myocardial perfusion during adenosine stress and of wall motion during DSMR is safe and feasible, and improves diagnostic yield.
112. Color-encoded Semiautomatic Analysis For Multi-slice First-pass Magnetic Resonance Perfusion: Comparison To 99M Technetium Spect And X-ray Angiography
Holger Thiele, MD,1 Sven Plein,2 John P. Ridgway,2 Marcel Breeuwer,3 Penelope J. Thorley,2 Gerhard Schuler,4 Mohan Sivananthan.2
Cardiology, University of Leipzig-Heart Center, Leipzig, Germany, Leeds General Infirmary, Leeds, United Kingdom, Philips Medical Systems, Best, Netherlands, University of Leipzig-Heart Center, Leipzig, Germany.
Introduction: First pass myocardial perfusion magnetic resonance (MR) has the advantage of a high spatial resolution, which allows differentiation between subendocardial and transmural perfusion defects. Furthermore it is free from attenuation artifacts. However, the absence of efficient, easy and reliable image analysis software is an obstacle for the introduction of this method into clinical practice.
Purpose: To assess a new MR first-pass perfusion software in comparison to SPECT and X-ray angiography.
Methods: Twenty-one patients underwent both 99m Technetium SPECT and first-pass magnetic resonance perfusion imaging under rest and stress using adenosine and additionally X-ray angiography. Off-line image analysis was performed in 6 steps on a dedicated workstation using prototype software (EasyScil, Philips Medical Systems, The Netherlands), which allows image analysis in less than 10 min. per slice and displays the results, which are based on the assessment of a myocardial perfusion reserve index derived from the upslopes of the time-signal-intensity curves, in color-encoded images. Visual interpretation of the color displays was performed by two independent observers and areas of relative underperfusion were reported. All SPECT studies were analyzed in the conventional manner using a subjective scale and results were compared to MR. The results of SPECT and MR perfusion were independently compared for the detection of >70% stenosis in X-ray angiography.
Results: Taking SPECT as a reference method resulted in a sensitivity of 80%, specificity of 91%, positive predictive value of 68%, negative predictive value of 95% and a total accuracy of 89%. In comparison to X-ray angiography overall accuracy was 87% for MR perfusion and 77% for SPECT to detect significant coronary artery disease with stenosis>70% (Fig. ).
Conclusions: Post-processing of first pass myocardial perfusion MR imaging using a new semiautomatic software, which easily generates the results semi-quantitatively and displays it visually as color-encoded images has a high sensitivity and specificity for detection of perfusion defects in comparison to SPECT and a higher accuracy in detecting significant coronary artery disease. This post-processing method may accelerate the time-consuming analysis of MR perfusion images thus enabling a more widespread clinical utility.
113. Combined T2-Weighted and Delayed Enhancement MRI Differentiates Acute from Chronic Myocardial Infarction
Hassan Abdel-Aty, Jeanette Schulz-Menger, Nidal Al-Saadi, Daniel Messroghli, Andreas Kumar, Andrew J. Taylor, Matthias G. Friedrich.
Cardiology, Franz-Volhard-Klinik, Charite, Campus Berlin-Buch, Humboldt University, Berlin, Germany.
Introduction: Delayed enhancement (DE) MRI accurately detects the localization and size of myocardial infarction (MI). Both acute and chronic (MI), however, show DE. STIR (Short TI inversion recovery) T2-weighted MRI identifies acute MI by virtue of infarct-associated myocardial edema, a pathological feature of acute but not of chronic MI.
Purpose: We investigated the clinical utility of a combined approach using DE and STIR to differentiate acute from chronic infarcts.
Methods: We studied 101 patients, 74 with acute MI (61 males, 56y±16, infarct duration 4±3days), 27 patients with chronic MI (21 males, 57y±13, infarct duration 18 months±13) and 18 healthy subjects (8 males, 35y±4) on a 1.5 T scanner using a breathhold STIR sequence (TR 2R-R intervals, TE 64 ms, TI 140 ms, number of slices 3) and DE imaging of the same short axis slices applying an inversion recovery gradient echo pulse sequence (TR 5.5 ms, TE 1.4 ms, TI 200-250 ms). The region of the infarction was defined by angiography combined with the presence of wall motion abnormality in the territory of the culprit coronary artery. MR images were evaluated by two independent observers for a match or mismatch pattern. A match was defined as the combination of positive DE and transmural high STIR signal intensity. Mismatch was defined as the presence of only one feature and the absence of the other in the infarct bed. Diagnostic accuracy for MR was evaluated for the detection of myocardial infarction (myocardial infarction present or not) and for the correct assignment to the territory of the infarct bed.
Results: All infarcts, acute and chronic (101 patients), could be detected by the presence of DE (sensitivity for the detection of any infraction 100%). DE alone, however, did not differentiate between acute and chronic infarcts. In 71/74 patients with acute infarcts but in none of the chronic infarct patients there was a transmural high signal intensity in the STIR images. Thus, a “match” pattern with both, DE and a transmural high signal in the STIR, identified acute myocardial infarction with a sensitivity of 96% and a specificity of 100%. DE and high STIR signal (when present) matched the territory of the infarct bed in all patients (acute and chronic). None of the control group showed DE or a high STIR signal.
Conclusion: A combined DE and STIR approach is not only sensitive in detecting myocardial infarction, but also accurately differentiates acute from chronic infarcts.
114. Does Transmyocardial Laser Revascularization (TMR) Give Rise to Myocardial Fibrosis?
Penelope R. Sensky,1 Nilesh J. Samani, FRCP,1 Graham R. Cherryman, FRCR.2
Cardiology, Glenfield Hospital, Leicester, United Kingdom, Radiology, Leicester Royal Infirmary, Leicester, United Kingdom.
Introduction: The longterm physiological effects of laser channel creation in human myocardium is unclear. Fibrosis hindering myocardial perfusion remains a concern.
Purpose: To compare remote microvascular integrity within myocardial regions undergoing laser treatment with non-lased regions (controls).
Methods: Ten patients referred for TMR because of refractory angina unsuitable for conventional revascularization were recruited. TMR was performed with a pulsed holmium. YAG laser (Cardiogenesis Corporation, Sunnyvale, California, USA, radiation wavelength 2.1 μm, energy 2 Joules per pulse, pulse duration 350 μs, 365 μm flexible fibre delivery system). Transmyocardial channels (2–3 pulses, distribution 1 cm−2, diameter 1 mm) were drilled from the epicardium to the endocardium in anterior, lateral and inferior walls. Only areas visually devoid of fibrous scar material were lased. Delayed contrast MRI was performed prior to surgery and 6 months afterwards. A short axis inversion recovery snapshot-FLASH sequence (TR=4.5 ms, TI=300 ms, TE=2 ms, FOV=3002 slice thickness=9 mm, 96×128 matrix) was employed at the level of the mid-papillary muscles before and 20 minutes after injection of a gadodiamide bolus (0.025 mmol/kg).The myocardium was divided into 4 regions of interest (ROI) corresponding to lased areas (anterior, inferior and lateral walls) and non-lased septum (control region). Regional signal intensity was extracted. To evaluate delayed hyperenhancement (DHE), the percent increase in signal intensity after gadodiamide compared with baseline was calculated. Differences in DHE between pre- and postoperative imaging in lased versus non-lased ROI were compared.
Results: An increase in DHE (Table ) was seen post-operatively in ROI in which laser channels were created (p<0.05). No increase was seen in the control ROI.
Conclusions: This finding implies that myocardial fibrosis may be stimulated by laser application, reflecting possible laser-induced injury or myocardial channel healing with scar formation. Such changes have the potential to have deleterious consequences on myocardial perfusion and function in the longterm. TMR remains a controversial treatment in patients with refractory angina.
Table 1.
115. Chagas Disease Is Characterized by Specific Pattern and Location of Myocardial Delayed Enhanced MRI
Carlos E. Rochitte, MD,1 Paulo F. Oliveria, MD,2 Joalbo M. Andrade, MD,2 José R. Parga, MD,2 Luiz F. Ávila, MD,2 Claudio C. Campi, MD,2 Rosa M. Piva, PhD,2 Roberto Kalil, Filho, MD,2 Giovanni G. Cerri, MD,2 José A. F. Ramires, MD.2
Cardiology, Heart Institute -InCor- University of Sao Paulo Medical School, São Paulo, Brazil, Cardiology, Heart Institute-InCor-University of São Paulo Medica School, São Paulo, Brazil.
Introduction: Myocardial fibrosis (MF) has been shown in pathological studies of Chagas disease (CD) and typically is located on apical and basal inferolateral left ventricular segments. Myocardial delayed enhanced MRI (MDE) delineates MF in detail in patients with previous myocardial infarction (MI).
Purpose: We investigated whether MDE could detect MF in patients (pts) with CD.
Methods: Twenty-five pts with CD and no MI underwent MRI exam on a 1.5T GE CV/i system. We acquired 8–10 left ventricle (LV) short-axis (SA) slices, using gradient-echo in steady-state acquisition (FIESTA) for LV function and gradient-echo with inversion-recovery pulse for MDE. Parameters for FIESTA: TR 3.9ms, TE 1.7ms, FA 45, Phases 20, Matrix 256×160, ST 8 mm, FOV 32–38 cm; and for MDE. TR 7.1ms, TE 3.1ms, TI 150-250ms, FA 20, BW 31.25 kHz, Matrix 256×192, NEX 2, RR 1. Two observers scored 425 LV segments for MF transmurality and pattern (subendocardial, midwall and subepicardial) and measured LV ejection fraction (EF), mass and the extent of MF (Fig. ).
Results: We identified MF in 22 of 25 pts and in 31.3% of LV segments (133/425). From the segments with MF, 51.9% (69/133) were located on the infero-lateral and apical segments. Moreover, midwall and subepicardial MF, with viable subendocardium, were observed in 46.6% (62/133) of segments, opposed to the typical subendocardial MF of MI.
The extent of MF had a good negative correlation with LVEF (r=−0.74, p<0.001). Pts with EF 40% (n=12) had a greater extent of MF (24.1 2.2% vs. 11.5 2.4%, p<0.001) than those with EF 40% (n=13).
Conclusions. Myocardial delayed enhanced MRI can characterize myocardial fibrosis on Chagas disease patients. This can contribute to the pathophysiological knowledge and may carry diagnostic and prognostic information.
Figure 1. Arrows indicate regions of myocardial hyperenhancement on anteroseptal and inferolateral LV segments on 3 adjacents short axis slices of the same patient. Note that, on inferolateral hyperenhanced region, most of the subendocardium is not enhanced (viable). Note also a small and focal hyperenhancement on inferior wall, not indicated by arrows. This displays a typical CD MDE study. several myocardial delayed hyperenhanced regions involving segments not related to any specific coronary artery territory, focal areas, and preserved, viable subendocardium.
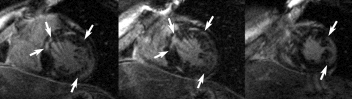
116. Myocardial Hyperenhancement in Hypertrophic Cardiomyopathy: Insights from a Genotyped Population
James C. C. Moon,1 Jens Morgensen,2 Gillian C. Smith,1 Andrew G. Elkington,1 Sanjay Prasad,1 Perry M. Elliott,2 Dudley J. Pennell,1 William J. McKenna.2
Royal Brompton Hospital, London, United Kingdom, St George's Hospital, London, United Kingdom.
Introduction: Patients with Hypertrophic Cardiomyopathy (HCM) may have areas of myocardial hyperenhancement after gadolinium-DTPA by CMR. The relationship of hyperenhancement to genotype and stage of disease is unknown.
Purpose: To study a population with a single sarcomeric protein mutation to better assess the relationship between hyperenhancement and disease stage.
Methods: Thirty patients from 13 families, all with mutations in Troponin I, underwent cine and contrast enhanced CMR. 15 patients had HCM with abnormal wall thickness on echo (LVH+) and 15 had normal echoes (LVH−) and therefore early or no manifest disease.
Results: All (100%) LVH+ patients and 4 (27%) LVH- patients had abnormal cine CMR with abnormal regional hypertrophy. Hyperenhancement was present in 12 (86%) LVH+ patients and 3 (20%) LVH-, although less extensive (15% LVH+, range 3-48% vs 3.6% LVH−). Overall, the extent of hyperenhancement was related to high clinical risk of sudden death (32 standard risk factors for sudden death), (mean 15% high risk vs 4%, p=0.03), total LV mass (r=0.56, P<0.001) and inversely to ejection fraction (r=0.58, P<0.001). Increased hyperenhancement was not associated with age over the whole group, but was within pedigrees (Fig. ). Segmental analysis demonstrated an increase in prevalence (p<0.0001 for trend)and extent of hyperenhancement (r=0.99) with increasing segmental wall thickness. There was no specific pattern of hyperenhancement within this genotype, but RV insertion point hyperenhancement was absent.
Conclusions: Hyperenhancement occurs in almost all LVH+ patients, but cine and contrast enhanced CMR detected disease expression in a significant additional number of echo classified LVH− patients. The intra-pedigree increase of hyperenhancement with age is evidence that hyperenhancement increases over time reflecting disease progression. Thus hyperenhancement may have a role both in the diagnosis of early disease and in established disease as a potential marker for clinical risk.
117. Delayed Enhanced Magnetic Resonance Imaging Detects Myocardium Injury in Acute Myocarditis
Marcelo Hadlich,1 João L. Petriz,2 Clerio F. Azevedo,2 Luis A. Mendonça,2 Jorge Moll,2 Carlos E. Rochitte.3
Cardiac MRI, Rede D'Or & Labs, Rio de janeiro, Brazil, Cardiac MRI, Rede D'Or & Labs, Rio de Janeiro, Brazil, Cardiac MRI, Heart Institute (InCor) University of São Paulo Medical School, São Paulo, Brazil.
Introduction: Contrast-enhanced MRI (CE) can delineate irreversible ischemic myocardial injury. Detection of myocardial injury in patients (pts) with myopericarditis and the differentiation from ischemic injury remains a challenge.
Purpose: To evaluate whether CE could detect irreversible myocardial injury in pts with myopericarditis and elevated cardiac serum markers.
Methods: Five pts with acute pericarditis and elevated cardiac markers were submitted to CE, using an inversion-recovery prepped gradient-echo after an IV injection of 0.2mmol/kg of gadolinium-DTPA (MDE) and a cine-MR using a steady state acquisition gradient-echo (B-FFE) in a 1.5T whole-body magnet (Intera NT, Philips). Parameters for B-FFE: TR 3.9 ms, TE 1.7 ms, FA 45, Phases 20, Matrix 256×160, ST 8 mm, FOV 32–38 cm; and for MDE: TR 7.1ms, TE 3.1ms, TI 150–250 ms, FA 20, BW 31.25kHz, Matrix 256×192, NEX 2, RR 1. The presence of myocardial delayed hyperenhancement, the transmural extent in 85 left ventricle (LV) segments and the global extent of hyperenhancement as percent of LV mass were measured.
Results: Forty percent of LV segments (34/85) showed hyperenhancement by CE. From those, 73% (25/34) involved midwall and subepicardium. No involvement of subendocardium noted. Hyperenhancement areas (arrows) were small and diffusely distributed, not restricted to any specific coronary artery territory (Fig. ). There was a good correlation (r=0.89) between total myocardial injury (15.2±2.1%) and LV ejection fraction (62.1±4.2%). The same areas of hyperenhancement were detected on the 3-month follow-up (1 pt), suggesting irreversible myocardial injury (Figure 1).
Conclusions: Delayed-enhanced MRI can detect myocardial irreversible injury in patients with myopericarditis. Delayed hyperenhancement patterns differ in myopericarditis from the myocardial infarction pattern. This technique can be a helpful tool on the differential diagnosis of myopericarditis and myocardial infarction.
118. Cardiac Magnetic Resonance Imaging Findings in Sarcoidosis
Olivier Vignaux, MD, PhD,1 Robin Dhote, MD,2 Denis Duboc, MD, PhD,3 Christophe Argaud, Msc,4 Paul Legmann, MD, PhD.5
Radiology, Hopital Cochin, Paris, France, Internal Medicine, Hopital Cochin, Paris, France, Cardiology, Hopital Cochin, Paris, France, GEMS, Buc, France, Radiology, Hopital Cochin, Paris, France.
Introduction: Cardiac involvement is symptomatic in only 5% of patients with sarcoidosis, although it is present in the myocardium at autopsy in 20 to 50% of patients, while sudden death accounts for 30–65% of deaths due to sarcoidosis. Non-invasive imaging methods such as echocardiography and thallium scan suffer from either low sensitivity or specificity and endomyocardial biopsy may be negative because of the patchy distribution of lesions.
Purpose: To prospectively evaluate the cardiac magnetic resonance (MR) imaging findings in various staged sarcoidosis.
Methods: Breath-hold T2-weighted black-blood single-shot fast spin-echo, functional segmented gradient echo and T1-weighted Gadolinium-DTPA-enhanced multislice MR examinations of the heart acquired at 1.5 T with ECG-triggering were performed in 62 consecutive patients with ongoing sarcoidosis. Cardiac evaluation included also physical examination, 24H-monitoring-ECG, echocardiography and thallium-201 myocardial scintigraphy.
Results: MR images were normal in cardiac-asymptomatic stage I or Lofgren syndrome patients (n=12). 32/45 patients with multiple organ cardiac-asymptomatic sarcoidosis displayed similar MR abnormalities to those observed in patients with cardiac symptoms (n=5). Focal myocardial thickness and/or an intramyocardial increased signal on T2-weighted and/or an intramyocardial increased signal on gadolinium-DTPA-enhanced T1-weighted images, were graded into three MR patterns based on histological data. Actually available MR one-year follow-up in 18 patients showed regression of gadolinium-DTPA uptake after corticoid therapy while progression was recorded in 5 patients without treatment.
Conclusions: MR imaging enables valuable detection and localisation of cardiac sarcoidosis. The occurrence of subclinical lesions in multiple organ sarcoidosis may legitimate the use of MR as a screening method to early identify patients requiring careful review and treatment.
119. Catheter-Based Projection Coronary MR Angiography—A Feasibility Study
Jordin D. Green, MS, Reed A. Omary, MD, MS, Richard Tang, MD, Yongzhong Li, MD, Brian E. Schirf, MD, J. Paul Finn, MD, Debiao Li, PhD.
Northwestern University, Chicago, IL, USA.
Introduction: One of the potential applications for MRI-guided interventions is angioplasty, which requires accurate delineation of coronary arteries before and after the procedure. After the catheter is placed in the coronary artery via MRI guidance, contrast agent can be injected directly into the coronary arteries. The feasibility of performing real-time visualization of coronary arteries after intra-arterial (IA) contrast injection has been recently demonstrated (1). Thus far, there has been no demonstration of diagnostic-quality submillimeter spatial resolution imaging of the coronary system with IA-contrast injection.
Purpose: The purpose of this study was to optimize the imaging protocol for obtaining diagnostic-quality submillimeter spatial resolution coronary MR angiography following IA contrast agent injection.
Methods: All MR scanning was performed on a 1.5 T Siemens Sonata system (Siemens Medical Solutions, Erlangen, Germany). 6-French catheters were introduced into the femoral artery of swine (n=4) and advanced into the coronary ostium under MR-guidance using a 0.30 inch diameter nitinol loopless guidewire antenna for signal detection (Intercept, Surgi-Vision, Inc, Gaithersburg, MD). Coronary angiography was performed by injecting 3–6 mL of diluted Gadolinium-chelate contrast agent (8% by volume; Magnevist, Berlex, Wayne, NJ) through the catheter over 2–4 s. To evaluate different acquisition schemes, each MRA was performed twice, once with a TrueFISP (Fast Imaging with Steady-State Precession) acquisition scheme and once with a Fast Low Angle Shot (FLASH) acquisition scheme. Both sequences were ECG-triggered to reduce blurring due to cardiac motion. To reduce background signal and increase vessel depiction, a magnetization-preparation scheme was selected which consists of a series of spoiled non-selective low flip angle pulses being played out during the trigger delay period. These are followed by a non-selective 180° inversion pulse, followed by an inversion time (TI), followed by centric-encoded section-selective data acquisition. For TrueFISP imaging, an alpha/2 preparation pulse was included at the end of the TI.
The sequence parameters for TrueFISP were as follows: TR/TE/flip angle=3.5 ms/1.5 ms/75°; FOV=200×400 mm2; matrix=256×512; slice thickness=50 mm; TI=50 ms. The sequence parameters for FLASH were identical except that a flip angle of 20° was used.
In coronary projection imaging, the coronary arteries can become obscured after contrast agent enters the myocardium. Therefore choosing the proper injection duration is very important. To examine the impact of injection duration on coronary artery depiction, in one animal, a long (20 s) injection of contrast agent was given and the coronary artery delineation was monitored 7 times a second over the entire injection.
Results: In all cases, the catheter was successfully placed in the left main coronary artery. Following injection of diluted contrast agent, the left main, left anterior descending, and/or the left circumflex coronary artery became enhanced (Fig. ). Because the magnetization-preparation scheme provides T1-weighted background suppression, the signal from background tissue (i.e. myocardium, fat) was low in all images. Mean CNR (Contrast-to-Noise Ratio) using the TrueFISP acquisition scheme was found to be 7.12, while mean CNR using the FLASH acquisition scheme was found to be 3.62. The increase in CNR for the trueFISP images was found to be statistically significant (p<0.05) (Fig. ). No significant artifacts were detected in any of the TrueFISP images.
When contrast was injected over a long duration, it was found that myocardium becomes enhanced and obscures the coronary artery after as little as 3–4 s. Therefore image acquisition time should be less than 4 s while acquiring enough lines to attain submillimeter resolution in the allotted image acquisition time. On the other hand, the number of lines/segment should be minimized in order to reduce the effect of cardiac motion. This suggests that 2D thick-slice projection imaging may be more appropriate for interventional diagnostic coronary imaging than 3D imaging, because for the same in-plane resolution, 3D imaging typically requires a larger image acquisition time compared to 2D imaging.
Conclusion: This study demonstrates that an optimized intraarterial coronary MRA sequence should use a TrueFISP acquisition scheme with a short acquisition time in order to minimize the injection duration. This technique may be useful in diagnosing coronary artery disease in an interventional setting using MRI.
Figure 1. a) Precontrast 3D TrueFISP localizer of the left anterior descending (LAD) coronary artery (black arrows) of a swine. b) Coronary system of the same animal following IA injection of diluted contrast agent through a percutaneously placed catheter. Both the LAD and the circumflex arteries are visible, as well as several marginal arteries.
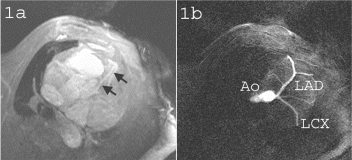
Figure 2. The LAD of a swine following dilute contrast agent injection acquired using a) FLASH and b) TrueFISP. Distal portions of the artery and the branch near the root (arrows) are better depicted in b) due to the better CNR obtained using the TrueFISP acquisition scheme.
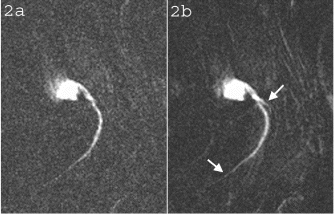
References
1. Serfaty J-M et. al. Radiology 2000; 217. 290–295.
120. MR Imaging at 3.0 Tesla and Coronary Artery Stents. Ex Vivo Evaluation of Magnetic Attraction Forces and Heating
Alexandra Schmiedel,1 David Maintz, MD,2 Matthias Hackenbroch, MD,1 Bettina Schmiedel, MD,1 Hans Schild, MD,1 Torsten Sommer, MD.1
Department of Radiology, University of Bonn, Bonn, Germany, Department of Radiology, University of Muenster, Muenster, Germany.
Introduction: Radio-frequency related heating and dislocation of metallic implants due to magnetic forces are main safty concerns in high-field MR-imaging.
Purpose: To evaluate the MR compatibility of various coronary artery stents with respect to magnetic attraction and heating at 3.0 Tesla.
Methods: 20 commonly used coronary artery stents were studied in a 3.0 T system (Intera, Philips Medical Systems). NIR Primo-NIR Royal-Radius (Boston Scientific Europe), Palmaz -Crown- Crossflex-Palmaz-BxVelocity (Cordis), ACS RX Multilink-ML Tetra-Herculink-ML Tristar-ACS Multilink Duett (Guidant), beStent-Wiktor (Medtronic) Sirius Carbostent (Sorin Biomedica), Jostent Flex-Jostent StentGraft (Jomed), Flex AS (Phytis), BiodivYsio (Biocompatibles). Each stent was evaluated for:
1. Magnetic deflection forces: The stents were suspended by a fine string and placed in the magnet bore at the location of the greatest magnetic field gradient. The deflection angle was measured 3 times and deflection forces were calculated.
