Abstract
The magnetic anisotropies and easy axes of magnetization at room temperature were determined, and the effects of rare-earth (RE) ions were clarified for RE-based cuprates, RE-doped bismuth-based cuprates and RE-doped Bi-based cobaltite regarding the grain orientation by magnetic field. The easy axis, determined from the powder orientation in a static field of 10 T, depended qualitatively on the type of RE ion for all three systems. On the other hand, the magnetization measurement of the c-axis oriented powders, aligned in static or rotating fields, revealed that the type of RE ion strongly affected not only the directions of the easy axis but also the absolute value of magnetic anisotropy, and an appropriate choice of RE ion is required to minimize the magnetic field used for grain orientation. We also studied the possibility of triaxial grain orientation in high-critical-temperature superconductors by a modulated oval magnetic field. In particular, triaxial orientation was attempted in a high-oxygen-pressure phase of orthorhombic RE-based cuprates Y2Ba4Cu7Oy. Although the experiment was performed in epoxy resin, which is not practical, in-plane alignment within 3° was achieved.
Introduction
Functional compounds with anisotropic crystal structures, such as layered structures, generally exhibit anisotropic physical properties and possess functionally superior crystallographic structure. In particular, the effect of the anisotropy on electronic transport properties is a major factor determining the functionality of thermoelectric and superconducting materials. For applications of these layered functional compounds as bulk materials, a grain orientation process is intrinsically indispensable. Moreover, in the case of superconducting materials based on copper oxides, which are high-critical-temperature (Tc) cuprate superconductors, not only the c-axis grain orientation of superconducting CuO2 planes extending in the ab-planes but also the alignment of crystallographic axes in the CuO2 planes, that is, triaxial or biaxial grain orientation, is required for practical use.
Magnetic orientation is one of the most useful methods of grain orientation. The recent development of superconducting magnets has realized the 10 T class of high static fields in a room-temperature bore without using liquid helium, and these magnetic fields can provide a kinetic energy comparable to gravity and thermal energy at room temperature, even for feeble magnetic materials, paramagnetic and diamagnetic materials. Therefore, magnetic orientation using cryogen-free superconducting magnets is one of the most useful techniques for layered compounds with feeble magnetic properties at room temperature. The materials requirements for the achievement of magnetic orientation are sufficiently large magnetic anisotropy at room temperature and the desired easy axis of magnetization. Although understanding these magnetic properties at room temperature is important for grain orientation, the factors determining magnetic anisotropy and the easy axis in functional layered oxides and methods for controlling these properties have not been clarified yet.
In this study, we focus on three different layered oxide systems, REBa2Cu3Oy (RE123), Bi2Sr2CaCu2Oy, (Bi2212) and Bi2Ca2Co2Oy (BiCa222), the former two of which are high-Tc cuprate superconductors with a CuO2 layer and the latter is a candidate thermoelectric oxide with a CoO2 layer. Figure shows the crystal structures of these three compounds, all of which possess a layered structure with alternately stacked blocking layers and two-dimensional TMO2 (TM=Cu and Co) layers. To clarify the possibility of control of the easy axis of magnetization and of the magnetic anisotropy between the in-plane and out-of-plane directions by RE ions, the magnetic roles of RE in RE123, RE-doped Bi2212 and RE-doped BiCa222 were investigated from the viewpoint of the magnetic susceptibility of magnetically oriented powders. Furthermore, we also discuss the possibility of formation of a triaxially oriented microstructure in high-Tc cuprates by magnetic field. We focus on Y2Ba4Cu7Oy (Y247, y∼15), which is a superconducting compound related to Y123 and does not possess a twin microstructure in its grains. We also attempted to fabricate a triaxially oriented powder in epoxy resin using a modulated oval magnetic field [Citation1]. This work is very important from the viewpoint of the practical use of RE-based cuprates because the symmetry of superconducting quasi-particles is d-wave, and because a change in the superconducting current depending on the misorientation angle at a single grain boundary emerges even for the in-plane direction [Citation2].
Experimental details
Powders of RE-doped BiCa222, RE-doped Bi2212 and RE123 with an average grain size of 1–3 μ m were synthesized by a solid-state reaction. Details of the synthesis conditions are as follows: Bi2 (Ca0.95RE0.05)2Co2Oy [Bi(Ca,RE)222] powders were synthesized from Bi2O3, CaCO3, RE2O3 and Co3O4 starting powders. After calcination at 750 °C for 12 h in air and thorough grinding, mixtures with an appropriate cationic ratio were sintered at 820 °C for 24 h in air. Ball-milling of the sintered powders was performed to prepare fine powders. Bi2Sr2 (Ca0.5RE0.5)Cu2Oy powders were obtained from Bi2O3, SrCO3, CaCO3, RE2O3 and CuO starting powders. After calcination at 790 °C for 12 h in air and thorough grinding, mixtures with an appropriate cationic ratio were sintered at 820 °C for 24 h in air. RE123 powders were synthesized from RE2O3, BaCO3 and CuO powders. Mixtures with an appropriate cationic ratio were sintered at 850–900 °C for 36 h in flowing gas mixture 1% O2 + 99% Ar for RE = La to Gd and Yb and at 880–900 °C for 18 h in air for RE = Dy−Tm. The oxygen contents of the RE123 powders were controlled to y∼6.9 by post-annealing at 400 °C in flowing oxygen.