2. Magnetic field-induced torque: Each stent was placed on a plastic Petri dish, with a millimeter ruler beneath, in a position perpendicular to the static magnetic field (position 90°) and slowly moved on the MR table into the center of the magnetic bore. Any possible displacement of the stents relative to the millimeter ruler was noted. The stent was turned in steps of 45°, and the procedure was repeated to encompass a full 360° rotation of positions.
3. Heating: The stents were implanted in a cadaveric porcine heart and placed in a rectangular plastic phantom filled with 0.9% saline solution. A fiber-optic temperature probe (resolution. 0.05°C, response time. 250 msec) was attached directly to the stents to measure temperature continuously during MR imaging. To simulate a worst case heating condition a fast spin echo sequence producing an estimated whole-body averaged absorption rate of 3.9 W/kg was applied for 15 min.
Results: Magnetic deflection force: In all devices tested the deflection force (range 0.01–0.18 mN, mean 0.07 mN ±0.02 [SD]) was less than the gravitational force (i.e. the stents weight).
Torque: None of the stents aligned to or rotated in the magnetic field at any of the various 45°-interval positions.
Heating: Maximum temperature increases of the stents ranged from 0.1°C to 0.8°C (mean 0.2°C ±0.19).
Conclusions: MR imaging at 3.0 Tesla may be performed safely in pts with any of the 20 different coronary artery stents evaluated in this study.
121. Artifact-Free Coronary MR Angiography and Coronary Vessel Wall Imaging In Vivo Using a New Metallic Coronary MRI Stent
Elmar Spuentrup,1 Alexander Ruebben,1 Andreas Mahnken,1 Matthias Stuber,2 Christian Koelker,1 Sylvia Kinzel,1 Trung Hieu Nguyen,1 Rolf W. Günther,1 Arno Buecker.1
Aachen Technical University, Aachen, Germany, Beth Israel Deaconess Medical Center and Harvard Medical School, Boston, MA, USA.
Introduction: In-stent lumen of currently used metallic coronary stents can not be visualized by coronary MR angiography (MRA) due to susceptibility artifacts and RF-shielding. Recently, a new dedicated MRI stent was introduced for artifact-free contrast-enhanced renal MRA (1). In-stent lumen visualization of coronary stents is more challenging due to the small size of the coronary arteries and the lack of exogenous contrast media application in currently used coronary MRA approaches.
Purpose: The aim of this study was the investigation of a coronary MRI stent for artifact-free coronary MRA and coronary vessel wall imaging using variable coronary MRA and coronary vessel wall imaging approaches.
Methods: Fifteen prototype coronary MRI stents (Aachen Resonance, Aachen, Germany) were deployed in coronary arteries in 15 healthy swine (RCA:n=3, LCX:n=2, LAD:n=10) and investigated on a 1.5T ACS-NT MR-scanner (Philips, Best, NL) with a standard double-oblique navigator-gated free-breathing T2-prepared 3D gradient-echo sequence (2) (TR=7.2ms, TE=2.0 ms, FA=30°, 0.1×0.7mm in-plane resolution), a T2-prepared 3D spiral coronary MRA sequence (3) (TR=30ms, TE=2.8, 0.7×0.7mm in-plane resolution) and a T2-prepared 3D steady-state-free-precession coronary MRA sequence (4,5) (TR=3.8ms, TE=1.9ms, FA=75°, 1.2×1.2mm in-plane resolution). Furthermore, black-blood coronary vessel wall imaging using a dual-inversion-recovery turbo spin-echo sequence was performed (TR=2 heart beats, TE=25ms, 0.6×0.6mm in-plane resolution, reconstructed to 0.312×0.312mm)(6). Artifacts of the stented vessel segment (no artifacts or minor/major artifacts) were visually assessed by two investigators. Furthermore, signal intensities of the coronary vessel lumen inside the stent and outside the stent were compared. Regions-of-interests for signal-to-noise (SNR) measurements were determined with respect to stent localization as derived from cardiac multislice CT.
Results: With all investigated sequences, coronary vessel lumen and coronary vessel wall could be visualized without any artifacts including the stented vessel segment. Quantitative SNR calculations yielded no significantly different SNR inside the stent when compared to the SNR outside the stent. Coronary vessel wall could be artifact-free visualized in the presence of the new coronary MRI stent (Fig. ).
Conclusions: The new coronary MRI stent allows for completely artifact-free coronary MR angiography and coronary vessel wall imaging.
Figure 1. Multiplanar reformats of the used coronary MRA sequences in the presence of the new metallic coronary MRI stent. The bars indicate stent position as derived from multislice CT. A=T2-prepared 3D gradient-echo sequence, stent in LAD, B=T2-prepared 3D spiral sequence, stent in LCX, C=T2-prepared 3D SSFP sequence, stent in RCA, D=coroanry vessel wall imaging perpendicular to the main axis of LAD at the level of the stent in A (white dots).
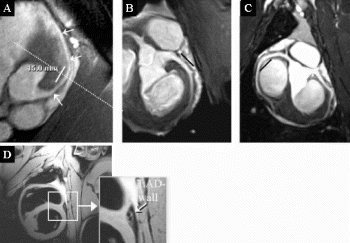
References
1. Buecker A, Spuentrup E, Ruebben A, Gunther RW. Artifact-free in-stent lumen visualization by standard magnetic resonance angiography using a new metallic magnetic resonance imaging stent. Circulation 2002; 105:1772–1775
2. Kim WY, Danias PG, Stuber M, Flamm SD, Plein S, Nagel E, Langerak SE, Weber OM, Pedersen EM, Schmidt M, Botnar RM, Manning WJ. Coronary magnetic resonance angiography for the detection of coronary stenoses. N Engl J Med 2001; 345:1863–1869
3. Bornert P, Stuber M, Botnar RM, Kissinger KV, Koken P, Spuentrup E, Manning WJ. Direct comparison of 3D spiral vs. Cartesian gradient-echo coronary magnetic resonance angiography. Magn Reson Med 2001; 46:789–794
4. Stuber M, Boernert P, Botnar RM, K.V. K, Spuentrup E, Manning WJ. Three-Dimensional TrueFISP Coronary Magnetic Resonance Angiography. Proc. Soc. Cardiovasc. Magn. Reson. 2001:157
5. Spuentrup E, Ruebben A, Schaeffter T, Manning WJ, Gunther RW, Buecker A. Magnetic resonace-guided coronary artery stent placement in a swine model. Circulation 2002; 105:874–879
6. Botnar RM, Stuber M, Kissinger KV, Kim WY, Spuentrup E, Manning WJ. Noninvasive coronary vessel wall and plaque imaging with magnetic resonance imaging. Circulation 2000; 102:2582–2587.
122. Initial Experience with Spiral MR Coronary Angiography at 3T: Anatomic Coverage, Image Quality, and Susceptibility Artifacts
Phillip Yang,1 Patricia Nguyen, MD,1 Ann Shimakawa, PhD,2 Jean Brittain, PhD,2 Bob Hu, MD,3 Michael McConnell, MD.1
Cardiovascular Medicine, Stanford University, Stanford, CA, USA, General Electric Medical Systems, Menlo Park, CA, USA, Cardiovascular Medicine, Palo Alto Medical Foundation, Palo Alto, CA, USA.
Introduction: Spiral MR coronary angiography (MRCA) has several advantages but is prone to susceptibility artifacts. MRCA at higher magnetic field strengths can enhance signal-to-noise ratio (SNR) enabling higher spatial resolution. However, limitations of higher field strength including RF inhomogeneity and susceptibility artifact have not been addressed.
Purpose: A study was conducted to assess anatomic coverage and image quality with spiral MRCA implemented at 3T.
Methods: Real-time and high-resolution spiral imaging sequences installed on GE Signa 3T whole body system (GE, Milwaukee, WI) equipped with a gradient system capable of 40 mT/m peak amplitude and 150 mT/m/msec slew rate were implemented with a 5-inch surface coil. The study consisted of 5 subjects (3 volunteers and 2 patients). The imaging protocol consisted of rapid real-time localization of the coronary arteries followed by high-resolution imaging. The real-time sequence consisted of gradient recalled echo sequence (31-ms TR, 5.2-ms TE, and 30° flip-angle) utilizing 10-ms spectral spatial pulse and 16-ms spiral read-out (4 interleaves and 4096 points). A frame rate of 5 complete frames/s generated a spatial resolution of 2.25mm with a FOV of 24cm. The high-resolution multi-slice sequence consisted of gradient recalled echo sequence (1 heartbeat TR, 5.2-ms TE, and 60° flip-angle) utilizing 10-ms spectral spatial pulse and 16-ms spiral read-out (20 interleaves and 4096 points). Five slices with 5 mm slice thickness and a FOV of 24 cm generated a spatial resolution of 0.69×0.69 mm in 20 heart-beat breath-hold. Analysis of coronary coverage was based on the number of coronary segments (9) seen in the coronary anatomy. Image quality was graded based on contiguity of the vessel border and artifact present in each segment. The scale ranged from 1–4 (1 excellent, 2 good, 3 fair, and 4 non-diagnostic).
Results: Coronary segments were visualized in 80% (36/45) of all segments. Excellent or good image quality was achieved in 76% of all segments. These segments were LM, proximal-LAD, mid-LAD, proximal-RCA, mid-RCA and proximal-LCx. Sample images of RCA, LCx, and mildly diseased LAD are shown in Fig. . Significant susceptibility effects were noted along the lateral and apical regions of the left ventricle as shown by a block arrow on Fig. .
Conclusion: High field imaging at 3T holds promise to enhance spiral MRCA. However, the image quality in the distal LAD and distal LCx may be limited by increased susceptibility at higher field strength.
123. Navigator-gated Coronary Mra With Subject-specific Acquisition Windows And Motion Adaptive Gating-comparison With A Conventional Acquisition Sequence
Sven Plein, Jones Tim, John P. Ridgway, Mohan U. Sivananthan.
Cardiac MRI Unit, Leeds General Infirmary, Leeds, United Kingdom.
Introduction: A practical limitation of free-breathing navigator-gated coronary magnetic resonance angiography (MRA) are the long scan times of this technique. These are caused mainly by (1) the narrow acquisition windows that have to be used to avoid image blurring from coronary motion and by (2) low navigator efficiencies.
(1) Conventionally, acquisition windows in coronary MRA are fixed and trigger delays set according to the heart rate. This approach does not consider the substantial variability of coronary motion between individuals. Subject-specific acquisition windows may allow the use of longer acquisition windows in particular in subjects with lower heart rates without impairing image quality.
(2) The low efficiency of navigator-gated MRA is caused mainly by irregular breathing patterns or drifting of the end-expiratory diaphragm position especially in long acquisitions. Motion adaptive gating, navigator drift corection and shorter scan times may improve navigator efficiency.
Purpose: To compare a coronary MRA technique which uses subject-specific acquisition windows and motion adaptive gating with conventional navigator gated acquisition with fixed acquisition and navigator windows.
Methods: Ten subjects (5 volunteers and 5 patients with CAD) were studied on a 1.5T MR system (Philips, Best, The Netherlands). From a coronary scout scan the course of the right and the left coronary systems was identified. High-resolution MRA scans of both coronary systems were then acquired using a conventional and the “adaptive” technique in identical locations and in random order. The conventional MRA sequence was a 3D segmented k-space gradient echo sequence (TE=2.7 ms, TR=9.4 ms, flip angle 20°, T2 preparation prepulses, spatial resolution 1.04×0.78 mm, 1.5 mm, 16 slices, acquisition window constant at approximately 73 ms, trigger delay set according to the heart rate) with a right-hemidiaphragmatic navigator (navigator acceptance window fixed at 5 mm). The “adaptive” acquisition sequence was identical except for a subject-specific acquisition window and an adaptive gating window. The acquisition window was determined individually from high temporal resolution cine images acquired in a plane perpendicular to the course of the proximal coronary arteries (Steady State Free Precession=SSFP, TR 2.8 ms, TE 1.4 ms, flip angle 55°, 30 phases). The acquisition window was set in the period of minimal coronary motion for each vessel. Adaptive gating used a 2 mm window for the central 35% ofk-space and 6 mm for the outer 65% of k-space. For this scan, we also allowed continuous correction of the mean gating window position.
The acqusition window, trigger delay, navigator efficiency and scan time were recorded for each coronary acquisition. The image quality of each data set was rated subjectively on a scale of 1–4 by an observer blinded to the acquistion method used. The total vessel length was measured and SNR in the aorta, proximal coronary vessel and outside the chest wall as well as the CNR between myocardium and blood pool determined. All measurements were compared using a paired t-test.
Results: The mean heart rate of the 10 subjects was 62.7 (±17) bpm and was below 60bpm in 6 subjects. The mean period of minimal coronary motion observed on the cine images was 145 ms with a linear relationship between longer rest period and lower HR.
Table lists all results in comparison for the left and right coronary systems. The scan times were significantly shorter with the adaptive technique and the acquisition window longer. Navigator efficiencies were slightly but not significantly higher with. There were no statistically significant differences for image quality score, vessel length and SNR. CNR was higher in the conventional acquisition.
Conclusions: By using subject-specific acquisition windows and MAG, scan time was reduced by a factor of almost 2.5 in patients and volunteers compared with a conventional navigator gated MRA sequence. The shorter scan times were mainly the result of longer acquisition windows. We found that if the heart rate of subjects was below 60 bpm, a coronary rest period of up at least 130ms was observed, allowing the use of significantly longer aquistion windows than with the conventional technique. Despite longer acquisition windows, no significant differences in image quality parameters were observed, except for a lower CNR. Using adaptive gating and continuous gating level correction failed to improve navigator efficiencies significantly.
Table 1.
124. Contrast-Enhanced MR Imaging of Coronary Arteries with Gadomer-17
Jörg Barkhausen,1 Christoph Herborn,1 Oliver Bruder,2 Walter Schueler,2 Eike Nagel,3 Kokahn Shamsi.4
Department of Diagnostic Radiology, University Hospital, Essen, Germany, Department of Cardiology, Elisabeth Hospital, Essen, Germany, German Heart Center, Berlin, Germany, Schering AG, Berlin, Germany.
Introduction: Up to date contrast-enhanced magnetic resonance angiography (MRA) in clinical routine mainly has been performed with T1-shortening compounds freely diffusible to the intestitium. Several new intravascular MR contrast agents have recently been designed to improve blood signal-to-noise ratio (SNR) as well as contrast-to-noise ratio (CNR) of blood vessels and surrounding tissues. These agents have demonstrated auspicious results in animal studies and preliminary clinical trials. With regard to their strong T1 relaxivity and long intravascular retention these agents appear well suited especially for magnetic resonance coronary angiography.
Purpose: The purpose of this study was to evaluate the efficacy and safety of Gadomer-17, a recently introduced blood pool compound, in Magnetic Resonance Coronary Angiography (MRCA).
Methods: The study was conducted in accordance with guidelines set forth by the national and local ethics committee. Gadomer-17 (SHL 643A, Schering, Berlin, Germany), is a gadolinium-based blood pool agent (0.5 mmol/ml) with high relaxivity and rapid renal excretion. 12 patients (7 male, 5 female; age range, 46–78; mean, 61.3 years) with angiographically proven coronary artery disease underwent breath-hold MRCA on a 1.5 T scanner (Magnetom Sonata, Siemens, Erlangen Germany). Unenhanced MRCA was performed with double oblique imaging planes oriented to the major axis of the coronary arteries using a 3D steady-state free precession sequence (TR 3.8 ms, TE 1.6 ms, FA 65°, Matrix 512). Following intravenous bolus administration of a body-weight adapted dose of Gadomer-17 (0.1 mmol/kg) images were obtained using a 3D inversion recovery turboFLASH sequence (TR 5.8 ms, TE 2.2 ms, FA 25°, Matrix 512). SNR and CNR were calculated from ROIs within the vessel lumen and the myocardium. Image quality was assessed on a 4-point scale (1 non-diagnostic, 2 moderate, 3 good, 4 excellent). Statistical analysis was based on a Student t test where statistical significance was established at p<0.05. Catheter angiography and MRCA of the main coronary artery, proximal and middle LAD, proximal CX as well as proximal and middle RCA were compared segment-per-segment by two experienced readers in consensus (Fig. ).
Results: Intravenous administration of gadomer-17 was well tolerated by all patients, neither acute nor late-phase adverse effects occurred. Compared to pre-contrast images the CNR of blood and myocardium improved by 200% (p<0.05). CNR slowly decreased and remained elevated as long as 30 minutes following administration. For the 72 segments examined the overall image quality significantly improved from 2.0±0.9 to 2.9±0.9 on the contrast enhanced scan, and the number of non-diagnostic segments significantly decreased from 38% to 10%. Compared with conventional catheter angiography contrast-enhanced MRCA had a sensitivity of 80% and a specificity of 93% for the detection of substantial stenoses (luminal narrowing >50%).
Conclusions: Gadomer-17 significantly improves magnetic resonance coronary angiography and mandates further clinical evaluation in a larger patient cohort with coronary artery disease.
125. Free-Breathing Non-gated Coronary MRA Using a Patient-Calibrated Complex 3D Motion Model
Dirk Manke, PhD, Kay Nehrke, PhD, Peter Börnert, PhD.
Philips Research Laboratories, Hamburg, Germany.
Introduction: Prospective respiratory motion correction in free-breathing CMRA is expected to have the potential to considerably suppress respiratory motion artifacts, if large gating windows are used to increase scan efficiency. Today, simple 1D slice tracking is typically applied in CMRA1. However, respiratory motion patterns are patient-specific and differ between breath-holding and free-breathing2,3. Furthermore, a three-dimensional correction model was proposed earlier4 to overcome limitations arising from the 1D approach.
To cope with these problems in this work a free-breathing calibration procedure has been derived and tested, which automatically determines the correspondence between the multiple respiratory navigators employed and the motion model parameters (3D-translation plus expansion in head-feet direction). This model is used for prospective respiratory motion correction in the subsequently performed high-resolution CMRA scan.
Purpose: To improve image quality and reduce acquisition time in free-breathing coronary MRA.
Methods: Model Calibration: Time series of low-resolution 3D-images were acquired with an ECG-triggered single-shot TFE-EPI sequence. Each 3D EPI-shot (FOV 256×256×64mm3 (8 mm)3-voxel) has a duration of 120 ms and freezes motion almost completely. The series thus reflects the respiration-induced motion of the heart. Signals from three respiratory navigators were recorded quasi-simultaneously. A model-based image registration delivered a set of model parameters (3D-translation and head-feet expansion) for each 3D-image. The data were analyzed using a principal component analysis (PCA) to reduce the model complexity to a few major modes (1–3) and to find the correspondence between the major modes and an equivalent number of navigators. The analysis yields a coefficient matrix, used during the subsequent CMRA scan to calculate estimates of the model parameters from the navigator signals to feed prospective motion correction (cf. Fig. ).
Coronary Imaging: Experiments were performed on a Philips GYROSCAN ACS-NT (1.5T, PowerTrak 6000 gradients). A standard 3D gradient echo sequence5 was used (TR=8.0ms, TE=3.2ms, flip=30°) with fat-saturation and T2-preparation applied before the mid-diastolic acquisition window (70ms). To perform prospective correction of 3D-translation and head-feet expansion the transmitter and receiver frequencies and the gradient waveforms were appropriately adapted based on the previously determined coefficient matrix and the measured navigator displacements6. Since no gating was involved, the typical scan-time was ∼6 min. The RCA was imaged in 7 healthy volunteers.
Results: The 3D-translation+expansion correction provided significant improvements in image quality in comparison to the 3D-translation correction and the 1D correction model with fixed correction factor in those subjects with considerable deformations of the heart in the respiratory cycle (Fig. ). Only minor improvements compared to the 3D translation were observed for subjects where the translational motion dominated. In these subjects the calibrated 3D translation model provided already significant improvements compared to the 1D correction model with fixed correction factor7(Fig. ).
Nonlinear properties of motion patterns (hysteresis)3 were better described by the motion model, when more than one navigator was applied, and when the non-linear components were well reflected by the additional navigators (e.g. chest-wall).
Conclusion: The presented free-breathing calibration approach considerably improves prospective motion correction, resulting in enhanced motion artifact suppression and in significantly increased gating efficiency. Furthermore, superiority of the patient-specific calibrated 3D-translation+expansion model over the 3D-translation model has been shown. Further improvements may be expected for a full 3D-affine correction, which corrects for additional (e.g. rotational) motion modes present in the respiratory cycle. Support by the European Commission (IST-2000-28168 MRI-MARCB)
Figure 1. Patient-specific motion model calibration and its subsequent application for prospective motion correction.
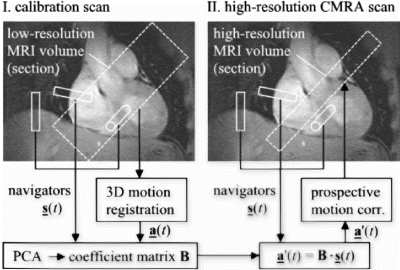
Figure 2. RCA of two volunteers (a–c and d–f) measured applying the three different motion models (a, d. 1D translation, b,e. 3D translation, c,f. 3D translation+linear expansion head-feet direction). No gating was involved.
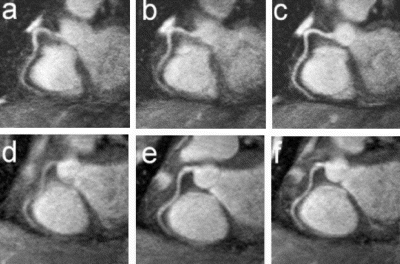
References
[1] Wang et al., MRM 33:713, 1995.
[2] Taylor et al., JMRI 9:786, 1999.
[3] Nehrke et al., Radiology 220:810, 2001.
[4] Manke et al., JMRI 15:661, 2002.
[5] Stuber et al., J.Am.Coll.Cardiol., 1999, 34:524
[6] Manke et al., ISMRM 2000, p.400.
[7] Manke et al., ESMRMB 2002, p.20.
126. Whole Heart Coronary MR Angiography Using a Magnetization Prepared SSFP Sequence
Oliver M. Weber, PhD, Alastair J. Martin, PhD, Charles B. Higgins, MD.
Department of Radiology, University of California, San Francisco, San Francisco, CA, USA.
Introduction: Free-breathing coronary magnetic resonance angiography (MRA) is a reliable and reproducible method to image the coronary arteries. Most proposed high-resolution sequences however target only a minor part of the coronary tree in thin slabs, requiring coverage in multiple volumes and focusing mainly on the proximal segments of the left main (LM), the left anterior descending (LAD), and the right coronary artery (RCA), but neglecting the left circumflex (LCX) coronary artery and more distal segments of all coronary arteries. Recently proposed refocused steady state free precession (SSFP) sequences, in combination with appropriate magnetization preparation, provide visualization of vessels over a longer distance with high vessel sharpness and excellent background suppression. We hypothesized therefore that such a sequence might be able to image the coronary arteries over their full length in a single scan covering the entire heart.
Purpose: To demonstrate feasibility of full-coverage coronary magnetic resonance angiography (MRA) with high spatial resolution and good vessel visualization, and to compare to a targeted thin volume approach.
Methods: Five normal volunteers were examined on a 1.5 T Intera I/T (Philips Medical Systems, Best, the Netherlands) using a five-element synergy cardiac receiver coil. An imaging volume, consisting of 60–80 slices with 1.5 mm thickness, reconstructed to twice as many 0.75 mm-slices, was defined to cover the entire heart. An axial slab with 256–272 cm field of view was covered with an in-plane resolution of 1×1 mm2 and reconstructed to a 5122 matrix. Imaging sequence was a T2-prepared (TE=50 ms), fat-suppressed, refocused SSFP sequence (balanced turbo field echo; TR=5.1ms, TE=2.5 ms, flip angle=90°, 25 excitations per cardiac cycle, SENSE factor=2). Respiratory motion was compensated for using a prospective real-time navigator placed on the right semi-diaphragm. Images were reformatted separately for the RCA, LAD, and LCX. For comparison purposes, RCA and LAD were additionally covered with a conventional, thin-slab approach (10 slices of 3 mm thickness each, reconstructed to 20 slices with 1.5 mm, TR=5.2 ms, TE=2.6 ms, 16 excitations per heart cycle, no SENSE, otherwise identical imaging parameters). Visible vessel length, vessel sharpness, and vessel diameter were determined in a post-processing and quantification tool (SoapBubble tool; Philips).
Results: The entire-heart approach resulted in visualization of the coronaries over a longer distance (LAD, +77%, p<0.01; LCX, +67%, p<0.05; RCA, +12%, n/s) due to better coverage (Fig. ). These findings reflect the fact that RCA frequently runs more or less within a single plane and may thus be covered in a thin slab, whereas LAD and especially LCX have a more complicated geometry requiring larger-volume coverage.
The full-coverage approach slightly improved vessel sharpness (on average, +14%, n/s), probably due to higher through-plane resolution. Vessel diameter was virtually unchanged (on average, −4%, n/s). Signal to noise ratio was reduced by approximately 10%, due to higher spatial resolution and a SENSE factor of two, which were only partially compensated for by the larger volume covered. Acquisition time for the large volume was similar to the combined examination time for two thin slabs (approximately 10 minutes).
Conclusion: The proposed method allows MRA of all the major coronary arteries over a long distance at high image quality in a single scan. Improved visualization tools are necessary for appropriate visualization of the full coronary tree.
127. Fast Black-Blood Imaging of Carotid Arteries Using Multi-Slice Double Inversion-Recovery
Vasily L. Yarnykh, Ph.D., Chun Yuan, Ph.D.
Radiology, University of Washington, Seattle, WA, USA.
Introduction: Efficient suppression of the signal from flowing blood is of critical importance for cardiovascular MRI. Double inversion recovery (1,2) is a basic black-blood imaging technique, which provides excellent flow suppression, but suffers from long scan time caused by sequential slice acquisition. Two methods (3,4) were proposed recently to improve time efficiency of DIR by using multi-slice acquisition. In the method (3), only one slice can be imaged at the exact zero-crossing point of blood. The common problem of both techniques is the construction of the preparative module, which includes a train of slice-selective inversions. This circumstance creates unequal conditions for the evolution of magnetization of different slices, since delay between non-selective inversion and slice-selective re-inversion depends on the actual slice number. Due to inherent limitations, both methods (3,4) were restricted by two-times reduction of scan time as compared to standard DIR.
Purpose: To develop a time-efficient multi-slice DIR technique providing acquisition of flexible number of slices per TR and to evaluate its application for imaging of carotid arteries (CA).
Methods: A multi-slice DIR pulse sequence is based on the idea of simultaneous re-inversion of a group of slices. In the double-inversion pulse pair, the slice-selective pulse is applied to an entire slice pack. The DIR preparation is executed before readout of each slice. Several slices can be sequentially imaged within TR due to shortened TI. The timing of the sequence allows for coincidence of the zero-crossing point of blood and the start of readout for each slice. The magnetization of inflowing blood approaches the pulsed steady state, where TI for zero-crossing point depends on the number of slices (N) and TR:where T1=1200 ms in blood. The multi-slice DIR pulse sequence was implemented on a 1.5T MR scanner (GE Signa EchoSpeed) in combination with a fast spin-echo (FSE) readout. To test flow suppression provided by the multi-slice DIR technique, the proton-density- (PD-) weighted black-blood images of cervical CA were obtained from three healthy volunteers at N ranged from 1 to 8. The common parameters for all scans were. TR 2500 ms, TE 9 ms, echo train 12, NEX 2, matrix 256×256, FOV 14×10 cm, slice thickness 2 mm, scan time 1 min 20 s, 4–12 slices were acquired for each N. TI(N) for scans with different N were 146(8), 190(6), 224(5), 272(4), 346(3), 469(2), and 691(1) ms. Additionally, the FSE scan with inferior and superior flow pre-saturation and with the same parameters of an imaging sequence was acquired for each volunteer. The signal-to-noise ratios (SNR) in the lumina of bilateral common, internal, and external CA at matched locations (42 measurements per N value) were compared among the protocols using paired Student's t-test. To estimate the saturation of steady tissues, the signal intensities (SI) in neck muscles were measured and normalized to the intensities for single-slice scan, SI(1). Clinical tests of the multi-slice DIR technique with N=4 were performed in three patients with carotid atherosclerosis.
Results: Multi-slice DIR with N=2–6 provided almost the same blood suppression as single-slice DIR with no statistically significant difference between scans with N=1–6 (Figs. ). At N=8, suppression of arterial flow became slightly compromised. At all N=1–8, blood suppression by DIR was significantly better than by inflow saturation. For high N=6–8, multi-slice method resulted in a considerable reduction of the signal from stationary tissues (Figs. ), which was associated with specific saturation produced by multiple DIR pulses. Two strategies can be suggested for further clinical applications of multi-slice DIR in carotid imaging. Due to more reliable flow suppression, multi-slice DIR with large N=5–8 can be used instead of inflow-saturated FSE for screening purposes, such as evaluation of obstructed lumen within a long segment of CA. For obtaining high-resolution images with high SNR, which are necessary for precise identification of plaque components and morphologic measurements, the technique can be applied with reduced N=3–4 (Fig. ) and still be much faster than single-slice DIR.
Conclusions: The new multi-slice DIR method provides up to eight-fold reduction of scan time as compared to the standard single-slice DIR. In the high-resolution imaging of CA, four-times improvement of the time-efficiency of DIR can be obtained by using the multi-slice technique with no penalties of impaired blood suppression or image quality.
Figure 1. PD-weighted images obtained from a healthy volunteer using DIR-FSE method with different N and FSE with inflow saturation.
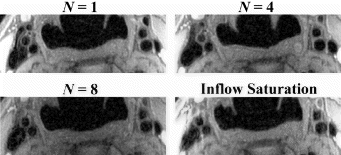
Figure 2. Results of image analysis for DIR-FSE scans with variable N and inflow-saturated FSE: left diagram-flow suppression; right diagram-effect on the signal from stationary tissues.
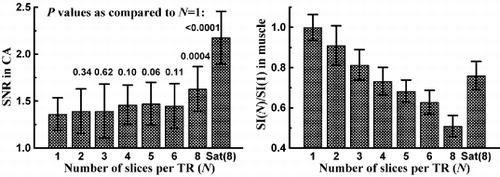
Figure 3. Comparison between single-slice (left column, 5 min 20 s total scan time per four slices) and four-slice (right column, scan time 1 min 20 s) DIR-FSE images obtained from an atherosclerosis patient with 50% stenosis of the right CA.
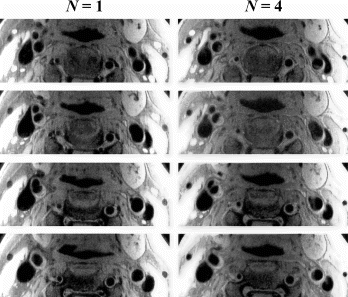
References
1. Edelman RR et al. Radiology 1991; 181:655–660.
2. Simonetti OP et al. Radiology 1996; 199:49–57.
3. Song HK et al. Magn Reson Med 2002; 47:616–620.
4. Parker DL et al. Magn Reson Med 2002; 47:1017–1021.
128. Assessment of Myocardial Viability in Patients with Left Ventricular Dysfunction Using Contrast-Enhanced Magnetic Resonance Imaging. Comparison to Thallium-201 Single Photon Emission Tomography
Matthias Regenfus, MD,1 Christian Schlundt, MD,1 Johannes von Erffa, MD,1 Robert Krähner, MD,1 Dieter Ropers, MD,1 Stephan Achenbach, MD,1 Michaela Schmidt, MS,2 Niels Oesingmann, PhD,2 Kristin Schmiedehausen, MD,3 Guenther Platsch, MD,3 Torsten Kuwert, MD,3 Werner G. Daniel, MD.1
Medical Clinic II, FAU Erlangen-Nuernberg, Erlangen, Germany, Medical Solutions, Siemens AG, Erlangen, Germany, Nuclear Medicine, FAU Erlangen-Nuernberg, Erlangen, Germany.
Introduction: Close correlation of contrast-enhanced (ce) magnetic resonance imaging (MRI) and positron emission tomography for assessment of myocardial viability has been shown recently. But there is no data on correlation of ce MRI and Thallium-201 (TI-201) single photon emission computed tomography (SPECT) as a routine nuclear cardiology tool for clinical viability testing.
Purpose: We compared ce MRI and 201-TI SPECT concerning detection of myocardial viability in patients with left ventricular dysfunction.
Methods: 53 patients (pts) with left ventricular dysfunction (EF 39±15%) who had suffered myocardial infarction (MI) (26 chronic MI, 27 within 7 days of acute MI) were examined on a 1.5T scanner (SONATA, Siemens, Erlangen, Germany). Ce images were acquired 10 min after intravenous injection of 0.1 mmol/kg Gd-DTPA (Magnevist, Schering, Berlin, Germany) using an inversion recovery Turbo FLASH sequence (TE 4.0ms, TR 8.0ms, flip angle 20°, inversion time 220–300ms). Rest-redistribution SPECT was performed according to standard protocols. A 14-segment model of corresponding basal, midventricular and apical slices was analysed independently for MRI and SPECT. Segmental hyperenhancement (HE) for MRI and defect size for SPECT were visually graded using a 5 point score. Segments were categorized as viable or nonviable according to SPECT. Moreover, segmental extent of infarction (SEI) was quantified for MRI.
Results: Viable (78%, 579/742) segments by SPECT showed significantly less SEI compared to nonviable (22%, 163/742) segments (12.3±19.7% vs. 61.1±27.5%, p<0.0001). Summed SPECT defect score and summed MRI infarct score showed close agreement for pts with chronic MI (r=0.8, p<0.0001) and acute MI (r=0.9, p<0.0001). However, SPECT failed to detect 70 of 352 (20%) segments showing HE by MRI, and, on a patient basis, missed 7 of 53 (11%) pts with small MI, which had all been detected by MRI. Moreover, of 163 segments assessed nonviable by SPECT only 83 (51%) showed transmural HE.Conclusions. Ce MRI and TI-201 SPECT show, in overall, a close correlation for determination of myocardial viability. Potential advantage of MRI is the superior spatial resolution which allows for determination of both the transmural extent of myocardial infarction and the detection of small myocardial necrosis.
129. Contrast-enhanced Mri Versus Pet In The Assessment Of Myocardial Viability—prediction Of Functional Recovery After Revascularisation
Peter Hunold, M.D.,1 Katja Brandt-Mainz, M.D.,2 Stephan Knipp, M.D.,3 Holger Eggebrecht, M.D.,4 Jörg F. Debatin, M.D.,1 Jörg Barkhausen, M.D.1
Dept. for Diagnostic and Interventional Radiology, University Hospital, Essen, Germany, Clinic and Policlinic for Nuclear Medicine, University Hospital, Essen, Germany, Department of Thoracic and Cardiovascular Surgery, University Hospital, Essen, Germany, Dept. of Cardiology, University Hospital, Essen, Germany.
Introduction: The “Late enhancement” (LE) concept in contrast-enhanced MRI has been shown to accurately assess myocardial viability in coronary artery disease (CAD). [18F]-FDG-PET is serving as the method of reference in this respect. The value of both techniques has to be proven by the outcome of ventricular function after revascularisation.
Purpose: To evaluate MRI and FDG-PET for the assessment of myocardial viability in CAD and to compare the preoperative prediction of functional recovery with the outcome following coronary artery bypass grafting (CABG).
Methods: 12 CAD patients with impaired left ventricular (LV) function were examined by MRI and PET prior to CABG. MRI follow-up was performed 5 to 6 months after CABG. The MRI (1.5T, Magnetom Sonata, Siemens) functional study using a segmented TrueFISP sequence (TR 3.0ms, TE 1.5ms, FA 60°, slice thickness 8mm) consisted of long axes scans and contiguous short axis scans of the entire left ventricle. 8–15 min after application of 0.2mmol/kg BW of Gd-DTPA, short axes were again scanned with a segmented inversion recovery TurboFLASH sequence (TR 8ms, TE 4ms, TI 200–240ms) to detect LE. LE was classified on a 4-point scale (1=no LE, 2=subendocardial LE:<50% of wall thickness, 3=non-transmural LE: >50%, 4=transmural LE)> based on a 8-segment model in all short axes. PET scans (ECAT HR+, Siemens) were performed following application of 370MBq of [18F]-FDG. The corresponding 8 mm short axis slices were reformatted from the 3D data set. FDG uptake in the same short axis segments was analysed using an analogous scale (1=normal, 2=reduced but >50% of maximum, 3=reduced and <50% of maximum, 4=no uptake). Functional recovery of formerly dysfunctional segments in the follow-up MRI study after CABG was correlated with the prediction of viable (score 1,2) and non-viable myocardium (score 3,4) by both techniques.
Results: Prior to CABG, a total of 1008 myocardial segments were analysed in MRI and PET separately in a blinded manner. 406 of 1008 (40%) segments were classified dysfunctional in cine MRI. Contrast-enhanced MRI revealed LE score 3 and 4 as a sign of scar in 234 segments, PET showed score 3 and 4 in 144 segments. Differences mainly contributed to non-transmural scars, which were more sensitively detected by MRI. After CABG, 150 of 406 (37%) dysfunctional segments improved function. For the detection of viable myocardium with regional functional recovery after revascularisation, the sensitivity and specificity were 95% and 72% for MRI, and 97% and 47% for PET, respectively. Positive (PPV) and negative predictive values (NPV) for regional improvement were 66% and 96% for MRI, and 52% and 97% for PET.
Conclusions: Contrast-enhanced MRI accurately distinguishes scar from viable myocardium, which is mandatory for the prediction of functional recovery after revascularisation in CAD. MRI provides higher specificity and PPV than [18F]-FDG-PET with comparable sensitivity and NPV. These results confirm the superiority of MRI over PET in the assessment of myocardial viability.
130. Peak Cardiac Troponin I Levels Correlate with Infarct Size on Contrast-Enhanced Magnetic Resonance Imaging
W. Patricia Ingkanisorn, MD, Andrew E. Arai, MD.
National Heart, Lung, and Blood Institute, Laboratory of Cardiac Energetics, National Institutes of Health, Bethesda, MD, USA.
Introduction: Both troponin-I (cTn-I) and ejection fraction (EF) can be used for risk assessment after myocardial infarction (MI). In general, both cTn-I and EF should correlate with the size of infarction but many factors such as reperfusion status and myocardial stunning may modulate these relationships.
Purpose: The purpose of this study was to correlate cTn-I and EF with infarct size as measured by MRI in patients after first acute MI.
Methods: MRI was performed in 33 patients with first MI. EF was measured with steady state free precession. Inversion recovery gradient echo methods were used to measure MI size 20 minutes after 0.2 mmol/kg gadolinium DTPA. MI size was quantified using computer-assisted planimetry. Peak cTn-I was measured using an immunometric technique (Fig. ).
Results: 23 patients had acute percutaneous interventions and 10 did not. MRI was performed 2±3 days after presentation. The median MI size was 13 g (range 1–102 g) and median EF was 57% (range 27–76) by MRI. Median cTn-I was 42 (range 2.0–745 ng/ml) overall. The correlation between cTn-I and MI size was. cTn-I=4.9*MIsize-22.9, r=0.80. The correlation between cTn-I and EF was. cTn-I=−4.4EF+320, r=0.37. The correlation between EF and MI size was. EF= −0.33*MIsize+61, r=0.65. Excluding two large but nonreperfused infarcts, the correlation between cTn-I and MI size improved to r=0.91.
Conclusions: MI size, as measured on gadolinium enhanced MRI, correlates closely with peak cTn-I in patients with first reperfused MI. MI size also correlates with EF despite the poorer correlation between EF and cTn-I. For post-MI risk stratification, MRI infarct size may prove to be a prognosticator with less variability than peak cTn-I and EF.
131. Influence of Image Acquisition Timing and of Contrast Agent Dose on the Quantitative Determination of Non-viable Myocardial Tissue Using Delayed Contrast-Enhanced Magnetic Resonance Imaging in Chronic Myocardial Infarction
Steffen E. Petersen,1 Oliver K. Mohrs,2 Georg Horstick,3 Katja Oberholzer,4 Nico Abegunewardene,3 Kordula Ruetzel,3 Joseph B. Selvanayagam,1 Matthew D. Robson, PhD,1 Stefan Neubauer, PhD,1 Manfred Thelen, PhD,4 Juergen Meyer, PhD,3 Karl-Friedrich Kreitner, PhD.4
Ocmr, John Radcliffe Hospital, Oxford, United Kingdom, Cardiology, Cardiololoy-angiology Centre Bethanien (CCB), Frankfurt a. Main, Germany, 2nd Medical Clinic, Johannes Gutenberg University Hospital, Mainz, Germany, Department of Radiology, Johannes Gutenberg University Hospital, Mainz, Germany.
Introduction: Delayed contrast-enhanced magnetic resonance imaging (ceMRI) has been shown to identify areas of irreversible myocardial injury due to infarction (MI) in the acute, sub-acute and chronic phase of MI with high spatial resolution, allowing precise quantification of non-viable myocardium. However, the underlying mechanisms and factors influencing ceMRI image contrast remain to be fully defined.
Purpose: To investigate the influence of contrast agent (CA) dose and image acquisition timing after contrast injection on the quantitative analysis of the size of infarcted non-viable myocardium.
Methods: Patients with a history of chronic (older than 8 weeks) anterior myocardial infarction and typical ECG changes were included (n=9) and two MR scans were performed on consecutive days on a 1.5 Tesla Siemens Sonata system (Fig. ).
On day 1, Cine sequences were performed to identify the area of myocardial infarction via altered regional wall motion (vertical (VLA) and horizontal (HLA) long axis cines and short axis (SA) cines encompassing the entire left ventricle). To follow image contrast changes over time, two SA slices were then chosen for ceMRI imaging and were acquired in an interleaved fashion every other minute until 40 minutes after CA injection (Gadolinium-DTPA, 0.1 mmol/kg body weight=single dose, segmented TurboFLASH, TR/TE 750/4.4 ms, constant TI of 260 ms, slice thickness 6 mm, slice gap 10 mm, FoV 276×340 mm2 Matrix 166×256). To quantify the entire mass of non-viable myocardium, between minutes 20 and 28, SA, VLA and HLA ceMRI images encompassing the entire left ventricle were acquired. To determine the influence of CA dose, on day 2 the protocol was repeated with double dose CA (0.2 mmol Gad-DTPA/kg body weight), using the identical imaging planes and acquisition protocol. Planimetric quantification of the areas of hyperenhancement was performed using Adobe® Photoshop; results were expressed as percentage of the total area (for slices) and total mass (for the entire left ventricle). The Wilcoxon test for paired variables was performed for differences between the CA doses and for differences between the area of hyperenhancement at each time point and the considered “gold standard” of double dose CA at 20 minutes. A p<0.05 was considered significant.
Results: 9 patients were imaged and all showed an area of hyperenhancement in the anterior myocardium. The quantification for the entire left ventricle showed a significant underestimation of infarct size for the single dose group with the images acquired between minutes 20 and 28 (Fig. ; 9.0% vs. 14.2% for single and double dose, respectively (p=0.03)).
After the application of CA there is a steep upslope in the curve (Fig. ) for both CA doses during the first 10 minutes (p>0.05 between groups; p<0.05 for both groups compared to double dose at 20 minutes).
After this upslope the curves reach a plateau phase. 30-40 minutes post CA application the size of hyperenhancement decreases rapidly for single dose compared to double dose (p<0.05 between groups; p<0.05 single dose and p>0.05 for double dose compared to double dose at 20 minutes).
Conclusions: Quantification of infarct size by ceMRI is influenced by CA dose and by timing of image acquisition. Between 10 and 20 minutes after CA injection, there is no significant difference between the two CA doses and to the considered “gold standard” double dose at 20 minutes. Thus, to minimize costs and CA dose, we suggest measuring the infarct size between 10 and 20 minutes after single dose CA injection. Measurements too early or too late lead to a significant underestimation of infarct size. Use of standardized protocols for ceMRI is important for comparison of results obtained at various cMR sites.
132. Myocardial Delayed Enhancement: Determining the Optimal Gadolinium Dose and Delay Time for Infarct Imaging
Cindy R. Comeau,1 Lyn M. Santiago,1 George Dangas,2 David Bolon,3 Ali F. Aboufares,3 Steven D. Wolff.1
Advanced Cardiovascular Imaging, Cardiovascular Research Foundation, Lenox Hill Hospital, New York, NY, USA, Cardiovascular Research Foundation, Lenox Hill Hospital, New York, NY, USA, Lenox Hill Hospital, New York, NY, USA.
Introduction: Cardiac MRI with gadolinium contrast identifies regions of myocardial infarction with high spatial resolution.1 Myocardial delayed enhancement (MDE), can demonstrate the transmural extent of infarction, which has been shown to be an important predictor of recovery of myocardial function following revascularization.2 With this technique infarcts may enhance more than normal myocardium after administration of gadolinium contrast because of a larger compartment into which gadolinium can partition3 and because of decreased perfusion (slower “wash-in’ and “wash-out’). However a review of the literature shows that it is unclear as to what dosage and delay time are optimal for infarct imaging.
Purpose: The purpose of this study was to determine the optimal dose of gadolinium contrast and the optimal delay time for imaging following contrast administration.
Method: This study was performed under Lenox Hill IRB approval. Seven patients with a clinical history of chronic myocardial infarction (>6weeks) underwent 3 MDE studies using a 1.5 Signa CV/i scanner (GE Medical Systems, Milwaukee, WI). The studies were performed on three separate days. Each patient received 0.05, 0.1, and 0.2 mmol/kg of Magnevist (gadopentate dimeglumine, Berlex Laboratories, Wayne, NJ) performed in random order. At each dose, short-axis MDE images were acquired at 5 min, 10 min, 20 min, and 30 min following gadolinium contrast administration. An IR prepped ECG gated gradient echo sequence was employed using the following parameters. TR/TE ∼5.4/1.4 ms, flip angle 20°, FOV 38×38 cm, matrix 256×160 slice thickness 8 mm. The inversion time (TI) was individually optimized for each dose and delay time. Short-axis cine images of the left ventricle were also obtained.
Qualitative Analysis: A total of 21 MDE studies from 7 patients were reviewed independently by 3 blinded readers. A single representative short-axis slice location was chosen for each patient. Twelve MDE images (3 doses×4 time points) of this slice location were randomly displayed on a sheet of film. The reviewer was asked to subjectively determine in which of the twelve images was the infarct most conspicuous. This was defined as the “Standard of Reference (SOR).” Reviewers were then asked to assess which of the remaining 11 viability images were “diagnostically acceptable.” Images were considered “diagnostically acceptable” if the infarct could be detected and if it had substantially the same size and distribution as the SOR. Images were “diagnostically unacceptable” if the infarct could not be detected or if its size or distribution was substantially different.
Quantitative Analysis: The signal-to-noise ratio (SNR) of the infarct was assessed at each of the 3 doses and at each of the four time points. In addition, the contrast-to-noise ratio (CNR. infarct/blood and infarct /viable tissue) was assessed.
Results: Figure shows images from a patient with anterior and inferior infarctions. Readers chose the 10 minute image at the 0.2 mmol/kg dose as the SOR. Cine images (not shown) demonstrated regional wall motion abnormalities that corresponded to both regions of hyperenhancement. Figure shows the qualitative analysis for the entire group of 7 patients. One can see that the 0.2 mmol/kg dose is almost always diagnostically acceptable, the 0.1 mmol/kg dose is sometimes diagnostically acceptable and the 0.05 mmol/kg dose is usually not diagnostically acceptable. The quantitative data support these findings as the higher doses of contrast resulted in higher contrast between infarct and muscle and between infarct and blood (Fig. ).
Conclusion: This initial study suggests that MDE imaging is best performed at a 0.2 mmol/kg dose to accurately determine the location and extent of a myocardial infarction. It also shows that maximal CNR is achieved as early as 10 minutes following contrast administration. Additional studies are needed to assess whether acute infarcts differ from chronic infarcts with regard to the optimal contrast dose and delay time.
Acknowledgment
This study was supported in part by GE Medical Systems.
References
1. Kim RJ, Fieno DS, Parrish TB et al, Cirrculation. 1999; 100:1992–2002
2. Kim RJ, Wu E, Rafael A et al, N Engl J Med 2000; 343:1445–53
3. Arheden H, Saeed M, Higgins C et al, Radiology 2000; 215:520–528
133. Infarct Size Identifies Patients with Substrate for Ventricular Tachycardia
David Bello, MD,1 David S. Fieno, PhD,2 F. Scott Pereles, MD,2 Gina K. Song, BA,2 Andrea Wesson, RN,1 Rod Passman, MD,1 Alan Kadish, MD, FACC,1 Jeffrey Goldberger, MD.1
Department of Medicine, Division of Cardiology, Northwestern University Feinberg School of Medicine, Chicago, IL, USA, Department of Radiology, Northwestern University Feinberg School of Medicine, Chicago, IL, USA.
To evaluate whether infarct morphology identifies patients (pts) with substrate for inducible monomorphic ventricular tachycardia (MVT), 48 pts (age 60±11 years, 56% male) with coronary artery disease undergoing electrophysiologic studies (EPS) had cine and contrast-enhanced (gadolinium) MRI. Planimetry of the contrast images was used to measure infarct mass and surface area by two readers blinded to EPS results.