For all the powders, magnetically aligned (MA) powder samples were fabricated in epoxy resin, which was cured for more than 12 h at room temperature under a static magnetic field (Ha static) of 10 T. The weight ratio of the powder and epoxy resin was 1 to 10. The easy axis of magnetization for each compound was determined from the x-ray diffraction (XRD) pattern of the surface perpendicular to Ha static using each MA powder sample. For RE123 compounds with the easy axis in the ab-plane, c-axis oriented powder samples were prepared in a rotated magnetic field (Ha rotated) of 12 T at room temperature. In this case, the powders in epoxy resin were horizontally rotated at 30 rpm in a transverse magnetic field of 12 T during the curing of the epoxy resin. The difference in magnetic susceptibility parallel to the c-axis (χ c) and in the ab-plane (χ ab) at room temperature (Δ χ = χ c - χ ab) was determined using a SQUID magnetometer for the c-axis oriented powder samples of RE123. The mass of RE123 powders in epoxy resin was determined by the Archimedes method.
Results and discussion
Effects of RE-doping on magnetic anisotropy and easy axis of magnetization
To study the magnetic effects of paramagnetic RE ions at room temperature, the easy axes of magnetization and Δ χ values were determined. XRD patterns for the MA powder samples aligned in a static field reveal that RE123 compounds with RE = Y, La, Pr, Nd, Sm, Dy and Ho exhibited an easy axis parallel to the c-axis and those with RE = Eu, Gd, Er, Tm and Yb had an easy axis in the ab-plane. In particular, RE123 compounds with the easy axis in the ab-plane are not desirable for the evaluation of magnetic anisotropy between the in-plane and out-of-plane directions. This is because a certain level of χ ab includes a component of the magnetic susceptibility normal to Ha static in these MA samples. Therefore, c-axis oriented powders are required. We attempted c-axis orientation using a rotated magnetic field, under which the hard axis of magnetization is aligned perpendicular to the Ha rotatedplane [Citation3, Citation4]. As a result of such grain orientation, c-axis oriented powder samples were successfully fabricated for RE = Eu, Er, Tm and Yb, and it was found that the rotated field was effective for the c-axis orientation of RE123 compounds with χ c < χ ab.
Figure shows the relation between the number of 4f electrons and Δ χ for the RE123 compounds, which was determined from magnetization measurements in directions parallel to the c-axis and ab-plane using the c-axis aligned powders samples. Clearly, Δ χ largely depended on the type of RE, and |Δ χ | in RE123 with a heavy RE was larger than that in RE123 with a light RE. The absolute values of Δ χ, |Δ χ |, were on the order of 10−4 for RE = Dy, Ho, Er and Tm. They were 4–20 times larger than that of Y123, and therefore the calculated Hast for magnetic grain orientation in the case of Dy123 is approximately 5 times lower than that for Y123. This behavior has also been observed qualitatively in oxygen-reduced RE123 compounds with y< 6.5 [Citation5], indicating that the roles of RE ions are basically preserved upon a change in the local crystal structure around the RE ions.
To investigate the universality of the magnetic roles of RE ions, RE doping was attempted for Bi2212 and BiCa222 as another superconducting cuprate and another functional layered oxide, respectively. Their easy axes of magnetization were revealed from the XRD measurement of these MA samples. Figure shows the XRD patterns of the MA samples of Bi(Ca,RE)222, together with the patterns of the MA sample of RE-free BiCa222. In the case of RE-free BiCa222, only (200) and (020) peaks were enhanced by the static magnetic field. That is, the easy axis for RE-free BiCa222 lies in the ab-plane, parallel to the thermoelectric CoO2 layers. The easy axis is derived from the magnetic anisotropy of the CoO2 layers because cations other than Co ions, that strongly affect the magnetic properties, are not present in the system.
For the doping of RE = Y−Gd, the XRD patterns were roughly coincident with that of the RE-free sample. Therefore, the easy axis was preserved in the ab-plane, and 5 mol% of doping by RE did not affect the magnetic anisotropy and easy axis of magnetization in the case of doping of a light RE. However, for the doping of RE = Tb−Ho, the (200) and (020) peaks disappeared and only (00l) peaks were seen. Even at a low doping level of RE, the easy axis was converted completely from the ab-plane to the c-axis direction. Moreover, in the case of RE = Er−Yb, clear enhancement of the (200) peak was observed, indicating that the magnetic anisotropy of RE123 was also enhanced, with the easy axis in the ab-plane (ab-plane magnetic anisotropy). These results mean that heavy RE ions affect the magnetic anisotropy and easy axis more than light RE ions. This clear difference in the magnetic roles of light and heavy RE ions is qualitatively consistent with the results for RE123 obtained from figure .