Results: 21 (44%) pts had no inducible ventricular arrhythmias (No VT), 18 (37%) pts had inducible MVT, cycle length 273±49 ms, and 9 (19%) pts had inducible polymorphic VT or ventricular fibrillation (PVT/VF). Table shows MRI results (in Table *=P<0.005 vs No VT). There were no differences in ejection fraction (EF) among the three groups, but pts with MVT had larger infarcts. By linear regression, infarct size correlates negatively with EF (R2 0.21 to 0.27, p<0.001). Infarct size was a better discriminant of inducible MVT than EF. The findings for PVT/VF were intermediate between those for No VT and MVT, consistent with the nonspecific nature of this induced arrhythmia. Transmural infarct, aneurysms, and infarct location did not discriminate between pts with inducible MVT and pts with No VT.
Conclusion: Infarct size is a better identifier of patients who have MVT than EF. As all current clinically used risk stratification strategies are based on EF, further evaluation of infarct morphology characterization by cardiac MRI is warranted.
134. Prognostic Significance of Unrecognized Myocardial Infarction Detected by Contrast Enhanced MRI
Han W. Kim, MD,1 Dipan Shah, MD,1 Edwin Wu, MD,2 Sheridan N. Meyers, MD,2 Michele A. Parker,1 Kelly M. Choi, MD,1 Francis J. Klocke, MD,3 Robert O. Bonow, MD,2 Robert M. Judd, PhD,1 Raymond J. Kim, MD.1
Duke Cardiovascular Magnetic Resonance Center, Durham, NC, USA, Northwestern University Medical School, Chicago, IL, USA, Feinberg Cardiovascular Institute, Chicago, IL, USA.
Introduction: Prior studies have shown that patients (pts) with unrecognized myocardial infarction (UMI) have a prognosis that is similar to those with recognized MI. Currently, the diagnosis of UMI is based on new Q waves on ECG. Contrast MRI (cMRI) detects both Q wave and non-Q wave MI with high sensitivity/specificity. However, the prognostic significance of UMI detected by cMRI is unknown.
Methods: 100 patients (mean age 63±11, male 82%, avg risk factors (RF) 2.1, mean EF 53±19%) without history of MI, who were referred for coronary angiography, underwent cine and cMRI. Hyperenhancement (HE) on cMRI was considered to represent MI. Q waves were defined by Minnesota code. Pts were followed for a mean of 21±13 months after MRI, during which 11 pts died. Cox regression was used to estimate risk of death associated with traditional RF, anginal and heart failure symptoms, Q waves, EF, angiographic severity of CAD, and presence/extent of HE (Fig. ).
Results: HE was present in 57% pts. The prevalence of HE was 3.9 fold higher than of Q waves (14%). Pts with UMI defined by cMRI and without Q waves had smaller infarcts than those with Q waves (15±13 vs 29±13% LV, p=0.002). The only significant univariate predictor of death (all cause) was extent of HE (p=0.02), although presence of HE (p=0.08), female gender (p=0.06), extent of CAD (p=0.07), and diabetes (p=0.08) approached significance. Actuarial analysis demonstrated that pts with >5% LV HE had increased mortality compared to pts with ≤5% (p=0.02). On multivariate analysis, extent of HE (>5% LV) was the only independent predictor of death (p=0.02) with the adjusted relative risk for extent of HE being 6.7 (95% CI. 1.4-31).
Conclusions: The extent of UMI identified by contrast MRI is an independent predictor of death in patients referred for angiography.
135. Washout Kinetics of Gd-DTPA Differentiates Acute from Chronic Myocardial Infarction in Delayed Enhancement MRI
Hassan Abdel-Aty, Nidal Al-Saadi, Andreas Kumar, Jeanette Schulz-Menger, Daniel Messroghli, Rainer Dietz, Matthias G. Friedrich.
Cardiology, Franz-Volhard-Klinik, Charite, Campus Berlin-Buch, Humboldt University, Berlin, Germany.
Introduction: Delayed enhancement (DE) magnetic resonance (MR) can depict, localize and determine the size and transmurality of both, acute and chronic myocardial infarcts (MI). The observed signal intensity increase of MI depends on altered wash-in/washout kinetics of the contrast agent injected as well as on the increased volume of distribution in the infarcted myocardium. A difference between acute and chronic MI in DE, however, has not been described, although this is to be expected by the different pathophysiology and is clinically relevant.
Purpose: we investigated the clinical utility of a dynamic delayed enhancement approach to measure the rate of contrast washout from the infarcted tissue to differentiate acute from chronic myocardial infarction
Methods: We studied 20 patients with acute (n=10; mean infarct age 3±1d) or chronic (n=10, mean infarct age 16±12 months) MI. 10 healthy subjects served as a control group. All examinations were conducted on a 1.5 T system (Signa CV/i, GE Medical Systems, Milwaukee, USA). A bolus of Gd-DTPA (0.2 mmol/kg BW Magnevist®, Schering AG, Berlin, Germany) was injected. A multi-slice, breath-hold, inversion recovery gradient echo pulse sequence (TR 5.5 ms, TE 1.4 ms, TI 250 ms) was performed every minute for 15 minutes. Regions of interest (ROIs) were defined in segments exhibiting hyperenhancement and in remote myocardium. The signal intensity (SI) was normalized to baseline for each image, and SI over time curves were generated and subsequently fitted to measure the exponential coefficient of decay representing the rate of washout of contrast from acute MI, chronic MI and normal myocardium.
Results: There was no obvious visual difference between acute and chronic infarcts neither before the injection of contrast, nor at any time point after contrast injection.
The exponential coefficient of decay (as a measure of contrast wash out) in controls (−0.61±0.09) was significantly higher than chronic (−0.23±0.03) and acute MI (−0.15±0.05) (p<0.001 for both). The wash out of chronic infarcts was significantly faster than that of acute MI (p=0.04).
Discussion: Delayed washout of Gd-DTPA from infarcted myocardial segments is explained by the increase volume of distribution of the contrast in these segments. The volume of distribution in acutely infarcted segments is significantly higher than in chronically infarcted ones underscoring that the washout characteristics of Gadolinium-DTPA may differentiate acute and chronic MIs (Fig. ).
Conclusion: Our results show that the Gd-DTPA washout kinetics of acute infarcts with myocardial tissue necrosis are different from that of chronic myocardial scar. This difference is important for defining the acquisition time window after the bolus and may help to differentiate acute from chronic myocardial infarcts.
136. Assessment of Function and Viability in Patients with Coronary Artery Disease with DENSE and Delayed Hyperenhancement Imaging
Anthony H. Aletras, Ph.D., Kwabena O. Agyeman, M.D., Wiphada P. Ingkanisorn, M.D., Andrew E. Arai, M.D.
National Heart, Lung and Blood Institute, National Institutes of Health, Bethesda, MD, USA.
Introduction: Displacement Encoding with Stimulated Echoes (DENSE) is a phase contrast imaging method for mapping cardiac motion and contractility. DENSE images allow regional quantitative evaluation of myocardial function (i.e. strain) over systole with minimal user intervention for data processing. Delayed hyperenhancement imaging following double dose Gd-DTPA administration allows visualization of infarcted myocardium with high correlation to histology. Recent reports suggest that wall thickening may remain normal in small regions of myocardial enhancement. Since wall thickening is a transmural measure of regional function, cine MRI might miss subtle abnormalities detectable by a quantitative high-resolution measurement of intramural strain as can be derived with DENSE.
Purpose: The purpose of this study was to quantitatively evaluate regional wall motion with DENSE and standard FISP in normal and hyperenhancing segments of patients with coronary artery disease (CAD).
Methods: Twelve patients with coronary artery disease were imaged with a General Electric CV/i system. Eight patients exhibited hyperenhanced regions 20 minutes after 0.2 mmol/kg Gd-DTPA using phase sensitive inversion recovery gradient recalled echo (PSIR-GRE). PSIR-GRE parameters were. TI approx. 300ms, bandwidth ±31.25 kHz, TE 3.4 msec, TR 7.8 msec, flip 20°, matrix 256×96, FOV 360×270, 8 mm slice thickness, 12 views per segment, acquisition time of 16 heartbeats. DENSE parameters were: encoding interval equal to systole, bandwidth ±31.25 kHz, 2.8×2.8 mm2 in-plane resolution, acquisition time of 14 heartbeats, displacement encoding of 2.0 mm/π, slice 8 mm, echo train length 24, and acquisition window 108 ms. FISP imaging parameters were: bandwidth ±125 kHz, TE 1.6 msec, TR 3.7msec, flip 50°, matrix 192×144, FOV 280×280, slice thickness 8 mm, 12 views per segment.
Hyperenhanced myocardial regions were identified. Wall thickening was measured by epicardial and endocardial segmentation of FISP images. Circumferential shortening (CS) and radial thickening (RT) were measured by DENSE. Similar measurements were performed in a region remote to the hyperenhancement. Average values were computed and student's t-tests (two-tailed) were used to compare enhanced and remote zones. To preserve sample independence, one enhanced and one remote zone only per patient were considered.
Results: A typical short axis slice exhibiting delayed hyperenhancement is shown in Fig. . The corresponding circumferential shortening DENSE map is shown in Fig. while the end-diastolic and end-systolic FISP images are shown in Fig. , respectively. Note that the endocardial surface is obstructed by trabeculations and the papillary muscles in the end-systolic FISP frame, making segmentation difficult. The average CS and RT for enhanced myocardial regions were 0.022 (sdev=0.049) and 0.102 (sdev=0.058), respectively. For remote regions average CS and RT were 0.233 (sdev=0.045) and 0.261 (sdev=0.071), respectively. CS was significantly different in enhanced regions than in remote ones (p<0.001). The same held true for RT (p=0.004). Average regional wall thickening as measured by FISP in enhanced regions was 2.2 mm (sdev=2.2) while in remote regions the thickening was 5.6 mm (sdev=2.8). Wall thickening in enhanced areas was significantly different to that of remote areas (p=0.02).
Conclusions: DENSE strain measurements at 2.8×2.8 mm2 in-plane resolution show that areas exhibiting delayed hyperenhancement show significant functional deficits when compared to remote regions. FISP wall thickening measurements also identified abnormal segments by simple measurements. However, the variance of the DENSE measurements is approximately half the variance of wall thickening measurements from cine MRI. The user's degree of proficiency in excluding trabeculae and papillary muscles in contouring the endocardium is critical to FISP measurements. Also, through-slice motion needs to be considered with FISP as an additional source of error. Automated DENSE measurements of regional contractile function appear promising.
137. An MR Method for Real Time Bicycle Exercise Stress Testing: Response of Right Ventricle to Peak Exercise in Adult Congenital Heart Disease
Amr G. El-Shafei, MD,1 Mary P. Watkins, RT(R)MR,1 LaTish McKinney, RN, BSN,1 Todd A. Williams, RT(R)MR,1 Katherine A. Lehr, RN,1 Shelton D. Caruthers, PhD,2 Ruud deBoer, PhD,3 Samuel Wickline, MD.1
Cardiovascular MR Laboratories, Washington University School of Medicine, St. Louis, MO, USA, Cardiovascular MR Laboratories, Washington University School of Medicine and Philips Medical Systems, Best, The Netherlands, St. Louis, MO, USA, Philips Medical Systems, Best, Netherlands.
Introduction: Evaluation and characterization of congenital heart disease is a common clinical indication for cardiovascular MR (CMR). In particular, quantitative assessment of right ventricular function as in patients with congenital heart disease is a common request for noninvasive imaging. Because CMR allows the acquisition of true RV short axis images that encompass the entire RV with high spatial and temporal resolution, highly accurate quantitative RV mass and functional data are available. However, the ability to impose a stress on the ventricle while assessing function often optimizes detection of contractile dysfunction.
Purpose: The objective of this project is the development of a rapid, simple and routine CMR stress test using an MR-compatible bicycle ergometer for evaluation of right ventricular function in patients with congenital heart diseases.
Methods: Patients with selected congenital heart diseases were included in the study and subjected to the following bicycle stress protocol, which was developed on 9 test subjects. Patients were subjected to a routine pre-stress interview and examination. standard exclusions for stress testing were applied. A “Predicted Maximum Heart Rate” (PMHR) was calculated as 85% of maximal hear rate (220-age×0.85), and was used as the end point for the stress test, unless another end point supervened such as shortness of breath, chest pain, leg cramps, etc. A twelve lead EKG was recorded before the stress test. In the scan room, a 4 lead VCG was positioned and a soft respiratory sensor was placed around the subjects' waist for synchronization of the scans and for monitoring. Patients were positioned on the MR scanner tabletop in a position to pedal a specially designed stationary nonferromagnetic bicycle ergometer that was affixed to the tabletop. The tabletop was then introduced into the magnet and pre-stress images were acquired according to a set protocol (see below). The tabletop and patient were then partially removed from the scanner to permit maximal exercise outside of the magnet bore while retaining positional information within the MR system.
Stress Protocol: Patients were asked to pedal the bicycle while lying supine on the scanner tabletop (Fig. ). The speed and resistance of the bicycle was increased every 3 minutes until the limits of fatigue, breathlessness, chest pain or other symptoms occurred. A nurse and physician monitored the test at all times. During the stress portion of the study, the subjects' heart rate, blood pressure, oxygen level and respiratory rate/rhythm were continuously monitored. Once the subject had attained PMHR, post stress images were recorded by quickly returning the patient on the tabletop into the magnet bore while still pedaling. This set of images required approximately 1.5 minutes to obtain. After MR images were obtained, the subject were removed from the scanner bore and recovered on the MR tabletop. A twelve-lead EKG was obtained immediately after their recovery period.
Imaging technique: “Real-time” MR imaging was performed using a 1.5T clinical scanner (Philips Intera CV, Best, the Netherlands) and a dedicated five-element phased-array cardiac coil. Three short axis views, a horizontal long axis and a vertical long axis view were obtained at baseline and immediately after peak exercise. Images were obtained using a balanced turbo field echo technique (bTFE) employing the SENSE technique with TR=2.4ms, TE=1.2ms, FOV=350mm, and reconstructed matrix. 77/128. The image acquisition was repeated as 200 dynamic scans, with a temporal resolution of 64ms per dynamic, for each of the aforementioned views. Interactive plan scanning was used to establish the scan geometries, which were stored for immediate activation by remote triggering at the scanner upon completion of the exercise.
Results: Four patients with congenital heart disease comprised the test set following protocol development on 9 patients. Patients' characteristics are shown in the Table . These patients were all clinically stable and well compensated, and have been followed routinely in the Adult Congenital Heart Disease Clinic at Washington University School of Medicine. The exercise response for the RV was graded subjectively as “improved” in all cases by a blinded reader.
Figure illustrates the effect of exercise on RV Ejection Fraction (EF). Volumes/areas were measured offline on a Philips EasyVision platform according to standard procedures. In general, for these well compensated cases, ejection fractions all improved markedly (P<0.003). The RV end-diastolic volumes increased by 14% (p=0.18) and the RV end-systolic volumes decreased by 23% (p=0.3).
Although these volumes were computed from a limited set of cross sections (3 short axis views) and might underestimate actual absolute values, the data are quantitative, objective and can be acquired in real time.
Conclusion: This study confirmed the capability of CMR to perform real time bicycle stress testing as a simple and rapid technique for evaluation of RV function in patients with congenital heart disease. Future improvements (e.g., obtaining more short axis views) are envisaged to improve absolute volume estimates.
138. In Vivo Quantitation of High-Speed Flow Jets in a Single Breath Hold
Krishna S. Nayak, Ph.D.,1 Miriam Amitai, M.D.,2 Michael V. McConnell, M.D.,2 Bob S. Hu, M.D.,3 Dwight G. Nishimura, Ph.D.1
Electrical Engineering, Stanford University, Stanford, CA, USA, Cardiovascular Medicine, Stanford University Medical Center, Stanford, CA, USA, Cardiovascular Medicine, Palo Alto Medical Foundation, Palo Alto, CA, USA.
Introduction: Flow jets containing velocities up to 5–7 m/s are common in patients with congenital defects and patients with valvular disease (stenosis and regurgitation). The quantification of peak velocity and flow volume in these jets is clinically significant but requires specialized imaging sequences. Conventional sequences are either too slow (2DFT) [1], or are highly corrupted by flow artifacts at these velocities (spiral/EPI with long readouts) [2] due to both phase dispersion and motion during excitation and readout. We present a new CINE gated method based on short-TR spiral phase contrast, which has a sufficiently short measurement interval (<4 ms) to minimize flow artifacts, while achieving high spatial resolution (2×2×4 mm) to minimize partial volume effects, all within a single breath hold. Simulations indicate this technique is capable of imaging through-plane flow jets upwards of 10 m/s, and initial in vivo studies in aortic stenosis patients show accurate measurement of jets up to m/s.
Purpose: To develop an imaging technique capable of measuring 5–7 m/s flow jets in short breath holds (5 to 10 seconds).
Methods: The main design considerations for flow jet imaging using phase contrast are: 1) partial volume effects, 2) distortion from through-plane motion during the excitation, and 3) shifts and distortion due to in-plane motion during the readout. We dealt with these by: 1) designing for high spatial resolution (2 mm) using interleaved spirals, 2) using an ultra short (0.48 ms) excitation, and 3) minimizing the echo time and using short (2.2 ms) spiral readouts. The pulse sequence used a 0.64 to 0.96 ms bipolar pulse for flow encoding, used a 20 degree flip angle, and achieved a TR of 5.5 ms. Bloch simulations revealed that this sequence was capable of imaging through-plane velocities up to 12 m/s without signal loss, and in-plane velocities up to 2.5 m/s with less than one pixel of displacement and minimal blurring (shown in Fig. ).
In-vivo experiments were performed on a GE 1.5T Signa CV/i scanner. Localization was done using a real-time color flow MRI system [2]. 20-interleave spirals were used to achieve 2 mm resolution over a 20 cm FOV. CINE acquisitions were performed over 5- or 10-heartbeat breath holds achieving 44 ms or 22 ms resolution respectively. Acquisitions were ECG triggered. Images were displayed using a color overlay analogous to ultrasound. In addition, time-velocity histograms were generated by plotting histograms of velocity within operator-defined regions of interest (ROIs) covering the aorta.
Results: Two normal volunteers and three aortic stenosis (AS) patients were scanned in an initial study. Figure contains sample images from two studies. Frames are shown spanning the first 300 ms of systole. Notice that coherent signal from approximately 4.2 m/s patient jets were achieved. The ROI-based time-velocity histograms for the three patients were comparable to echocardiography in both peak velocity (within 5%) and waveform.
Conclusions: The quantitative imaging of high-speed flow jets is possible in short breath holds using CINE short-TR spiral phase contrast. Simulations indicate that the accurate measurement of through-plane flows up to 10 m/s is possible. Preliminary in-vivo results have shown agreement with echocardiography in a cohort of patients with jets up to 5 m/s. A blinded patient study is currently underway at our institution.
Figure 1. Illustration of the minimal flow distortion: (top) effective slice profiles based on high-speed through-plane flow, and (bottom) velocity point spread functions based on moderate in-plane flow.
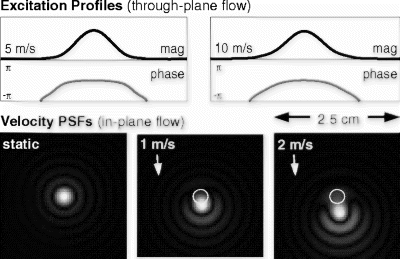
References
[1] Kilner PJ, et al. Circ 1993; 87:1239–1248.
[2] Nayak KS, et al. MRM 2000; 43:251–258.
139. A Novel Method of Estimating Pulmonary Vascular Resistance Utilizing Invasive Pressure Monitoring and MRI Flow Data
Vivek Muthurangu, MBChB(hons),1 Rado Andriantsimiavona, Msc,2 Derek L. G. Hill, PhD,3 Robert Tulloh, MBBS,4 Reza S. Razavi, MBBS.5
Division of Imaging, King's College London, London, United Kingdom, Division of Imaging, King's College, London, United Kingdom, Division of Imaging, KIng's College, London, United Kingdom, Congenital Heart Disease, Guy's Hospital, London, United Kingdom, Congenital Heart Disease, King's College/ Guy's Hospital, London, United Kingdom.
Introduction: Pulmonary vascular disease (PVD) is significant and ultimately fatal sequelae of many congenital heart defects. It is also fundamental in the pathophysiology of both primary pulmonary hypertension and cor pulmonale secondary to pulmonary disease. Physiologically PVD is assessed by calculation of pulmonary vascular resistance (PVR), which increases as PVD progresses. Current practice in clinical situations is to calculate pulmonary vascular resistance using invasive pressure measurements and pulmonary blood flow derived by Fick's principle. Fick's principle requires estimation of oxygen consumption and invasive measurement of oxygen saturations in the heart and vessels. This method is prone to errors firstly because oxygen consumption is estimated and secondly because where there is intra-cardiac mixing saturations values can be misleading (1). As the accuracy of the PVR is dependant on accurate flow estimation significant management decisions are currently being made using inaccurate information. It is therefore imperative that an accurate and safe method is developed to measure pulmonary flow and therefore resistance. Phase contrast MRI Allow the quantification of blood flow in major vessels (2).
Purpose: We have developed a novel method using simultaneously acquired invasive pressure measurements and MRI flow quantification In order to calculate pulmonary vascular resistance. We compare PVR calculated by both the traditional and new method in 5 patients. In addition we have been able to assess flow in both pulmonary arteries allowing calculation of individual lung vascular resistances.
Methods: 5 patients with congenital heart disease underwent simultaneous MRI imaging and cardiac catheterisation, in a XMR suite (1.5T Philips Intera I/T magnet and Philips Pulsera cardiac X-ray in a single suite), under general anaesthetic. In 2 patients response of PVR to nitric oxide was also studied. The unique characteristics of the XMR facility allowed catheter placement either under MRI guidance or using a combination of X-ray and MRI guidance. MRI visualization of catheter was achieved using a Swan-Ganz catheter balloon filled with carbon dioxide as a contrast agent. 40 phase contrast images (through plane flow) per cardiac cycle were acquired using retrospective gating. Images were acquired either at the midpoint of the pulmonary artery or at the mid point of SVC in patients with Glenn shunts. In addition phases contrast images were acquired at the mid point of the left and right pulmonary artery where possible.
Invasive pressure and oxygen saturations were measured using current standard protocols for cardiac catheterisation. Phase contrast data was analysed using ‘Flow, Medis, NL’, mathematical analysis was accomplished using Matlab.
Results: Table compares PVR calculated using MRI flow data and Fick's principle in all the patients. Table shows a summary of clinical details for patients in this study. Table shows analysis of data from right and left pulmonary artery in patient 1.
Conclusion: We have shown that it is possible to estimate PVR using simultaneous invasive pressure measurements and MRI flow data. This novel approach is dependant on the unique properties of a XMR suite and has therefore not previously been described. Our results show a large discrepancy between pulmonary vascular resistance calculated using Fick's principle and MRI flow quantification. This is most apparent in patients with complex congenital heart disease where intracardiac blood mixing makes Fick's method less accurate. The clinical implications are significant as such a discrepancy may be the difference between surgical correction or palliation and a more conservative approach.
As already stated pulmonary flow estimated using Fick's principle contains large errors (1) especially in patients with complex congenital heart disease. Although there is no gold standard measurement of flow, phase contrast MRI has been rigorously validated in vivo and operates with a known accuracy (2) making it a more effective in quantifying flow than Fick's method. The combination of MRI derived flow data and invasive pressure monitoring (presently the gold standard for pressure measurement) allows an accurate estimation of PVR.
In addition we have been able to acquire information regarding flow in the right and left pulmonary artery. This has enabled calculation of vascular resistance in each lung, previously not described. Future improvements in MRI visible catheters will hopefully allow this procedure to be undertaken purely under MRI guidance.
Table 1. Comparison flow estimation using MRI and Fick's principle (pulmonary vascular resistance=transpulmonary pressure/flow)
Table 2. Clinical details of patients in study
Table 3. Estimation of resistance in each lung using MRI flow data and invasive pressure from patient 1 (RPA, right pulmonary artery; LPA; left pulmonary artery; VR, vascular resistance)
References
1. Spirometric versus Fick-derived oxygen consumption. which method is better? Crit Care Med 1996 Jan 24(1):91-5, Thrush DN.
2. Precision of Magnetic Resonance Velocity and acceleration Measurements. Theoretical Issues and Phantom Experiments. Journal of Magnetic Resonance Imaging 2001 13:445-451, Durand E et al.
140. The Hybrid Interactive Magnetic Resonance Imaging (iMRI)/X-Ray Suite Is a Cardiovascular Interventional Procedure Development Tool
Kendrick A. Shunk,1 Oliver M. Weber, PhD,2 Maythem Saeed, DVM,2 Alastair J. Martin, PhD,3 Charles B. Higgins, MD.2
Medicine-Cardiology, UCSF, San Francisco, CA, USA, Radiology, UCSF, San Francisco, CA, USA, Philips Medical Systems, Best, Netherlands.
Introduction: Hybrid-configuration of an iMRI system with X-ray angiography capabilities enables the development of therapeutic percutaneous cardiovascular procedures which depend on both modalities for their efficient development but may ultimately be solely iMRI-guided.
Purpose: To test the hypothesis that as a model interventional cardiovascular procedure, iMRI-guided percutaneous coronary intervention/stenting (PCI) can be subdivided into its constituent sub-procedures, each of which can be refined and performed in sequence using iMRI for primary imaging, and on-line X-ray imaging as a complementary modality to enhance the iterative process of stand-alone iMRI-guided technique development.
Methods: A hybrid iMRI/X-ray suite (XMR, Philips) was used to define a procedural technique for iMRI-guided PCI. A cast-silicone phantom of the human aorta and coronary tree and anesthetized canine models were used to evaluate the MR-suitability of candidate wires, catheters, and stents. Key procedural steps were defined and appropriate imaging protocols selected. 1) Selection of appropriate planes to serve as working views from a high-resolution coronary MR angiogram (T2-prepared segmented fast gradient echo sequence), 2) MR “Fluoroscopic” guidance of coronary guide catheters over a wire and into the ascending aorta using real-time radial steady state free precession with sliding window reconstruction (15 frames/s, <200 ms latency), 3) Engagement of each coronary artery, 4) Confirmation of engagement by intra-coronary Gd-enhanced real-time angiography (T1-enhanced gradient echo sequence), 5) Coronary wire placement, 6) Stent advancement and deployment, and 7) High-resolution post-stent imaging. Complementary gold-standard x-ray coronary angiography was performed. After stent placement the animals were sacrificed and the hearts harvested for gross examination (Fig. ).
Results: We identified 7 key steps in iMRI-guided PCI. Using iMRI guidance as the sole imaging modality, 6 of these could be performed reliably and sequentially by an experienced interventional cardiologist, compared with 7 when X-ray was utilized in tandem. The need for X-ray was driven by poor guide catheter suitability, leading to inability to safely advance the stent and closely monitor its advancement without losing guide engagement of the vessel. In one case, X-ray angiography, high-resolution MRI and gross pathology demonstrated evidence of catheter-induced vessel trauma that was evident on iMRI only in retrospect.
Conclusions: 1) Using a hybrid iMRI/X-ray procedural suite as a development tool and iMRI-guided PCI as a model cardiovascular procedure, constituent sub-procedural steps were identified. 2) This approach allows efficient targeting of subsequent efforts. 3) A lack of availability of highly evolved MRI-compatible catheters and interventional devices emerged as a particularly rich area for further development.
141. A Fast, TI Insensitive Infarct Imaging Technique
Yiu-Cho Chung,1 Peter Kellman, Ph.D.,2 Daniel Lee, M.D.,3 Orlando Simonetti, Ph.D.1
Siemens Medical Solutions USA, Inc., Chicago, IL, USA, National Institute of Health, Bethesda, MD, USA, Northwestern Memorial Hospital, Chicago, IL, USA.
Introduction: Following myocardial infarction, the extent of irreversible myocardial injury has important prognostic implications. In MRI, the delayed enhancement technique can distinguish between viable and non-viable myocardium [1]. Segmented inversion recovery turbo FLASH (IR-TFL) has been shown to give the best contrast between viable and infarcted myocardium [2]. However, the segmentation technique requires breathholding and a regular RR interval for good image quality. Single shot inversion recovery trueFISP (IR-trueFISP) gives good image quality independent of heart rate variation [3] but complete nulling of normal myocardium is difficult due to the long acquisition window, resulting in reduced contrast between normal and infarcted myocardium. The TI scouting method [4] may be used to optimize TI but it also depends on a good breathhold and regular cardiac rhythm. A MR infarct imaging technique insensitive to both TI and RR interval variation is highly desirable for arrhythmic patients.
Purpose: To design an arrhythmia and TI insensitive sequence for infarct imaging on a clinical MRI scanner.
Methods: The sequence: The acquisition of the IR-prep image was based on the single shot 2D IR-trueFISP sequence [3]. Phase sensitive reconstruction (PSRecon) as proposed in [5] was used to make the method insensitive to TI by eliminating the need for precise nulling of normal myocardium. Phase information needed in PSRecon was obtained from a reference image acquired in the second (or later) heart beat, performed with identical gradient structures as the IR-prep image except that a low flip angle (8° in this case) was used. The low flip angle acquisition gives a reference image that is nearly proton density weighted. In both acquisitions, the linear flip angle method was used for steady state preparation before echo collection. Asymmetric echoes are used to shorten TR and hence enable the collection of more lines per shot. Figure shows the sequence timing.
Patient imaging:
5 patients with known infarcts were imaged using the two sequences described above. All patients gave written informed consent. The protocol was approved by the IRB of Northwestern University. After initial scout images were obtained, cine images were acquired for multiple short axis (8–10 slices) and two long axis (2-chamber and 4-chamber) views to evaluate contractile function. Gadoteridol (0.1 mmol/kg of Prohance from Bracco) was then administered intravenously. Images were acquired for identical views ∼10 min after contrast injection using the IR-TFL sequence. PSRecon IR-trueFISP was then used to image the slices where infarcts were present. Parameters used in the sequences were: IR-TFL: ECG trig, 124 lines, 25 segments, FOV=∼270mm×360mm, slice=6mm, flip angle=30°, TE/TR=4.3ms/11 ms, TI=300–400ms, bandwidth/pixel=140 Hz, gradient refocused, image matrix=2562. Lines acquired every other beat. Scan time=10 beats. PSRecon IR-TrueFISP: ECG trig, ∼100 lines, single shot, FOV=∼270mm×360mm, slice=6–8mm, flip angle=30°–50°. TE/TR=1.2ms./2.7ms, TI=∼300ms, bandwidth/pixel=980Hz–1200Hz depending on heart rate, image matrix=2562.
Nonselective IR pulses were used in both cases to avoid flow artifact. Acquisitions were timed to occur at the diastole and the window was kept to about 275ms.
Results: Infarcts in all 5 patients showed up very well in images acquired using the sequence in Fig. . Figure shows a set of typical images from this technique obtained from a patient with acute MI. The conventional IR-TFL image, obtained with careful choice of TI to null normal myocardium, showed the infarct and the no-reflow region (Fig. ). Figure was obtained from the single shot IR-trueFISP with an TI shorter than it should be for nulling of normal myocardium. Note the reduced contrast and signal variability in the normal myocardium. Figure shows the PSRecon of the same data set with the help of the reference image (Fig. ) using the new technique. The infarcts and the no-reflow zone were shown with uniform nulling of viable myocardium.
Conclusion: The PSRecon IR-trueFISP acquired images comparable in quality to IR-TFL without the requirement of precise TI selection for myocardial nulling or uniform R-R intervals over multiple heart beats. The short acquisition time makes this sequence less susceptible to variations in R-R interval (e.g., PVC's) than the standard segmented technique. Additionally since the scan time of the technique is so short and the TI does not need to be changed from one scan to the other, repeated acquisitions can be performed quickly and easily. These properties of PSRecon IR-trueFISP may make it useful in the screening of patients for myocardial infaction.
Figure 1. The phase sensitive reconstruction IR-trueFISP sequence. Both IR-trueFISP and IR-TFL were implemented on a MAGNETOM Sonata (Siemens, Erlangen, Germany, max. gradient=40mT/m, max. slew rate=200mT/m/ms). Image reconstruction was performed online.

References
[1] Kim RJ et al., Circulation 100(9). p.1992–2001, 1999.
[2] Simonetti OP et al., Radiology 218. p.215–223, 2001.
[3] Chung et al., JCMR vol.4, no.1, p.12, 2002.
[4] Chung et al., Proc.10th ISMRM Scientific Meeting, p.219, 2002.
[5] Kellman P et al., Med Reson Med. Vol.47, p.372–383, 2002.
142. Simulations for Optimization of Design Parameters for Intravascular Imaging Microcoil Construction
Eddy Y. Wong,1 Claudia M. Hillenbrand, Ph.D.,2 Jonathan S. Lewin, M.D.,2 Jeffrey L. Duerk, Ph.D.2
Department of Biomedical Engineering, Case Western Reserve University, Cleveland, OH, USA, Department of Radiology, University Hospitals of Cleveland, Cleveland, OH, USA.
Introduction: With the evolution of magnetic resonance imaging (MRI) into a therapeutic tool for minimally invasive procedures, there is an increased need for accurate visualization of the vasculature. These needs are particularly acute in vessel wall imaging for disease identification and visualization for surgical procedures. In these procedures, where identification of pathology is based on varying grayscale intensities, an imaging coil with no signal drop-off or spatial inhomogeneities, which might otherwise be mistaken for pathology, is critical. There exist several antenna designs for intravascular imaging (e.g., dipole antennas, single loop antennas and antennas of the opposed solenoid design). In this project we seek to identify key design parameters and then optimize the design of an MRI intravascular microcoil. The opposed solenoid design was chosen for investigation here because it offers good radial homogeneity, which is important for applications aimed at characterization of the vessel wall or locating vulnerable plaque. Due to the complexity and lengthy process require for construction of a catheter-based imaging coil, simulations are first performed in order to determine the coil parameters that provides the optimal SNR and field homogeneity.
Methods: Biot-Savart simulations were performed using MATLAB. B-field components were calculated for prescribed imaging planes with respect to the location of the coil. A separate text file containing individual wire elements for the opposed solenoid coil was created and then altered for the different design scenarios to be simulated. Four different variables and their effect on the B-field were examined; they were the total number of windings, diameter of the solenoid, pitch of the solenoid windings and the separation distance between the opposed solenoid coils.
Guided by the simulation results, an opposed solenoid imaging coil was created and mounted on a 5-French catheter. Each solenoid consisted of 5 windings of 30 AWG copper magnet wire with an inter-winding spacing of approximately one wire diameter. The opposed solenoid elements were place 10 mm apart and the pair of elements was tuned and matched using surface mount capacitors. Micro-coaxial cable was utilized to provide capacitive coupling to the MR receiver. A picture of the device is shown in Fig. . Imaging experiments were conducted using a 1.5 T Siemens Sonata clinical scanner on a porcine model. The catheter was introduced through the femoral artery and imaging was performed both in vivo and in situ.
Results: Simulation results show the following trends: (1) a direct relationship between radius and Bxy (Fig. ) (2) a direct relationship between number of turns and Bxy (Fig. ) (3) a parabolic relationship between pitch and Bxy with a maxima occurring at a spacing equal to that of one diameter of the wire (Fig. ) (4) and a parabolic relationship between maximum radial imaging depth and coil separation with a maxima at approximately 2 to 3 times the diameter of the coil (Fig. ). Experimental imaging shows details of the vessel wall being resolved (Fig. ).
Discussion: The results of the Biot-Savart simulations follow the trends prescribed by the equations defining the B-field of a solenoid. Inside a solenoid, the equation for calculating a B-field is given as B=(μ0IN)/L. From this formula, a direct relationship between the number of turns (N) and B is observed while an inverse relationship between the pitch of the windings, which relates to overall length (L), and B is also observed. For calculating the field outside of the solenoid, which determines how far out in the radial direction an imaging coil can be used, the following equation applies B(z)=(μ0IA)/(2πz3). Here, a direct relationship between the diameter of the coil and B is observed. Two of the simulation's variables show increases without bounds and are limited by physical constraints. The diameter of the solenoid is ultimately limited by the dimensions of the vasculature to be investigated. While maximizing device size, it is also important to maintain sufficient space for avoiding issues such as vessel occlusions and/or loosening plaque components. The number of windings of the solenoid is also subject to physical limitations. These include the increase in resistance that comes from increased use of wire necessary to increase loops, which lowers the overall Q of the circuit. However, the effect of solenoid resistance on coil Q is not taken into account this simulation. The inductance of the solenoid also increases as the number of windings increases. In order to create a resonant circuit, the inductor must be matched with a capacitor to define the resonant frequency. A balance must be struck with the capacitor and extreme values should not be utilized in a circuit. Therefore in all practicality, 5–10 windings are used to maintain this balance. A parabolic relationship is observed when altering the pitch of the windings, with a maximum occurring at an inter-loop spacing of one wire diameter. Less spacing results in field line cancellation between winding elements, while increasing the spacing beyond optimum results in the loss of flux linkage between the winding elements of the solenoid. Finally, when considering the spacing of the two coils in an opposed solenoid configuration, a maxima is seen when the coils are placed at a distance approximately two to three times that of the diameter of the solenoid coil. The distance between the opposed solenoid coils should be maximized to increase the length of vessel that can be imaged without necessitating the repositioning of the imaging coil. However, too great of a separation distance will result in signal losses due to lack of coupling between the two solenoid coils.
Conclusions: Biot-Savart simulations serve as a useful design aid to optimize parameters for intravascular imaging coil construction. The opposed solenoid configuration for a micro-imaging coil is one that provides good radial homogeneity, which is requisite in identification and characterization imaging procedures. The inline nature of the opposed solenoid coil also allows easy integration onto interventional devices, such as catheters. Imaging coils constructed from results obtained through simulations demonstrate the ability to resolve vessel wall structure.
Figure 2. Images from the conventional IR-TFL (a), the magnitude reconstructed IR-trueFISP (with TI=300 ms) (b)-note the reduced contrast between infarct and normal myocardium, as well as the signal variability, the corresponding PSRecon IR-trueFISP (c) obtained using the coil sensitivity map (d) collected from the low flip angle trueFISP acquisition.
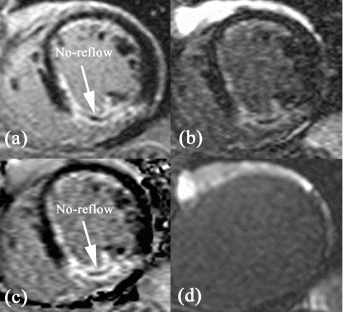
143. Relaxation Rate Kinetics in Blood, Normal Myocardium, and Infarct Tissue as a Function of Time After Contrast Injection
Puneet Sharma,1 John N. Oshinski, PhD,2 Salil J. Patel, MD,3 Josh Socolow, MD,3 Roderic I. Pettigrew, PhD, MD.2
Biomedical Engineering, Georgia Institute of Technology, Atlanta, GA, USA, Radiology, Emory University, Atlanta, GA, USA, Cardiology, Emory University, Atlanta, GA, USA.
Introduction: Myocardial infarction (MI) can be visualized with MRI and the contrast agent Gd-DTPA using delayed contrast-enhancement imaging. The method utilizes an inversion recovery (IR) pulse to enhance the image contrast between the MI, where Gd-DTPA has accumulated, and viable tissue, where concentrations of the agent are lower. The IR pulse is executed a certain “delay time” (TI) prior to image acquisition. The selection of TI is based on the user's intent to completely null the signal from viable tissue, so that the MI will appear significantly brighter, producing optimal image contrast. The ability to null the signal in this manner is based on the relaxation rate (R1=1/T1) of the tissue of interest. Since Gd-DTPA is constantly being circulated and eliminated by the body, R1 varies with time and contrast concentration. As a result, the selection of TI becomes dependent on the time after injection, and will vary between patients, since individual physiology differs. Many users resort to educated guesses to pinpoint the correct IR delay time. This imposes constraints on both imaging efficiency and accuracy.
Purpose: The purpose of this study is to quantify relaxation rates (R1) in normal myocardium, blood and MI after Gd-DTPA injection in order to: 1) determine the variability that exists in selecting the inversion time (TI) at selected time points; and 2) to establish whether these tissues can be distinguished uniquely. This examination intends to determine the range of TI values to suppress normal myocardium, making it easier for users to decide what TIs to use at specific times.
Methods: Nine patients with with prior MI as diagnosed by elevated cardaic enzymes (CK, CKMB, Troponin I), ECG, and catheterization were examined in this study. Each individual was imaged using a 1.5T Philips Intera system using a cardiac phased array coil.
R1 relaxation rates were measured using an inversion recovery segmented “balanced” gradient echo sequence (TR/TE 3.4/1.3ms, flip angle 40, TFE segments 42, spatial resolution 1.4×1.4×8mm3). The protocol was executed twice using inversion delay times of 100 and 500 ms. Each scan was a breath hold image of about 12–15 seconds depending on the patient's heart rate. From the two IR scans, region of interest (ROI) measurements were taken of normal myocardium, infarcted tissue and blood. The signal intensities (SIs) recorded from each of the two scans represented two points on a R1 relaxation curve corresponding to the two selected delay times (100 and 500 ms). By rescaling and dividing the two SIs, one can determine R1 within 5% with the aid of an in-house computer program (Matlab). Three time points after Gd injection (.1 mmol/kg) were investigated. immediate following injection, 10, and 20 minutes.
To determine the corresponding TI range to null normal myocardium at each time point (immediate, 10, 20 min), the high, low, and mean R1 of normal myocardium over all patients were determined. A simulation routine was performed (Mathematica, Wolfram) to determine the TI needed to null normal myocardium if a delayed enhancement sequence was used. The following assumptions about the delayed enhancement sequence were made: 1) the center of k-space was not acquired until the 6th heartbeat (linear acquisition); 2) one beat interval between inversions; 3) constant heart rate of 70bpm; and 4) R1 relaxation was mono-exponential and not deterred significantly by previous image acquisitions.
Results: R1 values of blood, infarcted tissue and normal myocardium were not distinguishable from one another immediately after Gd injection (p<.01); however, normal myocardium was uniquely distinguishable from blood and infarct at both 10 and 20 minutes post-contrast (p>.05) (Table ). Immediately after injection, the R1 values have high standard deviations (SDs) since the contrast agent still exists at high concentrations, and concentration is changing rapidly. Standard deviations tend to reduce over time, but there is still significant variance between the patients studied, which indicates physiological influences on R1 time courses. The corresponding TIs needed to null normal myocardium exhibit similar behavior (Fig. ). Immediately after injection, the TI range to null normal myocardium is largest (177–256ms), based on the broader distribution of R1 values of normal myocardium. The TI range decreases as one moves to the higher time points (224–278ms and 262–279ms, for 10 and 20 min, respectively). This allows a more directed selection of TI at these time points.
Conclusions: This study suggests that individual patient physiology may play a significant role in selecting the delay time needed to suppress normal myocardium. A large variance in R1 and TI range exists even at 10 and 20 minutes post contrast. This study also suggests that a more directed method for TI selection is possible if R1 time courses are known. From the viewpoint of imaging efficiency, there is clinical relevance in having known ranges of TIs at various time points. The data in this study shows that a generalized TI range is not possible for all time points; rather, smaller ranges exist and need to be specified. Smaller TI ranges are more common 10 minutes or more after contrast injection, with values remaining below 280ms.
Figure 3. Experimental in situ imaging results with opposed solenoid coil. The coil was placed in the femoral artery of a pig and imaged using a spin echo sequence.
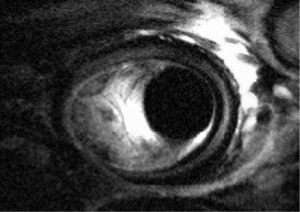
Table 1. R1 values (sec^-1) of blood, normal myocardium, and infarct tissue
144. Qp/Qs in Atrial Septal Defects by Cardiac Magnetic Resonance Imaging Is Comparable to Qp/Qs by Cardiac Catheterization
Uzma Iqbal,1 Anthon R. Fuisz,2 Lowell Satler,2 Augusto Pichard,2 Srirama Swaminathan,2 Sue Apple,2 Ellen E. Pinnow,2 Joseph Lindsay.2
Cardiology, Wasington Hospital Center, Washington, DC, USA, Cardiology, Washington Hospital Center, Washington, DC, USA.
Background: Right heart cath (RHC) with O2 saturation measurements is the diagnostic method of choice for assessment of intracardiac shunts. We hypothesized that cardiac magnetic resonance (CMR) acquisition combining phase velocity mapping (PVM) and biventricular stroke volume (SV) measurements could identify pts with a shunt requiring closure i.e. Qp/Qs of >1.5.
Methods: We analyzed 16 consecutive pts undergoing evaluation of an atrial septal defect (ASD) in whom complete RHC and CMR data were available. Using a Philips Intera CV system, short axis views of both ventricles were acquired using a breath-hold steady state free precision technique. Flow through the aorta was measured using a PVM sequence containing 50-70 phases with a velocity encoding of 200–250 cm/s, and a transverse plane that contained the ascending aorta. Pulmonary flow was measured by choosing a plane perpendicular to the main pulmonary artery. All sequences were completed over a total time of about 11 minutes. LV and RV volumes were computed by Simpson's rule. PVM volumes were computed using system software. RHC was performed directly after the CMR. Qp/Qs ratios were calculated from O2 saturation measurements.
Results: Results show an excellent correlation between SV and PVM (r=0.90; p<0.0001) and a good correlation between Qp/Qs by either of the CMR techniques compared to RHC (SV vs RHC: r= 0.55; p=0.027, PVM vs RHC: r=0.62; p=0.0096). 15 of the 16 pts (94%) were correctly characterized as having shunts >1.5 or <1.5.
Conclusion: Either of the CMR techniques can reliably characterize patients with significant inter-atrial shunts. The combination of SV, PVM, and imaging of the defect itself provides a robust method for non-invasively evaluating the patient with an ASD.
145. Quantitative Assessment of Atrial Septal Defect Closure Using a Hybrid XMR System
Simon Schalla, MD,1 Maythem Saeed, PhD,1 Alastair Martin, PhD,2 Oliver Weber, PhD,1 Charles B. Higgins, MD,1 Phillip Moore, MD.3
Radiology, University of California San Francisco, San Francisco, CA, USA, Philips Medical Systems, San Francisco, CA, USA, Pediatric Cardiology, University of California San Francisco, San Francisco, CA, USA.
Introduction: Defects of the atrial septum are the most common congenital heart disease. They are associated with stroke, heart failure and pulmonary hypertension. Therefore, these defects often require closure to prevent the formation of emboli or heart failure. X-ray fluoroscopy guided catheter based closure devices have been used to avoid open heart surgery. The recent development of hybrid XMR systems (a suite adjoining MRI scanner with x-ray catheterization laboratory) allow the placement of septal defect closure devices under MR guidance. This MR technique minimizes the radiation exposure of patients and staff and provides real-time images. Furthermore, it is useful for evaluating the accomplishment of interventional procedures. At the present time, however, commercially available delivery systems and closure devices cause large susceptibility artefacts on MRI.
Purpose: Aims of this study were to 1) built MR compatible delivery systems using commercially available materials, 2) use the unique capabilities of XMR system in the deployment and assessment of septal defect closure. Velocity encoded cine and contrast enhanced fast MRI were used for assessment of volumetric blood flow through the defect and to demonstrate the leakage of contrast media through the septal defect, respectively.
Methods: A septal defect was created in 7 pigs (20–40 kg) with a needle catheter and subsequent balloon dilatation under x-ray fluoroscopy. The balloon was inflated with the iodinated contrast Omnipaque in the x-ray unit and with 5mM Gd-DTPA-BMA in the MRI unit prior to delivery of the closure. Catheters were placed in the main pulmonary and iliac arteries to monitor central and peripheral blood pressures during and after the intervention. The animals were then transferred to the MR unit on a tabletop (1.5 T Philips Intera scanner, Philips Medical Systems, Best, The Netherlands). Pulmonary and aortic flow measurements were obtained using retrospectively gated phase contrast gradient echo sequence. The imaging parameters were TR=15 ms, TE=4 ms, flip angle=20°, scan matrix 192×192, slice thickness 8 mm, FOV 200 mm). A T1-TFE imaging sequence was used for the first pass of Gd-DTPA-BMA (20 ml of 20% of stock solution) through the central circulatory system. The imaging parameters were TR=3.8 ms, TE=1.4 ms, flip angle=30°, scan matrix=192×192, slice thickness 8 mm and FOV=20 cm. After these baseline measurements, a catheter based closure device was used for defect closure under MR guidance. A real-time bFFE acquisition was used with TR=3 ms, TE=1.5 ms, flip angle=60°, slice thickness=10 mm, FOV=200 mm, scan matrix=128×128, and 10 frames/s).
The delivery device consisted of a sheath, dilator, snare wire and Amplatzer closure device. All materials were commercially available, but home-assembled. The closure device was mounted on the tip of a dilator and retracted by a snare wire. Following the positioning of the sheath, the closure device was advanced through it across the defect and slowly released. After defect closure, all measurements performed at baseline were repeated. Central and peripheral pressures as well as heart rate and oxygen saturation were measured using saline filled pressure transducer and oxymeter. At postmortem the shunt size was measured and the device position examined.
Results: Deployment of the closure device: The interventional procedure of creating the septal defect was performed under x-ray fluoroscopy. The septal hole was dilated with a balloon catheter to a diameter of 2.4cm (Fig. ). The animal was then transferred to the MRI unit on a tabletop in less than a minute without changing the position of the animal. The home-assembled catheter system was free of susceptibility artefacts on MRI. Figure shows the sheath through the septum and the process of unfolding of the first and second disk of the closure device under MRI guidance.
Hemodynamic measurements: The central pulmonary and peripheral arterial pressures and heart rate were determined and related to the amount of left-to-right shunt flow. Mean pulmonary arterial pressure was 33±3 mmHg. Systolic, diastolic and mean arterial pressures were 137±12, 108±8 and 94±8mmHg, respectively. Heart rate min and oxygen saturation were 111±6 beat/min and 97±2%.
Flow measurements: Under the above physiology condition, mean blood flow through the main pulmonary artery was 4.23±0.33 L/min after creation of the defect and 3.69±0.68 L/min after closure of the defect. While the aortic flow was 3.44±0.49 L/min after creation of the defect and 3.85±0.58 L/min after defect closure. After creation of the defect, aortic flow was substantially smaller than that in main pulmonary flow. The difference between the flow in both vessels represents the amount of left-to-right shunt flow (0.73±0.33 L/min). This difference subsided after placement of the Amplatzer closure. Phasic flow (16 phases/cardiac cycle) showed no difference in pattern before and after closure deployment, suggesting that the heart rapidly accommodate to changes in preload and afterload.
First pass Gd-DTPA-BMA in the central circulation. Bolus administration of Gd-DTPA-BMA (20ml of 20% stock solution) in the main pulmonary artery showed immediate enhancement of left atrium and right atrium prior to the closure of the defect. After placement of the closure device we found small leakage of the contrast medium through the shunt.
Conclusions: The placement of septal defect closure devices under MR guidance is feasible using commercially available catheters, wires and closure devices. Furthermore, MR imaging has the benefit of quantitative assessment of the success of interventions. Visualisation of the defect site on contrast enhanced MRI and measurement of the amount of flow through the shunt are dependent on several physiological and pathological factors, such as pressure afterload, cardiac compliance and the size of the defect.
146. A Comparative Study of Left Ventricular Mass Between Cardiac MR and 2-D Echo in Hypertensive Subjects
Khaled Alfakih, MBBS,1 Tim Bloomer, MBBS BSc,1 Sam Sleight, MSc,2 Gavin Bainbridge, DCR,1 John Ridgway, PhD,3 Gordon Williams, MD,2 Mohan Sivananthan, MD.1
Cardiac MRI Unit, Leeds General Infirmary, Leeds, United Kingdom, Dept. of Echocardiography, Leeds General Infirmary, Leeds, United Kingdom, Dept. of Medical Physics, Leeds General Infirmary, Leeds, United Kingdom.
Introduction: Elevated left ventricular mass (LVM) is a predictor of cardiovascular morbidity and mortality in hypertension. 2-D echocardiography is used to estimate LVM.
Purpose: To compare LVM as measured by Cardiac MRI (CMR) with 2-D echo in Fundamental and Harmonic imaging modalities.
Methods: Thirty two hypertensive patients were recruited from cardiology out-patients departments with a mean 24hr BP of 140/90 or higher. Echo LVM was calculated using both Harmonic and Fundamental imaging methods. A parasternal short axis view at the level of the papillary muscle tips and the apical four or two chamber view (whichever maximizes left ventricular length) were obtained and applied to: Truncated Ellipse (TE) and Area Length (AL) formulae. One experienced cardiac technician performed the echocardiogram once on each patient and analysed the video images twice for each method on different days taking the mean measurement. CMR was performed on a Philips 1.5T ACS NT MRI scanner with a cardiac phased array surface coil. Standard short axis views were acquired using Turbo Gradient Echo with the following parameters TR 8.0, TE 4.9, Flip angle 35, 11–13 Phases. Volumetric analysis was performed using MASS software (MEDIS, Leiden). The LVM was calculated using diastolic slices.
Results: Seven subjects were excluded due to inadequate echo views. Twenty five patients had both echo and CMR. The four different echo means were compared with the CMR mean using Bland Altman analysis (Table ). The intra-observer variability for CMR expressed as bias±SD was 2.3±9.2 g and the inter-observer variability 3.8±10.4 g. The Echocardiography intra-observer variability for the four different measurements were; Fundamental TE 0.4±26.8 g, Fundamental AL-0.6±27.0 g, Harmonic TE 6.7±21.8 g, Harmonic AL 6.4±22.9 g.
Conclusions: The observer variability for LV mass as measured by cardiac MRI is much smaller than that for any of the four echocardiography methods used. The use of Harmonic imaging did not make any significant difference. This may be because the endocardial and epicardial borders are less well defined on echo than MRI, but also the 2-D echo LVM measurement relies on geometric formulae that assume the Left ventricle to be a truncated ellipsoid or prolate ellipsoid. This is not the case in CMR as the LVM is calculated through spatially defined three-dimensional datasets.
Comparing the AL methods with CMR, the bias (the mean difference) is very small between the two methods irrespective of the imaging modality used. Comparing the TE methods there is a bigger and negative bias, implying that the TE method underestimates LVM irrespective of the imaging modality.
Table 1. MR vs. echo measurments
147. Assessment of Fiber Architecture and Remodeling Process in Myocardial Infarction by Using Diffusion Tensor MR
Ming-Ting Wu, M.D.,1 Wen-Yi I. Tseng, M.D., Ph.D,2 Mao-Yuan Su,3 Van Wedeen, M.D.,4 Timothy Reese, Ph.D.,4 Kuan-Rou Chiou, M.D.,5 Chun-Peng Liu, M.D.5
Department of Radiology, Kaohsiung Veterans General Hospital, Kaohsiung, Taiwan Republic of China, Medical College, Center for Optoelectronic Biomedicine, National Taiwan University, Taiwan Republic of China, National Yang-Ming University, Institute of Medical Engineer, Kaohsiung, Taiwan Republic of China, NMR Center, Massachusetts General Hospital, Charlestown, MA, USA, Division of Cardiology, Department of Medicine, Kaohsiung Veterans General Hospital, Kaohsiung, Taiwan Republic of China.
Introduction: The left ventricle undergoes marked architectural changes in response to myocardial infarction. The remodeling process, while remains not completely understood, involves ventricular dilatation, distortion, mural hypertrophy and functional alternation. The interplay between function and structure change is critical in the biomechanics of ventricular remodeling, previous morphological studies were limited to the wall thickness and myocardial tagging. The ventricular fiber architecture, however, has been difficult to characterize. We have reported a cardiac MRI using diffusion tensor image to map the fiber orientation of the beating human heart. It is now possible to employ this method to investigate the internal structure change in the myocardium post infarction.
Purpose: We sought to characterize the fiber architecture disarray in the myocardium post infarction.
Methods: Nine subjects (37–71 y/o, median 59) with acute MI was enrolled. Initial MRI performed within 10 days of the episode. Follow-up MRI was performed 6 months later. MRI were all performed on GE CVi 1.5 T scanner. Imaging protocol included: (1) cine gradient echo in short axis for wall motion evaluation. (2) double-gated stimulated echo of diffusion weighted echo planar images. The diffusion gradient was applied in 6 directions and each direction required two heart beats. Patients were asked to keep constant position of end tidal expiration and last for 14 heart beats for one complete set of diffusion tensor images. It usually repeated 6–12 averages to improve image quality. The slices were chosed constantly at three levels; i.e., apex, mid ventricle and base. (3). myocardial contrast delay enhancement for viability map. The sequence was normal myocardium-nulled and enhanced the delay wash-out effect in the infarted area. The viability map served as a reference of segmentation of infarct zone, border zone and remote zone. The trace apparent diffusion coefficiency (ADC),fractional anisotrophy (FA), and histogram of fiber helix angle of each zone were computed and compared (Fig. ).
Results: The infarct zone showed higher trace ADC (p=0.001)and lower FA (p=0.002) as comapred to the remote zone. There was zonal effect of fiber helix angle distribution. The inner-wall fiber showed significant decrase in the infarct zone, as compared to border zone (p=0l01) or remote zone (p=0.01). The diference progressed in the follow-up MRI (p<0.001 for both). The outerer-wall fiber showed significant increase in the infarct zone, as compared to other zones (p<0.001), however, the difference was only observed in the follow-up MRI. As for the time effect, in the infarct zone, the inner-wall fiber showed gradually decreased (p=0.04) and the out-wall fiber incresed (p=0.04). In contrary, the remote zone showed inner-wall fiber increased (p=0.04)and out-wall fiber decreased (p=0.03).
Conclusions: Increase of ADC and decrease of FA characterized the cellular breakdown and loss of tissue archietecture in the infarct zone. Redistribution of helix angles may mediate the adaptation of ventricular structure and kinematics which shed light on the mechanism of ventricular remodeling post infarction.
148. Exercise Cardiac Stress Testing Using Real-Time MRI: A Comparison with Stress Echo in Patients
James F. London, Gauri Tilak, Srirama V. Swaminathan, John Whang.
The Heart and Vascular Institute of Florida, Safety Harbor, FL, USA.
Introduction: Cardiac magnetic resonance imaging (MRI) is emerging as an important imaging modality for pharmacological stress testing. Recent developments in real-time MR imaging techniques may make performing high-quality real-time stress imaging with adequate temporal and spatial resolution possible.
Purpose: The aims of this study was to compare the image quality of this technique to that of the standard technique used for the assessment of wall motion with exercise stress testing, stress echocardiography.
Methods: The study population consisted of 30 consecutive patients (16 men and 14 women; age 55±10.6) referred for exercise stress echocardiography. Real-time MRI of the heart was performed using balanced fast field echo and SENSE on a 1.5 Tesla scanner (Philips Intera CV, Nederland, B.V.). Eight short-axis slices were acquired during each scan. Scan parameters included. flip angle=55 degrees, matrix=128×64, field of view=53×40 cm, fat/water shift=0.139 pixels, TR=2.4 ms, TE=1.2 mm, fractional NEX acquisition=0.5, slice thickness=8 mm, and an inter-slice gap=4.6 mm, SENSE factor=2. We achieved an imaging rate of 25 frames per second.
Exercise was achieved using a MRI compatible ergometer (Lode, Groningen, Netherlands). The subjects performed exercise in the supine position on the examination table. The workload was increased by 20 watts every 2 minutes until a fatigue-limited maximum was achieved. During exercise, heart rate, rhythm, and blood pressure were monitored using an MRI compatible patient monitoring system (MRE, Bay Shore, NY). Rate pressure products at both rest and stress were calculated for each subject. Subjects also underwent stress echocardiography with harmonic imaging. Two blinded readers independently evaluated wall motion and image quality using the 16 segment model.
Results: All 30 patients were able to complete the exercise MRI study without complication. Three subjects refused exercise stress echocardiography. Using the MRI ergometer subjects exercised for 7.5±1.7 minutes achieving a workload of 105±23 Watts. The rate pressure product was higher with exercise MRI than with stress echo 25,201±6,483 vs 23,435±5,628 (p<.05). Both exercise and imaging were well tolerated by all subjects. There were no arrhythmias with exercise. The ejection fraction as measured by MRI was 58.9% at rest and increased on average 12.3±6.9% with stress (p<.05). No patients showed evidence of exercise induced ischemia with either technique. At rest all segments could be adequately visualized with both MRI and echo. At stress more segments could be adequately visualized with MRI than echo 100% vs 90.7% (p<.05).
Conclusions: Real-time exercise MRI allowed the quantitative assessment of global LV systolic function during a high level of physiologic stress and showed significant increases compared to resting values. Stress MRI image quality was superior to stress echo image quality.
149. Gender Differences in Myocardial Contraction Fraction, a Volumetric Measure of Myocardial Performance
Michael L. Chuang,1 Carol J. Salton,1 Christopher J. O'Donnell,2 Kraig V. Kissinger,1 Daniel Levy,2 Warren J. Manning.1
Cardiovascular Medicine, Beth Israel Deaconess Medical Center, Boston, MA, USA, The NHLBI's Framingham Heart Study, Framingham, MA, USA.
Introduction: Patients with hypertrophied ventricles may have preserved ejection fraction (EF) in the presence of heart failure symptoms. The linear measure of mid-wall shortening fraction (MWSF) has been used to identify groups with depressed myocardial function despite apparently normal EF. Recently, the ratio of left venticular (LV) stroke volume (SV) to myocardial volume (MV), or “myocardial contraction fraction” (MCF=SV/MV), has been proposed as a volumetric extension of the MWSF and has been used to differentiate between hypertensive and physiologic (athletic) LV hypertrophy [DL King, et al., JACC 2002; 40:325–329]. The MCF may be more sensitive than the MWSF, as the former summarizes both radial and base-to-apex contraction, while the latter primarily reflects radial shortening. Age, sex and obesity are known to affect myocardial volume, but the potential effects of these characteristics on the MCF is not known.
Purpose: We sought to determine the influence of age, sex and body habitus on the MCF in a large, longitudinally followed population-based sample of adults without clinical evidence of cardiac disease.
Methods: Three-hundred eighteen adult members of the Framingham Heart Study (FHS) offspring cohort, all free of clinical cardiac disease by history and physical examination, were scanned using contiguous multislice breathhold cine MRI. Imaging parameters included TR=R-R interval, TE=9 ms, FA=30°, 1.25×2.0×10.0 mm3 voxels. The SV and MV could be calculated, using a summation of disks method, in 304 subjects (155 women, 149 men, respectively aged 60.4±9.0 and 59.3±9.0 years, p=NS); for whom the MCF also was calculated. In addition to sex, subjects were further partitioned by decade age (≤50, 51–60, 61–70 and >70 years) or body mass index (BMI, lean: 19≤BMI<25, overweight. 25≤BMI<30, obese: BMI≥30 kg/m2). Continuous data are summarized as mean±SD. Pearson correlation was used to summarize relationships between MCF and age or BMI. Simple t tests were used to compare the MCF between sexes and between subgroups. A p value<0.05 was considered statistically significant.
Results: Overall, the MCF was greater in women than men in the study population (W: 0.58±0.13; M: 0.51±0.11, p<0.0001). Greater MCF in women persisted even when only normotensive subjects (presentation systolic blood pressure <140 mmHg) were considered, with MCF=0.59±0.12 for normotensive women and 0.53±0.12 for normotensive men, p<0.0001. There was a weak inverse correlation between age and the MCF in women (r=−0.16, p=0.05) but not for men (Fig. ). There was no relationship between the MCF and BMI for men or women.
Conclusions: The MCF is a volumetric measure of cardiac performance that relates LV stroke volume to myocardial volume. The present study suggests that middle-aged and older adult women have a greater MCF than similarly aged men, so that for a given volume of myocardium, the woman's heart ejects a proportionally greater volume of blood. MCF may decline with increasing age in women, but does not appear to do so in men. Whether this sex-differential effect holds true in children or younger adults remains to be studied. Further, the effects of physical training and their interaction with various forms of cardiovascular disease on the MCF are unknown. Finally, the CMR-based values for MCF are greater than those determined by King et al for normal sedentary subjects using three-dimensional echocardiography, and additional study is necessary to reconcile the apparent differences between these two volumetric imaging methods.
150. Safety and Feasibility of High-Dose Dobutamine-Atropine Stress MRI for Diagnosis of Myocardial Ischemia: Experience in 1000 Consecutive Cases
Andreas Wahl, MD,1 Albrecht Gollesch,2 Ingo Paetsch, MD,2 Stefan Roethemeyer, MD,3 Daniela Foell, MD,4 Holger Langreck, MD,2 Rolf Gebker, MD,2 Kristof Graf, MD,2 Christoph Klein, MD,2 Eckart Fleck, MD,2 Eike Nagel, MD.2
Cardiology, Swiss Cardiovascular Center Bern, Bern, Switzerland, Cardiology, German Heart Institute Berlin, Berlin, Germany, Cardiology, Heart Center Osnabrueck-Bad Rothenfelde, Bad-Rothenfelde, Germany, Cardiology, University Hospital of Freiburg, Freiburg, Germany.
Introduction: High-dose dobutamine-atropine stress MRI (DSMR) recently emerged as a highly accurate diagnostic modality. However, no large scale clinical experience has been reported so far.
Purpose: To determine the safety, feasibility and adverse effects of DSMR in a large unselected patient population.
Methods: From July 1997 to June 2002, 1079 consecutive DSMR examinations were attempted in 1039 consecutive patients. Images were acquired at rest and during a standardized high-dose dobutamine-atropine protocol in 3 short-axis, a 4- and a 2-chamber view. A single-slice segmented gradient echo (TR/TE/flip 5.6/1.9/25), and from 2001 on, a steady state free precession technique (TR/TE/flip 3.0/1.5/55) were used. Dobutamine was infused at doses of 10, 20, 30, and 40 μg kg−1 min−1, and supplemented by atropine if needed, until ≥85% of age-predicted heart rate was reached. Stress testing was discontinued when ≥85% of age-predicted heart rate was reached, on patient request, protocol termination, or when new or worsening wall motion abnormalities, severe angina, dyspnea, severe increase or decrease in blood pressure, or severe arrhythmias occurred.
Results: In 79 cases (7.3%), MR-imaging could not be performed due to claustrophobia (n=64; 5.9%), severe obesity (n=11; 1%) or non MR-compatible metallic implants (n=4; 0.4%). In the remaining 1000 cases in 960 patients (60±10 years; prior myocardial infarction 24%; prior percutaneous or surgical revascularization in 40% and 18%, respectively) with suspected (n=495; 49.5%) or known (n=505; 50.5%) coronary artery disease, DSMR was successfully performed in all but 4 cases (0.4%; insufficient ECG-triggering). In the absence of ischemia, target heart rate was not reached in 95 cases (9.5%), due to end of protocol in submaximal negative examinations in 21 cases (2.1%), and limiting side effects in 74 cases (7.4%), including severe chest pain (n=30; 3%), dyspnea (n=10; 1%), nausea (n=4; 0.4%), urinary urgency (n=1; 0.1%), severe increase (n=5; 0.5%) or decrease (n=3; 0.3%) in blood pressure, ventricular extrasystoly (n=8; 0.8%), paroxysmal atrial fibrillation (n=5; 0.5%), and patient request (n=8; 0.8%). Total number of side effects included one case (0.1%) of sustained and 4 cases (0.4%) of non-sustained ventricular tachycardia, 16 cases (1.6%) of paroxysmal atrial fibrillation, 2 cases (0.2%) of transient second degree AV block and 31 cases (3.1%) of nausea. Image quality was non diagnostic in 6 cases (0.6%), due to breathing or motion artifacts. Four hundred twenty patients (42%) showed a new or worsening wall motion abnormality, while 475 (47.5%) did not.
Conclusions: High-dose DSMR is safe and feasible in patients with suspected or known coronary artery disease. However, patients must be closely monitored, and resuscitation equipment and trained personnel must be available.
151. An Mr Derived Aorto-ventricular Coupling Index Is More Predictive Of Cardiac Death Than Ef: An Nhlbi Sponsored Women's Ischemia Syndrome Evaluation (Wise) Study
Mark Doyle, Ph.D.,1 Diane A. Vido, MS,1 Gerald M. Pohost, MD,2 Sheryl F. Kelsey, Ph.D.,3 Delia B. Johnson, Ph.D.,3 William J. Rogers, MD,4 Steve Reis, MD,5 Marian Olson, MS,6 Leslee Shaw, Ph.D.,7 Barry Sharaf, MD,8 Carl Pepine, MD,9 Noel C. Bairey Merz, MD,10 Sunil Mankad, MD,1 Robert W. W. Biederman, MD.1
Med, AGH, Pittsburgh, PA, USA, Med, USC, Los Angeles, CA, USA, Graduate School of Public Health, Epidemiology Data Center, Pittsburgh, PA, USA, Med, UAB, Birmingham, AL, USA, Med, UPMC, Pittsburgh, PA, USA, UPMC, Pittsburgh, PA, USA, ACRI, Atlanta, GA, USA, Cardiology, Rhode Island Hospital, Providence, RI, USA, Med, University of Florida, Gainesville, FL, USA, Med, Cedars-Sinai Research Institute, Los Angeles, CA, USA.
Introduction: The prognostic value of ejection fraction (EF) in cardiovascular disease is well-established. However, physiologic adaptations that serve to sustain EF level may make it a late indicator of disease and thus a relatively late predictor of adverse events. We investigated a ventricular energy model that formulated a ventricular–aortic coupling index that can be derived from the data obtained in a standard MRI evaluation of the left ventricle.1
Purpose: To test whether a noninvasively derived MRI index of ventricular performance has higher clinical prognostic value than the widely used ejection fraction in patients with a suspicion of coronary artery disease.
Methods: Two hundred and one women undergoing myocardial perfusion for chest pain evaluation as part of the NHLBI-Sponsored Women's Ischemia Syndrome Evaluation (WISE) Study and 21 control women with low risk of cardiovascular disease were included in this analysis. All patients underwent functional MRI examination of the left ventricle and blood pressure measured by arm cuff plethysmography. Following the example established in echocardiography, an index of ventricular-aortic coupling was calculated from the MR derived measurements of end-diastolic volume, end-systolic volume and systolic blood pressure.2 The ventricular-aortic coupling index was calculated as the slope of the line connecting the end-systolic to end-diastolic points of the pressure–volume loop. End-systolic (EDV) and end-diastolic (EDV) volumes were calculated by planimetering the endocardial border in multiple short axis images through the left ventricle. In the absence of the availability of ventricular pressures, end-systolic pressure was taken as the systolic pressure (SBP) measured by arm-cuff and end-diastolic pressure was taken as being proportional to the end-diastolic volume, with the constant of proportionality taken as 0.005.3 The formula for the ventricular-aortic coupling index is:(SBP-EDV×0.005)/(EDVi-ESVi)
Where EDVi and ESVi are the end-diastolic and end-sytolic volumes normalized by body mass index. The EF was calculated using the same ventricular parameters. In addition to MRI functional analysis, data were available from radionuclide SPECT to detect the presence of ischemia or scar, and from coronary catheterization to measure the largest stenosis in any major coronary artery. A cardiologist at a core facility, using computerized quantitative methodologies, interpreted the coronary angiograms. Follow-up was performed to monitor cardiovascular death. A multivariate Cox regression model was performed to determine which diagnostic indicators were independently predictive of the endpoint of death. Diagnostic and physiologic variables entered into the model included: EF, the ventricular-aortic coupling index, presence of ischemia or scar noted by SPECT, the highest recorded stenosis value by quantitative coronary angiography and age in addition to the indexed variables of EDVi, ESVi and SBP. Significance criteria were set at 0.05 for inclusion in the model, and 0.10 for removal from the model. Statistical analyses were performed using SPSS version 9.0.
Results: When comparing patients to controls, ejection fraction did not differ significantly between groups (62.2±11 vs. 60.0±5, p=NS) while the ventricular-aortic coupling index was significantly higher for patients (2.38±0.9 vs. 1.56±0.2, p<0.001), Fig. . During the mean follow-up duration of 28±7 months, twelve (5.9%) patients died. A multivariate analysis using Cox stepwise regression revealed that ventricular-aortic coupling index was the strongest prognostic predictor of death (Chi-square=29.2, p<0.001). When only EF was entered into the model it predicted cardiac death with a substantially lower goodness of fit (Chi-square=12.2, p<0.05). If the ventricular-aortic coupling index was removed from the model, the largest noted coronary artery stenosis became the best predictor, also with a significantly lower goodness of fit (Chi-square=9.2, p<0.001), Fig. . We note with interest that for patients in whom the ventricular-aortic coupling index was known, 12 of 12 deaths occurred in patients within the highest quartile of the ventricular-aortic coupling index. This compared favorably to EF in which only 8 of 12 deaths occurred in patients within the lowest quartile of EF. Indeed, a threshold of a vascular coupling index of >2.8 was 100% accurate in predicting all of the observed deaths at the 2 year follow-up.
Conclusions: Although EF remains widely used as a prognostic indicator of patients with a suspicion of coronary artery disease and as the threshold for administering therapy, it is widely acknowledged that it is an imperfect index of ventricular performance. In this study, EF was not different between patients and controls. The use of EF has been defended on grounds that it is intuitive and relatively easy to measure. The non-invasive ventricular-aortic coupling index is equally easy to calculate and incorporates the cuff derived blood pressure measurement. Importantly, we demonstrate here that the MR derived ventricular-aortic coupling index has higher prognostic value than EF and is a very strong prognostic indicator of mortality in women undergoing evaluation of symptoms of ischemia. This MRI derived parameter may unmask disease at an earlier stage than the traditional EF parameter and may become the new standard for prognostication and stratification.
Figure 1. Plot of percent difference in physiologic variables between patients and contrzols. Significant change is exhibited in the MRI derived EDVi, and ESVi, the systolic blood pressure (SBP) and the ventricular-aortic coupling index (VACi). Note that EF is not significantly different between groups.
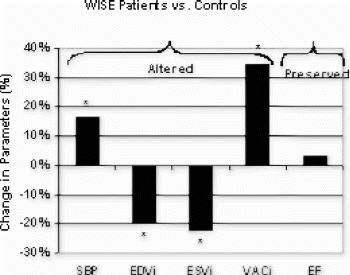
References
1. R.M. Shoucri. “Pressure-volume relation in left ventricle,” Am J Physiol vol.260, ppH282–H291, 1991.
2. Saba PS, Ganau A, Devereux RB, Pini R, Pickering TG, Roman MJ, Impact of arterial elastance as a measure of vascular load on left ventricular geometry in hypertension, J Hypertens 1999 Jul;17(7):1007–1015.
3. Carabello, BA, Abnormalities in Cardiac Contraction. Systolic Dysfunction, Congestive Heart Failure, Eds Hosenpud, JD, Greenberg, BH, Springer-Verlag, New York, 1994, pp54–67.
152. Dynamic 3D MR Angiography for the Assessment of Parenchymal Perfusion in Pulmonary Embolism and Pulmonary Circulation Disorders
Alexander Kluge, MD,1 Clemens Mueller, PhD,1 Jochen Hansel, MD,2 Thorsten Dill, MD,2 Georg Bachmann, MD.1
Diagnostic Radiology, Kerckhoff-Clinic, Bad Nauheim, Germany, Cardiology, Kerckhoff-Clinic, Bad Nauheim, Germany.
Introduction: Conventional MRA for the detection of pulmonary embolism offers a resolution adequate for the depiction of segmental, subsegmental and subsubsegemental pulmonary arterial vessels. Pulmonary embolism at the level of subsegmental pulmonary arteries, however, cannot be excluded with sufficient certainty.
Purpose: Contrast enhanced dynamic 3d angiography with a spatial resolution low enough to average the pulmonary artery vessel signal allows the assessment of parenchymal perfusion. We tested its value for the diagnosis of pulmonary embolism.
Methods: We studied the pulmonary perfusion in 15 patients referred for suspected pulmonary embolism with a 1.5T MR scanner (Sonata, Siemens). We used contrast enhanced high-resolution MR angiography (CE-HR-MRA), single shot true FISP-sequences and dynamic 3d flash contrast enhanced MRA with low spatial resolution and high temporal resolution. The sequence used allowed the coverage of the entire lung with 14 slabs and a voxel size of 1.5×1.5×8–10 mm in 2.2 sec. Two separate injections of 0.1 mmol/kg Gd-DTPA for HR-CE-MRA and 0,77 mmol/kg for dynamic MRA were used at 4 ml/sec.
Results: Compared to true FISP sequences and HR-MRA, dynamic MRA depicted 17 of 24 segments with embolic material. Recanalized embolism and older embolic material was not detected. Seven small subsegmental perfusion defects were detected with dynamic MRA only. Two cases with pulmonary arterial perfusion disorders caused by pulmonary vein stenosis after ablation of atrial fibrillation were obvious in dynamic MRA and difficult to detect in HR-MRA (Fig. ).
Conclusions: Dynamic low resolution MRA covering the entire lung is an additional tool for the assessment of pulmonary embolism in MRI and detects smaller subsegmental perfusion defects than conventional MRA. Perfusion changes due to pulmonary vein stenosis after ablation of atrial fibrillation could be detected in MRI with dynamic MRA for the first time. If used in a combination with high resolution CE-MRA and a robust method for emergency diagnostic, e.g. true FISP single shot sequences, all stages of pulmonary embolism can be diagnosed in MRI.
153. Real-Time MRI-Guided Endograft Delivery in a Porcine Model of Abdominal Aortic Aneurysm
Venkatesh K. Raman, MD, Michael A. Guttman, MA, Breno S. Pessanha, MD, Alexander J. Dick, MD, Dana C. Peters, PhD, Elliot R. McVeigh, PhD, Robert J. Lederman, MD.
National Heart Lung & Blood Institute, National Institutes of Health, Bethesda, MD, USA.
Introduction: Catheter-based endovascular repair is emerging as an alternative to surgery for management of abdominal aortic aneurysm. Such procedures are currently performed under x-ray fluoroscopy. Thus far, endografting has been limited by the frequent occurrence of endoleaks due to graft malapposition, aneurysm anatomy and branch vessels. Real-time MRI (rtMRI) may improve the safety and effectiveness of endovascular techniques by providing tissue context and three-dimensional anatomy to guide procedures.
Purpose: We test the feasibility of endograft delivery under real-time MRI guidance in a porcine model of abdominal aortic aneurysm.
Methods/Results: rtMRI was performed using a clinical 1.5T GE cardiac scanner customized with a fast external reconstruction engine, in-room consoles, and interactive features such as magnetization preparation and interleaved multiplanar acquisition for the interventional procedure. Imaging with steady-state free precession (SSFP) used the following typical parameters. 256×128 matrix, partial phase Fourier, flip angle 60, FOV 36 cm, and bandwidth ±62.5 kHz. 50–70 kg swine were subjected to 2-fold balloon overstretch to create a dilated segment appearing as an abdominal aortic aneurysm. Clinical tubular stent-graft device (Vanguard, Boston Scientific) positioning and delivery was facilitated using an intravascular 0.030″ MRI guidewire receiver coil (Intercept, Surgi-Vision). Post-deployment MR angiography confirmed exclusion of the aneurysmal segment.
Conclusions: rtMRI successfully guided endograft delivery in an experimental model and may be promising for the clinical treatment of abdominal aortic aneurysm. Customization of endografts and delivery systems can be expected to facilitate accurate device placement in patients with complex aneurysm disease.
154. Picomolar Concentrations of Targeted UltraParamagnetic Nanoparticles Detect Microscopic Pathology
Anne M. Morawski,1 Patrick M. Winter,1 Shelton D. Caruthers,2 Ralph W. Fuhrhop,1 Samuel A. Wickline,1 Gregory M. Lanza.1
Cardiovascular MR Laboratories, Washington University School of Medicine, St. Louis, MO, USA,Philips Medical Systems, Best, Netherlands.
Introduction: Basic research into the genetic, biochemical and physiological mechanisms of disease processes have lead to the discovery of unique molecular signatures for many pathologies. The emerging field of “molecular imaging” is largely focused on the sensitive and specific detection and visualization of these unique epitopes for the early detection of disease. In the field of MRI, practical application of molecular imaging is often limited by the signal amplification available from paramagnetic agents when imaging sparse concentrations of epitopes in nanomolar or picomolar quantities.
We have developed a molecular imaging contrast agent platform consisting of liquid perfluorocarbon nanoparticles coupled to targeting ligands and paramagnetic chelates. These nanoparticles exhibit extremely high relaxivity at clinical imaging field strengths and have been used to detect fibrin associated with vulnerable atherosclerotic plaques (1), tissue factor related to vascular injury (2), angiogenesis (3), and even single monolayers of smooth muscle cells in culture (5).
To define the minimum concentration of nanoparticles required to differentiate the targeted pathology from normal tissue, we developed a mathematical model to simulate MR signals acquired with typical pulse sequences. We predicted and then experimentally verified the number of nanoparticles required for adequate signal enhancement using phantoms at 1.5T. The results from the model and phantom imaging indicate that picomolar concentrations of our paramagnetic nanoparticles can provide sufficient contrast-to-noise (CNR) to detect microscopic pathology.
Methods: Nanoparticle Preparation: Liquid perfluorocarbon nanoparticles (nominal diameter of 250 nm) were produced by incorporating a paramagnetic chelate (Gd-DTPA-BOA) into the outer lipid membrane. The final Gd3+ and nanoparticle concentrations were 4.7 mmol and 55 nmol per liter of emulsion, respectively. The longitudinal and transverse relaxivities (r1 and r2) of the nanoparticles at 1.5T are 17.7 and 25.3 (s mM)−1, respectively, in terms of [Gd3+]. For a targeted contrast agent, however, relaxivity per nanoparticle offers a more instructive measurement of the paramagnetic payload delivered to each epitope. In this case, r1 and r2 are 1.51×106 and 2.16×106 (s mM)−1, respectively, in terms of [nanoparticle]. At 4.7T, r1 and r2 are 9.7 and 29.4 (s mM)−1, respectively, in terms of [Gd3+], and 8.28×105 and 2.51×106 (s mM)−1, respectively, in terms of [nanoparticle].
Model Development: MatLab version 6.1 was used to model spin echo MRI signals. The T1 relaxation time in the presence of nanoparticles was calculated based on the concentration of gadolinium and the nanoparticle relativity at 1.5T (4). The spin-echo signal intensity is related to the tissue T1 and T2 as well as the TR and TE of the pulse sequence (4). For two tissues with different T1s, the CNR between the two is maximized at the optimum TR (4). The model uses these equations to quantify the amount of nanoparticles necessary to produce adequate contrast between the targeted tissue (containing nanoparticles) and the native tissue (without nanoparticles). Identical calculations were performed with the relaxivity values and typical relaxation times at 3T and 4.7T.
Phantom Imaging: Phantoms consisted of 40% glycerol in water, mimicking the T1 and T2 of blood (∼1200 and 50 ms, respectively). Separate phantoms with varying concentrations of paramagnetic nanoparticles (Sample 1: 0 μM Gd3+, Sample 2: 8.3 μM Gd3+ and Sample 3: 25.8 μM Gd3+) were imaged simultaneously using a clinical 1.5T magnet (Gyroscan NT, Powertrak 6000, Philips Medical Systems, Best, Netherlands) and a quadrature birdcage head coil. T1 measurements of each sample were obtained using a Look-Locker sequence and 2D spin echo images (TE=15 ms, NSA=2, 2 mm slice thickness, 0.55 mm by 0.55 mm in-plane resolution) were collected over a range of TRs (including the theoretical optimum). The signal intensity of each phantom and the noise were measured at each value of TR. The CNRs of each phantom were calculated relative to the phantom without nanoparticles, and then plotted.
Results: Table summarizes the numerical results of the phantom experiments at 1.5T. Given the [Gd3+] and the nanoparticle relaxivity, the model outputs CNR between a control phantom (#1. no Gd3+) and phantoms 2 and 3 for optimal TR. Figure illustrates the close correspondance between predictions and measured phantom data for CNR. The phantom experiments confirm the model predictions at 1.5T, with CNR reaching the theoretical maximum at the optimum TR.
The model predicts that under conditions of optimal TR at 1.5T, a nanoparticle concentration of 79 pM will provide sufficient contrast enhancement to allow differentiation between two tissues (CNR=5). It also indicates that imaging ∼400 ms away from the optimum TR can result in ∼30% loss in CNR (Fig. ). The model predicts that even lower nanoparticle concentrations will produce CNR=5 at higher field strengths. 31 pM at 4.7T.
Conclusion. These experiments demonstrate that even at very low concentrations of ultra-paramagnetic nanoparticles (∼100 pM), adequate contrast can be obtained with spin-echo MRI at the optimum value of TR using a clinical scanner. In addition, we have confirmed close agreement between the theoretical and experimental CNR obtained with our contrast agent. The computer program also predicts adequate CNR with lower nanoparticle concentrations at higher field strengths. The mathematical modeling of signal intensities as a function of pulse sequence parameters and measured paramagnetic nanoparticle relaxivities provide a theoretical framework for interpretation of experimental and clinical image data, and for modification and evaluation of a broad range of molecular contrast agents. In addition, these quantitative models can easily be extended to evaluate other imaging pulse sequences (i.e., gradient echo), field strengths, and relaxivities. Based on the high relaxivities of these nanoparticles and the need for only picomolar concentrations, we anticipate that molecular imaging of pathologic molecules at preclinical stages of cardiovascular disease might be possible.
Figure 1. CNR as a function of TR (1.5T) from samples 2 and 3 relative to sample 1 determined experimentally (data points) and theoretically (solid lines).
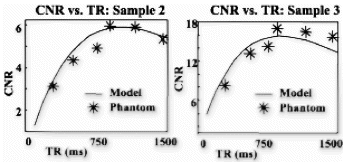
Table 1. Glycerol phantom properties and comparison of experimental and theoretical CNR values at 1.5T. The CNR of samples 2 and 3 were calculated relative to sample 1
References
1. Flacke S., et. al. Circulation, 104:1280, 2001.
2. Lanza G.M., et. al. Investig Radiol., 35:227, 2000.
3. Anderson S. A., et. al. Magn Reson Med., 44:433, 2000.
4. Ahrens E.T., et al. Proc Natl Acad Sci USA 95:8443, 1998.
5. Lanza et al. Circulation 2002, (in press).
155. Towards Rapid High Resolution Endovascular MRI
Claudia M. Hillenbrand, Ph.D.,1 Eddy Y. Wong, MSE,2 Frank K. Wacker, MD,1 Sherif G. Nour, MD,1 Shaoxiang Zhang, MD,1 Jonathan S. Lewin, MD,1 Jeffrey L. Duerk, Ph.D.1
Department of Radiology, University Hospitals of Cleveland, Cleveland, OH, USA, Department of Biomedical Engineering, Case Western Reserve University, Cleveland, OH, USA.
Purpose: To identify fast MRI sequences that provide high contrast, high SNR and high-resolution MR images of vessel walls under in vivo and/or in situ conditions when used in combination with endovascular coils.
Introduction: Atherosclerosis is a degenerative arterial disease that leads to the gradual blockage of vessels due to plaque formation or acute ischemic events such as plaque rupture and subsequent thrombus. The underlying cause of myocardial infarction and cerebrovascular stroke is, in most cases, the development and rupture of soft atherosclerotic (vulnerable) plaques in the coronary and carotid arteries respectively. Knowledge of artherosclerotic plaque composition (e.g. content of fat, fibrous tissue, calcification) in vivo would be a tremendous aid towards evaluation and selection of appropriate clinical interventions and timely detection of unstable atheroma.
Magnetic resonance imaging (MRI) of atherosclerotic plaque requires high spatial resolution (ideally less than 200μm), which is at present, only realized for superficial vessels (e.g. the carotid arteries) when standard surface coils are used for signal reception. To attain adequate signal to noise ratio for imaging of internal vessels, the use of catheter-based intravascular coils is required. However, many in vivo studies on endovascular MRI in literature report poor image quality from respiratory, cardiac, vessel and device motion as well as from blood flow artifacts during the long measurement times normally necessary to achieve the required resolution. Further, historically, most effort has focused on development of endovascular receiver coils, with less attention being paid to the appropriateness of the actual pulse sequences. Fortunately, the quality of fast sequence techniques like EPI, TrueFISP and HASTE have improved in parallel with the continued improvement of scanner hardware during the last years. Higher maximum gradient amplitude and faster slew rates in particular have offered opportunities for new sequences with promising opportunities even for intravascular MRI.
Therefore, the aim of this study was to evaluate whether a dedicated intravascular coil in combination with fast high-resolution MRI sequences is able to depict vessel wall features with high SNR, high CNR and acquisition times of less than a minute.
Material and Methods: High resolution MR imaging of the abdominal aorta and iliac artery was performed in animal imaging experiments (6 healthy domestic farm pigs and one Watanabe hyperlipidemic (WHHL-) rabbit) using a clinical 1.5T Siemens Sonata scanner. All procedures were performed in accordance with approved IACUC protocols. A catheter-based, opposed solenoid RF coil was developed for the imaging procedures. Images were acquired in vivo during sedation and in situ immediately after the animal was sacrificed. Imaging protocols included the use of SE-EPI, segmented EPI, HASTE, (turbo) spin echo schemes, and TrueFISP. Imaging parameters were. (a) SE-EPI (see Fig. ). ETL 40, TR/TE 1000/68ms, TA 28sec, SL 3mm, FOV 27×87mm2, matrix 40×128; (b) Segmented EPI (Fig. ). ETL 7, TR/TE 1000/26ms, TA 2min, SL 3mm, FOV 27×87mm2, matrix 39×128; (c) Segmented EPI (Fig. ). ETL 7, TR/TE 1070/38ms, TA 2:08min, SL 3mm, FOV 25×80mm2, matrix 80×256; (d) HASTE (Fig. ). TR/TE 3000/93ms, TA 3:10min, SL 3mm, FOV 41×54mm2, matrix 192×256; (e) TSE with dark blood preparation (double IR) (Fig. ). TR/TE 3537/123ms, TA 7:45min, SL 3mm, FOV 50×50mm2, matrix 256×256; (f) TrueFISP (Fig. ). TR/TE 7.5/3.8ms, TA 56sec/slice, SL 2mm, FOV 35×40mm2, matrix 112×128, and TR/TE 12/6ms, TA 43sec/slice (Fig. ). For in situ TrueFISP imaging the resolution was further enhanced by using the following parameters. TR/TE 17.5/8.7ms, TA 2min/slice, SL 2mm, FOV 28×28mm2, matrix 256×256. Images (Fig. ) were compared with conventional Spin Echo results (Fig. ). TR/TE 1800/77ms, TA 73min, SL 2mm, FOV 14×14 mm2, matrix 246×256.
The images were analyzed using qualitative criteria such as overall image quality, artifact behavior, vessel delineation and overall SNR and CNR anticipated to be necessary for future clinical evaluation. All images were compared to each other and to long duration vessel wall imaging protocols previously described in the literature and largely accepted as a gold standard today in vessel wall trials.
Results: Figure shows a representative example of all imaging sequences applied to one single pig. During in vivo imaging experiments, flow artifacts are barely visible, since water techniques like parallel saturation bands or dark blood preparation (see e.g. Fig. ) were successfully used to suppress signal from inflowing blood. Still, slight artifacts from the movement of the endovascular device were observed. The resulting artifacts were not felt to compromise image quality, and most importantly the depiction of the vessel wall was not affected.
As expected, multi echo methods like SE-EPI and HASTE, benefit from an intrinsic flow suppression due to their long effective TE. They are therefore ideal black blood techniques, but suffer in terms of signal to noise for the same reason.
Our results clearly show that TrueFISP, followed by segmented EPI offers the best image quality. The TrueFISP images shown in Fig. have an in plane resolution of 300μm and were acquired in less than a minute per slice: acquisition time was only 55sec/slice. Here, motion insensitivity was achieved by using short TE and TR (3.8ms, 7.5ms, respectively).
Spin echo acquisitions produced images with the best resolution (Fig. ), but required the longest imaging time during in situ experiments (from 12min to 73min when several averages were necessary). Even when used in situ, TrueFISP seems to be an interesting alternative (Fig. ) and provides similar imaging contrast in shorter acquisition times (2min/per slice) than e.g. TSE scans, that are one to two orders of magnitude longer in duration. All structures of the vessel wall identified in the SE image also appear clearly in the TrueFISP image.
Finally, Fig. demonstrates again the great opportunities TrueFISP is offering for fast intravascular MRI since these in vivo data were acquired in 43sec per slice.
Conclusion: The results suggest that the use of a dedicated intravascular coil in combination with fast imaging, especially TrueFISP, has the potential for in vivo characterization of vessel walls and identification of vessel disease.
Acknowledgments: This work was supported by DFG grant HI 787/1-1, NIH grants R33CA88144 and R01CA81431, and Siemens Medical Solutions.
156. Macrophage Targeted MRI Contrast Agents: Effect of Time, Dose, and Biochemical Environment
Walter J. Rogers, PhD,1 Partha Basu, PhD.2
Radiology and Internal Medicine, University of Virginia, Charlottesville, VA, USA, Biochemistry, Duquesne University, Pittsburgh, PA, USA.
Introduction: Atherosclerotic disease is a chronic inflammatory process in which endothelial dysfunction leads to the attraction and incorporation into the vessel wall of monocytes, macrophages and T-cells. Superparamagnetic iron oxide (SPIO) nanoparticles have an affinity for the monocyte-macrophage system, result in a focal region of signal loss on T2* magnetic resonance images and thus have been proposed as a means to determine the presence and location of active inflammation in the arterial system. However factors regulating the rate and extent of coated iron oxide particles is not known.
Purpose: The purpose of this study was to quantify the effect of dose, time and native and administered bio-molecules on SPIO uptake by macrophages.
Methods: Murine macrophages (J774A.1, American Type Culture Collection) were grown in Dulbecco's Modified Eagles's medium (DMEM) with 10% fetal Bovine serum (FBS). These macrophages are constitutively activated and contain most of the receptors found in human atherosclerotic macrophages. Cells were seeded into 8 well chamber slides. To determine the time course of uptake, a group of cells were fixed at 5, 10, 60, 240, 360, and 480 min. Dose effect was determined over the range of 2–16 μL of stock Feridex. One column served as a control the other treated with Human Interleukin-4 (IL-4, 40 ng/ml), Human Interferon gamma (IFN-γ, 1000U/ml), Mevinolin (HMG, 1.0 μM). Cells were incubated with the treatment agent for 24 hrs. Feridex (Berlex Labs) was then added (11.2 ng, 112.0 ng, 1.12 mg) for 4 hours. Slides were stained with acridine orange for the cell nucleus and cytoplasm and counter stained with Prussian Blue for iron.
Analysis: Slides were digitally scanned using a ChromaVision (San Juan Capistrano, CA) Automated Cell Imaging system. The system was calibrated to measure the area of the slide associated with Prussian Blue and the shade of orange associated with the macrophage nucleus. Iron concentration was expressed as the ratio of iron (blue area) to nuclear area (dark orange). This system permitted iron not contained within cells to be excluded. Non-viable cells were also excluded due to only pale orange staining of the cell nucleus.
Results: Figure shows the time-uptake relationship. Uptake at 5 min was significantly greater than background, however substantial uptake did not occur until 1 hour. SPIO uptake was significantly related to dose (Fig. r2=0.98) but the slope (0.35) indicated saturation of uptake at higher SPIO concentrations.
Figure shows macrophage uptake of SPIO as a percentage of control (pooled over all SPIO doses) for 3 bio-molecules. Both cytokines tested resulted in enhanced endocytosis of SPIO, while HMG treatment substantially inhibited uptake.
Conclusions: These results have significance for use of SPIO contrast agents for targeting the macrophages associated with atherosclerotic plaque. The in vitro uptake rate data indicate that sufficient blood residence time would be required to achieve an MRI observable macrophage SPIO concentration. Cytokines associated with vascular inflammation upregulate SPIO endocytosis. However the widely used lipid lowering class of drugs (statins) may significantly inhibit SPIO uptake even when macrophages are present. This latter effect has the potential for evaluation of anti-inflammatory drug therapy.
157. Combined Transesophageal and Surface MRI Plaque Volume Is the Most Reproducible Index of Aortic Atherosclerosis
Henning Steen, MD.
Cardiac MRI, Johns Hopkins Hospital, Baltimore, MD, USA.
Introduction: The usefulness of MRI to determine the evolution of atherosclerosis in clinical studies is defined by its reproducibility.
Purpose: We hypothesized that three-dimensional (3D) transesophageal MRI (TEMRI) measurements of aortic atherosclerotic plaque volume would be more reproducible indices of plaque burden than two-dimensional (2D) plaque area or one-dimensional (1D) plaque thickness.
Methods: Combined surface and TEMRI of the thoracic aorta was performed in 10 patients with documented cardiovascular disease. We obtained a second MRI within one week for reproducibility analysis of plaque size measurements. A TE receiver coil was positioned in the esophagus through a nasogastric tube. We selected the area of thoracic aorta with the thickest plaque seen on saggital scout images and obtained 6 cross-sectional images with 4mm slice thickness and no gap. Both T2 weighted (T2W) and proton-density-weighted (PDW) images were obtained during breath holds. Plaque measurements were performed by 2 independent blinded observers. We measured plaque thickness (mm) and plaque area (cm2) for each image and calculated the 3D plaque volume (cm3) for the composite of all images using Simpson's rule. Reproducibility was calculated for all parameters using Intraclass Correlation Coefficient (R) and Coefficient of Variation (CV).
Results: TEMRI measurement of plaque volume was the most reproducible method, with a higher R and a lower CV than plaque thickness or area (Table 1).
Conclusions: We found that 3D TEMRI measurements of aortic plaque volume are the most reproducible indices of plaque size, at least three times better than aortic plaque thickness or area. This method is suitable for prospective epidemiological studies and therapeutic trials of strategies aimed at altering aortic atherosclerosis.
158. AngioSURF-Based Assessment of Atherosclerotic Disease: Additional Findings and Impact on Patient Management in 200 Patients with Peripheral Vascular Disease
Mathias Goyen, MD,1 Jörg Barkhausen, MD,1 Christoph U. Herborn, MD,1 Knut Kröger, MD,2 Jörg F. Debatin, MD,1 Stefan G. Ruehm, MD.1
University Hospital Essen, Department of Diagnostic and Interventional Radiology, Essen, Germany, University Hospital Essen, Department of Angiology, Essen, Germany.
Introduction: Peripheral vascular disease (PVD) is frequently associated with coronary, renal, and carotid arterial disease. Localizing and gauging the severity of arterial lesions is crucial for therapeutic decision making. Recently, whole-body 3 D MR-angiography has become available.
Purpose: The purpose of our study was to assess the potential of whole-body 3D MR-angiography with regard to the display of additional atherosclerotic lesions in patients initially referred for the MR-based assessment of the peripheral vasculature.
Methods: Within a 22 month-period 203 consecutive patients with PVD were prospectively examined by means of whole-body 3D MRA using the AngioSURF-system (MR-Innovation GmbH, Essen/Germany) on a 1.5 T MR-Scanner (SIEMENS Sonata®, Erlangen, Germany). Five 40 cm volumes were prescribed. A 3D FLASH sequence was collected over 12 s (table repositioning. 3 s, total scan time. 72 s). Gd-BOPTA (Multihance®, BRACCO, Italy) was power-injected using a biphasic injection protocol at a dose of 0.2 mmol/kg BW. For image analysis the arterial tree was divided into 30 segments per patient. Arterial disease documented by whole body 3D MRA outside the peripheral tree was confirmed if deemed necessary by the referring clinician. All correlative imaging studies performed within 60 days of whole body MRA were included in the efficacy analysis.
Results. Three patients with technical failure of CE-MRA were excluded from the evaluation. In the remaining 200 patients the exam rendered diagnostic image quality from the carotid arteries to the tibial vessels. Apart from the proven PVD additional clinical relevant disease was found in 21%. 32 patients had a renal artery narrowing, in 24 patients a carotid arterial stenosis was detected, three patients had a stenosis of the subclavian artery, and four patients showed an AAA. Confirmatory studies performed on 23 patients in this study revealed no false positive or false negative exams (Fig. ).
Conclusions: Noninvasiveness, three-dimensionality, extended coverage and high contrast conspicuity are the characteristics of the applied whole body 3D MRA-approach allowing for a quick, risk-free, and comprehensive evaluation of the arterial system in patients with atherosclerosis.
159. Improved Adjacent Region Function Mediates Benefits of AT2-R Overexpression Post-MI
Christina M. Bove, MD, Christopher D. Scott, Zequan Yang, MD, PhD, Joseph M. DiMaria, BA, Wesley D. Gilson, MS, Frederick H. Epstein, PhD, Stuart S. Berr, PhD, Brent A. French, PhD, Christopher M. Kramer, MD.
Departments of Medicine, Radiology and Biomedical Engineering, University of Virginia, Charlottesville, VA, USA.
Introduction: Angiotensin II type II receptor (AT2-R) overexpression in the mouse heart improves LV systolic function at baseline and preserves left ventricular (LV) size and global LV function during post-MI remodeling1. Regional heterogeneity of myocardial function occurs in non-infarcted regions after acute-MI2. Regions adjacent to the infarct demonstrate greater and persistent dysfunction and are associated with LV remodeling post-MI2. Myocardial tagging can detect regional differences in contractile function post-MI in mice3.
Purpose: We hypothesized that 1) MRI tagging would localize regional improvements in myocardial function during post-MI remodeling in AT2-R overexpressed mice and that 2) improvement in adjacent zone function is responsible for the preservation of global LV function post-MI seen in these mice.
Methods: Animal Model: Six male wild type (WT) C57BL/6 mice and 10 transgenic (TG) mice which overexpress the AT2-R in the heart were studied by cardiac MRI at baseline (day 0) and days 1, 7, and 28 post-MI. MI was induced by a 1 hour occlusion of the LAD followed by reperfusion.
Mouse Imaging: Anesthesia was induced with 3.0% inhaled isofluorane and maintained with 1.0% isofluorane. Cine and tagging MRI was performed on a Varian Inova (Varian Inc., Palo Alto, CA) 4.7T MRI scanner with a custom-built 2.5cm Litz RF coil (Doty Scientific, Columbia, SC). Twenty minutes prior to imaging on day 1 post-MI, 0.3mM/kg gadolinium-DTPA (Gd) was infused intraperitoneally to assess infarct size. An ECG-triggered 2D cine FLASH sequence was used to obtain 7–8 1 mm thick short axis slices from apex to base. Cine imaging used TE=3.9ms and TR was adjusted (8.0–14.0ms) to obtain 16 phases per cardiac cycle. A 20° flip angle was used except on day 1 when a 60° flip angle was used to increase the amount of T1 weighting in post-Gd imaging. A 2.56cm×2.56cm FOV was used with a matrix size of 128×128. The tagging sequence used a double inversion recovery black blood SPAMM sequence4 with a multiphase FLASH acquisition and were acquired in two orthogonal directions. Specific parameters included TR=8–12ms, TE= 5.5ms, FOV=2.56cm×2.56cm, matrix size=192 (readout)×96 (phase encode), slice thickness 1mm. The composite SPAMM flip angle was 180° and tag separation was 0.7mm.
Data Analysis: The infarct region was defined as the area of delayed hyperenhancement in day 1 post-Gd images where the signal intensity was greater than 2 standard deviations above the mean of remote regions. Percent circumferential shortening (%CS) was measured from tagged images using the Findtags program and 12 sectors per slice. Epicardial and endocardial borders for tag analysis were traced from the black blood images. A 12 sector model was aligned such that 2 sector borders matched the Gd-enhanced borders. Sectors within the Gd-enhanced region were termed infarct (inf), those bordering either side of the infarct in the short axis were termed adjacent (adj), and all remaining sectors remote (rem). On day 1 images, 2 slices containing approximately 40–60% of the myocardial area with transmural contrast enhancement were subject to strain analysis. For other timepoints (bsl, d7, and d28), corresponding slices were chosen for strain analysis, with care taken to align the sectors in the same anatomic locations for each corresponding slice. Mean %CS was calculated within each zone at each time point in WT and TG mice (See Fig. ). Two-way ANOVA confirmed intragroup differences by region and time point. Regional and between group differences in %CS were analyzed using pairwise multiple comparisons subtesting (Tukey test). Data are presented as mean±S.E.
Results: Infarct size was similar in WT and TG mice, 43.6±4.5% and 44.4±6.9% of LV mass respectively (p=NS). In the segments defined as infarcted, while there was higher %CS in TG mice compared to WT at day 0 (14.8±0.3% vs. 12.0±0.4% respectively, p<0.001), %CS was similar by day 28 (1.6±0.4% for TG vs. 0.4±0.7% for WT, p=0.10). Similarly, for remote regions, although %CS was higher in TG at day 0 (14.2±0.4% vs. 12.0±0.6% for TG vs. WT, p=0.004), by day 28 there was no difference (12.6±0.5% vs. 12.6±0.6%; TG vs. WT, p=1.00). Within adjacent zones, while there was no significant difference in %CS at day 0 or 1 in TG vs. WT, %CS was significantly higher in TG compared to WT by day 7 (10.3±0.7% vs. 6.9±1.0% respectively, p=0.005), and these changes persisted out to day 28 post-MI (9.3±0.7% vs. 5.9±1.0%, p=0.004) (See Fig. ).
Conclusion: Myocardial tagging can detect regional differences in contractile function post-MI in mice that overexpress the AT2-R. AT2-R overexpression in the murine heart is associated with improved contractile function in adjacent, non-infarcted myocardium starting at day 7 post-MI which persists to day 28. This improvement in adjacent zone function likely explains the improvement in global LV size and function during post-MI remodeling seen with AT2-R overexpression.
Figure 1. End-systolic tagged images (A,B) and corresponding %CS color maps (C,D) on day 28 post-MI of a WT (A,C) and TG (B,D) mouse. Note the cavity dilation and wall thinning in the infarct zone in WT compared to TG.
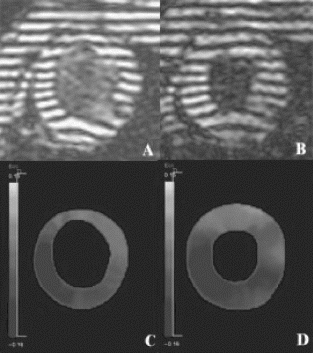
References
1. Yang Z, et al. Circulation. 2002; 106:106–111.
2. Kramer CM, et al. Circulation 1993; 188:1279–1288.
3. Epstein FH, et al. Magn Reson Med. 2002; 48:399–403.
4. Epstein FH, et al. JCMR 2002; 4:113–114.
160. A Switchable Multi-coil Array Optimized for Endovascular Procedures and High Resolution Vessel Wall Imaging
Claudia M. Hillenbrand, Ph.D.,1 Eddy Y. Wong, MSE,2 Daniel R. Elgort, MSE,2 Shaoxiang Zhang, MD,1 Frank K. Wacker, MD,1 Jonathan S. Lewin, MD,1 Jeffrey L. Duerk, Ph.D.1
Department of Radiology, University Hospitals of Cleveland, Cleveland, OH, USA, Department of Biomedical Engineering, Case Western Reserve University, Cleveland, OH, USA.
Purpose: To design and test applicability of an endovascular catheter probe optimized for both MR tracking and high-resolution plaque imaging.
Introduction: The ultimate goal of endovascular MRI guided interventions is the combination of MRI's diagnostic capabilities (e.g. angiography, plaque analysis, perfusion imaging) along with therapies like angioplasty, atherectomy, stent placement or other interventional therapies. It is planned that the diagnosis and therapy can be performed using MR alone. Clearly, this goal can only be achieved with integrated, multi-functional devices. Therefore, the aim of this study was to develop and test a configuration of two independent solenoid coils mounted on a catheter to fulfill two important functions required for future MRI guided endovascular therapy: 1) MR guidance of the interventional device to the target region (e.g. an atherosclerotic plaque lesion), and 2) high resolution imaging at the target location. In our implementation, we sought to determine if two independent coils, wound in opposite direction, can be combined so they produce the sensitivity and signal characteristics of a conventional opposed solenoid coil for high resolution imaging while also permitting active catheter tracking from the signal generated in one (or two) of the solenoid coils independently.
Material and Methods: Two independent solenoid coils were wound in opposite direction on a cylindrical form (Fig. ). When considered together, the design and geometry were similar to that of a conventional opposed solenoid antenna. However, each coil is (a) individually tuned to 63.6 MHz, (b) matched to 50 Ohm, and (c) connected to a separate receive channel of the MR system. Each coil can therefore be turned on or off independently during the MR experiment. This has the advantage that signals from the single coils can be reconstructed/processed together or independently. 30 AWG copper wire was used for both coils. Each coil diameter was 5F. Each solenoid probe consisted of 5 windings and had a length of 4.5mm. The gap between the coils was 1cm. Tuning and matching were performed on the tip of the catheter. Passive decoupling of each single independent coil during excitation was accomplished using crossed diodes. They too were integrated into the tip of the catheter. The coil was encased in biocompatible polymer shrink tubing over the distal 40 cm length. The maximum outer diameter of the prototype imaging/tracking catheter was 12F. Small diameter transmission lines and interface connectors were used to link the coils to independent channels of a Siemens Magnetom Sonata 1.5T whole body scanner. RF excitation was performed using the standard body coil.
For phantom imaging experiments, the catheter probe was placed in a vessel wall phantom consisting of two concentric NMR sample tubes, sealed at one end, and inserted into a 200ml plastic bottle. The cylindrical cavities were filled with different solutions of saline and copper sulfate to establish different contrasts between the inner lumen (the inner lumen of the small diameter tube), the simulated vessel wall (outside the inner tube yet inside the larger diameter) and the simulated non-vascular tissue (outside the larger sample tube within the bottle). Tracking capabilities of the coil configuration were tested using an automated tracking method implemented previously by our group {D.Elgort et al, Proc ISMRM 2002, p17}. Signals from each coil were processed independently. In addition, multi-slice proton density weighted FLASH images were acquired in order to study the signal characteristics of the coil array. Combined images were calculated from signals received simultaneously from both elements. These images were compared to those from a single channel opposed solenoid coil normally used for high resolution imaging in our lab. All coils had identical dimensions and primary winding distributions.
Results: The location of each coil could be determined independently. During tracking, each coil gave rise to a single high amplitude peak in the projection data sets acquired with each RF channel. The projection data from the two RF channels are acquired simultaneously. The location information could be used for successful device tracking and automated slice positioning (location and orientation) for high resolution imaging experiments.
Figure shows representative slices from the FLASH experiments designed to explore the spatial sensitivity pattern of the multi-coil implementation in comparison to a conventional opposed solenoid. Specifically, Fig. shows the spatial sensitivity of the individual coil elements. The combined image formed from the square root of the sum of squares images are compared to the respective slices from a conventional opposed solenoid design. The combined coil sensitivity profile is similar to the conventional opposed solenoid design. Specifically, the sensitivity of the coil array is highest adjacent to its outer wall and drops off rapidly and it is radially symmetrically. The regions of zero sensitivity that are inherent to the (opposed) solenoid designs are also clearly visible in all images.
Discussion and Conclusion: MR compatible catheter designs presented in literature to date focus either on device tracking or on high resolution imaging using dedicated endovascular micro-coils. Here, we show a new method for MR tracking and high resolution imaging using a single fixed coil configuration. In combination with 3 gradient projections, each coil provides the opportunity for device position tracking. If tracking is done with both coils, device position and orientation can be determined. Finally, the design provides opposed solenoidal imaging performance if the signals from the two independent coils are combined during an imaging sequence. Hence, the proposed catheter-mounted array allows the independent coils to be used individually for tracking as well as in a combined mode for high resolution imaging, without seriously compromising coil sensitivity.
Figure 2. Comparison of sensitivity profiles of the opposed solenoid array elements and the conventional opposed solenoid coil.
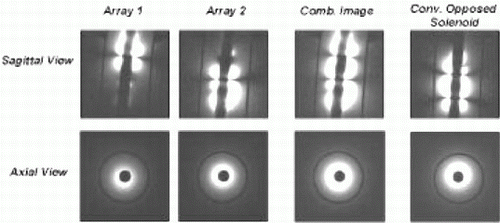
Acknowledgments: This work was supported by DFG grant HI 787/1-1, NIH grants R33CA88144 and R01CA81431, and Siemens Medical Solutions.
161. Serial In Vivo Magnetic Resonance Imaging of Magnetically Labeled Stem and Progenitor Cells Using Micron Scale Iron Oxide Particles.
Jonathan M. Hill, MD,1 Alexander J. Dick, MD,1 Venakatesh K. Raman, MD,1 Richard B. Thompson, PhD,1 Erik Shapiro, PhD,2 Breno S. Pessanha, MD,1 Cynthia E. Dunbar, MD,3 Robert J. Lederman, MD.1
Cardiovascular Branch, National Institutes of Health, Bethesda, MD, USA, National Institutes of Health, Bethesda, MD, USA, Hematology Branch/NHLBI, National Institutes of Health, Bethesda, MD, USA.
Introduction: Serial in vivo tracking of transplanted stem and progenitor cells for cardiovascular cell therapy could offer biological insights into homing properties, environmental niches, and the efficiency and time course of engraftment. As the use of cell therapies for myocardial regeneration and arteriogenesis applications increases, this knowledge will be central to assessing safety, efficacy and basic biologic properties of each cell population. Magnetic resonance imaging offers a desirable technology to follow the distribution of cells, in vivo, following transcatheter direct myocardial delivery or to assess the efficiency and time course of intravenous homing to sites of myocardial injury. Ultra-small dextran-coated iron oxide particles have previously been developed for uptake into cells, allowing imaging via MRI, with preliminary success in animal models of neurotransplantation and hematopoietic stem cell homing. Real-time imaging of labeled cells in beating heart has not previously been demonstrated. Here we present a novel use of a class of much larger micron-scale iron oxide magnetic particles with enhanced MR susceptibility. These particles, in addition, possess a fluorophore, which permits flow cytometric analysis and histological confirmation of labeling efficiency.
Purpose: To establish in vitro feasibility and toxicity of stem and progenitor cell labeling using a fluorescent micron scale iron oxide particle(IFP). To serially image in vivo transplanted cells and to recover fluorescent and magnetically labeled cells at histology.
Methods: Skeletal myoblasts, endothelial progenitor cells, hematopoeitic stem cells and mesenchymal stem cells were isolated from porcine and human sources. A micron scale iron oxide particle was admixed with a flurophore and encased in an inert di-vinyl benzene shell. Using a 1% solids solution a range of concentrations from 0.1μl/ml–50μl/ml was added to cell culture. Growth and in vitro differentiation was assessed using a modified colorimetric MTT assay and standard colony proliferation assays. For adherent cell populations non-endocytosed particles were removed by vigorous washing. Cells could then be trypsinized in preparation for transplantation. To assess in vitro relaxometry characteristics agarose suspensions of labeled cells were prepared at varying concentrations. In a swine closed chest model of myocardial infarction, using coil embolization, labeled cells were delivered using an X-ray guided endomyocardial delivery catheter (Stiletto™, Boston Scientific, Natick MA). Following cell delivery animals underwent serial cardiac MRI (Siemens Sonata, Erlangen, Germany) up to 3 weeks.
Endomyocardial injection sites were identified using tissue fast dyes admixed with the labeled cells to aid in heart sectioning following sacrifice. Hearts were preserved by snap freezing and tissue sections imaged using fluorescence confocal and differential interference microscopy.
Results: We demonstrated dose-dependent and highly efficient perinuclear uptake of IFP into skeletal myoblasts, endothelial progenitor cells, hematopoietic CD34+ stem cells and mesenchymal stem cells documented by confocal microscopy and electron microscopy. After overnight labeling particle uptake was greater than 95% with virtually every cell containing a high density of particles encased within membrane-bound cytoplasmic organelles. There was minimal toxicity of the labeling process, as measured by in vitro assays of primitive hematopoietic cell function and in vitro differentiation assays. In MRI studies, both CD34+ cells and MSCs loaded with these microparticles were very easily resolved at single cell resolution. In vitro relaxometry measurements showed a marked T2* susceptibility with little or no T1 effect (Fig. ). Following direct endomyocardial delivery of 105–106 labeled MSCs into normal and infarcted myocardium MSCs could be readily detected by MRI immediately after injection (Fig. and were serially imaged up to 3 weeks (Fig. ). Following sacrifice 3 weeks after cell delivery explanted hearts were imaged using high resolution to further image sites of labeled cell delivery (Fig. ) IFP-labeled cells could be reproducibly recovered using fluorescence confocal microscopy at sites corresponding to signal void on MRI.
Conclusions: Micron scale iron oxide particle labeling of stem and progenitor cells for cardiovascular cellular therapeutics offers a promising tool for assessment of in vivo homing, migration and engraftment. IFP-labeled stem and progenitor cells maintain proliferative and differentiation characteristics suggesting potential clinical utility of this application.
162. Precise Targeting of Endomyocardial Injection of Stem Cells to Porcine Myocardial Infarction Using Real-Time Magnetic Resonance Imaging
Alexander J. Dick,1 Michael A. Guttman,2 Venkatesh K. Raman,1 Dana C. Peters,2 Jonathan M. Hill,1 Scott Smith,3 Greig Scott,3 Elliot R. McVeigh,2 Robert J. Lederman.1
Cardiovascular Branch, National Heart Lung and Blood Institute, Bethesda, MD, USA, Laboratory of Cardiac Energetics, National Heart Lung and Blood Institute, Bethesda, MD, USA, Boston Scientific Scimed, Plymouth, MN, USA.
Introduction: Catheter navigation and myocardial injections have previously been demonstrated under real-time magnetic resonance imaging (RT-MRI) guidance. Potential applications include transcatheter injections of therapeutic cell preparations. To be clinically useful, such an imaging technology should simultaneously provide robust identification of myocardial viability and targeting of the delivery device.
Purpose: We test the feasibility of RT-MRI for both the identification of myocardial infarctions (MI) and targeted delivery of mesenchymal stem cells (MSCs).
Methods: Porcine MSCs were dually MRI- and fluorescence-labeled using intracellular iron-fluorophore particles (IFPs). In five mini-swine, one to seven days prior to the RT-MRI study, MI was induced by percutaneous coronary artery coil-occlusion. Transfemoral delivery catheters and injection needles (Stiletto™, Boston Scientific) were modified to serve as MRI receiver coils. The coaxial guiding and injection needle were color-highlighted in green and red respectively. A 1.5T clinical MRI scanner was customized with rapid image reconstruction and interactive features to enhance interventional guidance. Contrast between normal and non viable infarcted myocardium was enhanced by intravenous injection 20 minutes prior to imaging of 0.2mmol/kg Gd-DTPA resulting in delayed hyperenhancement of the infarct.
Results: Real-time steady state free-precession (RT-SSFP) imaging with echo-sharing yielded 7 frames/s of 1.7×3.3×8mm voxels. Myocardial infarctions were clearly identifiable in real-time by patterns of myocardial contraction and delayed hyperenhancement. The average contrast to noise ratio between infarcted and normal myocardium was 23±7.3 for RT-SSFP delayed hyperenhancement imaging. Catheter navigation and two to six injections were performed in each pig wholly under RT-MRI guidance. Individual color highlighting of images produced by receiver coils within the guiding catheter and Stiletto allowed targeting of the needle position to infarct and its borders. Targets were selected based on hyperenhancement and wall motion abnormalities of infarcted tissue. IFP-labeled MSCs were readily visualized in vivo by MRI as a signal void and post-mortem using confocal fluorescence microscopy.
Conclusion: Targeted delivery of potentially regenerative cellular treatments to infarcted myocardium is feasible using multiple catheter coils and RT-MRI visualization of catheter navigation, myocardial function, myocardial viability, and labeled cells.
163. Intravascular Catheter Tracking with Adaptive Image Parameters
Daniel R. Elgort,1 Eddy Wong,1 Claudia Hillenbrand,2 Frank K. Wacker,2 Jonathan S. Lewin,2 Jeffrey L. Duerk.2
Biomedical Engineering, Case Western Reserve University, Cleveland, OH, USA, Radiology, University Hospitals of Cleveland, Cleveland, OH, USA.
Objective: Adjusting the MR image parameters (e.g., slice position, orientation, resolution, TE, TR, etc.) during an interventional catheter-based procedure is usually a cumbersome process that oftentimes requires the interventionalist or technologist to leave the magnet room and use a keyboard and a mouse in combination with a graphical user interface. This work aims to provide the interventional radiologist with a means to affect a change in specific image parameters, in real-time, using nothing more than the catheter itself. An active tracking system is implemented which not only updates the scan plane position and orientation, but also monitors the catheter's insertion velocity. Changes in catheter velocity are automatically used by the scanner to adjust image resolution, signal-to-noise ratio, field of view, slice thickness, or even adjust contrast properties. This allows the radiologist to seamlessly transition from rapid survey imaging to high-resolution interrogation imaging simply by slowing the catheter's velocity of insertion.
Methods and Materials: An existing automated tracking method implemented by our group on a Siemens 1.5T Sonata imager was modified to allow for adaptive image parameters during data acquisition. The tracking software uses an analytic radial localization technique (Flask et al. JMRI 14:617–627(2001)) that determines the current position and orientation of the catheter in 3D space prior to acquisition of image data. Localization from multiple time points is used to calculate the insertion velocity of the catheter. The system uses the output of the velocity calculation to adjust specific adaptive parameters (FOV, resolution, slice thickness, SNR, TR, TE, etc.) of the sequence. The adaptive parameter's can be adjusted by the scanner in two modes. In a continuous mode, the parameters are varied according to a sigmoidal function of the catheter velocity. Upper/lower asymptotes prevent pulse sequence parameters that would exceed hardware limitations. These functions also have static sensitivities and default set points that can be adjusted via the user interface. There is also a binary mode in which the scanner determines if the catheter is moving or stationary, and sets specific imaging parameters accordingly. To evaluate the system's feasibility and performance in vivo and ex vivo, trials were conducted in two vessel phantoms and four porcine imaging experiments (approved by our institutions IACUC).
Results: The system collected all of the necessary tracking data within 40 ms; an additional 10 to 20 ms was then required to perform the localization, velocity calculations, and update the image parameter values. Based on results from in vivo experiments, the system is able to accurately (within 2 mm) localize a motionless catheter 100% of the time, and a moving catheter 98% of the time. The system was running continuously and responding in real-time to calculated changes. Following parameter determination, the system successfully responded to changes in device velocity by dynamically adjusting specified image parameters. In both the phantom and porcine trials, the resolution and FOV were automatically varied. The images below (Figs. ) illustrate a temporal sequence in which the catheter is slowed to a stop; one set of images is in vivo data collected in a porcine experiment in which the catheter was inserted throughout the length of the abdominal aorta; the other set of images is data collected in a vessel phantom experiment. In all cases, the slice plane location and orientation was automatically placed at the catheter tip using information extracted during the localization phase.
Conclusions: Allowing the MR scanner to automatically respond to a moving catheter by adjusting the value of imaging parameters creates a more natural interface with the MR scanner for the clinician during intravascular procedures by eliminating the need for manual adjustment of the scan plane position or specific image parameters during the intervention. These scan plane adjustments are applied to imaging slices that are also following the location and orientation of the catheter. Hence, simply advancing the catheter more slowly will automatically improve the resolution or SNR properties of the images, or can even effect a total change in tissue contrast to allow more accurate characterization of vessel wall pathology if the clinician wishes to see more detail in a certain region of interest. This technology offers a completely new method for intravascular imaging and performance of image guided intravascular procedures.
Acknowledgments: Work was supported by Siemens Medical Solutions, grant DFG HI 787/1-1, and NIH grants R33CA88144 and R01CA81431.