We investigated the effects of doping RE = Eu, Er, Tm and Yb, which had the ability to enhance the ab-plane magnetic anisotropy in BiCa222, on the easy axes for Bi2212 with the easy axis parallel to the c-axis. Figure shows XRD patterns of the MA samples for RE-doped Bi2212 (RE = Eu, Er, Tm and Yb), together with the patterns for the MA sample of RE-free Bi2212. As mentioned above, the easy axis for RE-free Bi2212 is the c-axis. In the case of RE = Er and Tm, although RE doping induced a Bi2Sr2CuOy (Bi2201) phase, the (00l) peaks disappeared and the (200) and (020) peaks were markedly enhanced. Namely, the easy axis was converted from the ab-plane to the c-axis by the doping of Er and Tm. However, in the case of RE = Eu and Yb, the 50% substitution of RE is insufficient for the complete conversion of the easy axis into the ab-plane. This is probably because the ab-plane magnetic anisotropies generated by the 50% doping of these two RE ions were smaller than or comparable to the intrinsic c-axis magnetic anisotropy of the CuO2 plane in RE-free Bi2212. This result also demonstrates that the ability to control the easy axis and magnetic anisotropy depended on the type of RE.
The easy axes of the three functional layered oxides obtained in this study are summarized in table , also with the sign of the second-order Stevens factor, α J, [Citation6, Citation7] for each RE ion. From the comparison between the directions of the easy axis in RE123 and the sign of αJ, the easy axis was clearly correlated with the sign of α J and can be explained in terms of the single-ion anisotropy of RE3 +. However, the absolute values of Δ χ are significantly different between light and heavy RE ions, which is in contrast to the magnetic anisotropy of RE metals [Citation8]. Note that the calculated magnetic anisotropy constants for the heavy and light RE were almost the same. This is presumably due to differences in the structural symmetry around the RE and the measurement temperature, meaning that it is important to experimentally evaluate the roles of RE ions for each material. The easy axes for RE-doped Bi2212 corresponded well with the result for RE123, whereas those for RE-doped BiCa222 were different, particularly in the case of doping of a light RE. This is due to the intrinsic difference in the easy axes and magnetic anisotropy among these RE-free compounds. As suggested from the RE-free, Y and La-doped samples, the easy axes for the CuO2 and CoO2 planes were the c-axis and an axis in the ab-plane, respectively. Therefore, for the doping of a light RE with small magnetic anisotropy, such as Pr or Nd, these c-axis magnetic anisotropies are insufficient to convert the easy axis of BiCa222 to the c-axis. On the other hand, in the case of a heavy RE, such as Tb, Dy or Ho, with c-axis magnetic anisotropy much larger than those of the light RE, even 5 mol% RE substitution can convert the easy axis effectively.
Table 1 Second-order Stevens factor (αJ) and the easy axes for RE123, RE-doped Bi2212 and RE-doped BiCa222.
Triaxial orientation of Y247 by a modulated oval magnetic field
Polycrystalline Y247 was synthesized by a solid-state reaction under high oxygen pressure. Details of the synthesis process are described elsewhere [Citation9]. Y247 powder with particles of mean diameter 6 μ m was mixed with epoxy resin, and this mixture was cured for 8 h at room temperature in a modulated oval magnetic field. The weight ratio of the Y247 powder and epoxy resin is 1:10. Triaxial orientation by the modulated oval field was performed by the repetition of static and rotation modes for 8 h. Details are as follows: in the rotation mode, the mixture was horizontally rotated at a rotation speed of 50 rpm for 15 min in a transverse field (Ha) of 12 T. Then, the rotation was stopped for 15 min and the sample stage was located such that the direction normal to the α-plane (see inset of figure ) overlapped with the Ha direction in each static mode.
Figure 5 XRD patterns of the α-, β-, γ- and δ- planes of the magnetically oriented Y247 powder in the modulated oval field.
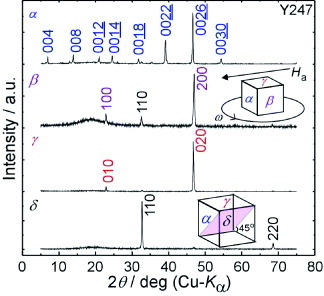
Figure shows XRD patterns of the α-, β- and γ-planes (see inset) for the Y247 powders magnetically oriented using the modulated oval field, together with the pattern taken for the δ-plane, which is perpendicular to the α-plane and tilted at 45° relative to the β- and γ-planes. For the α-plane, only the (00 l) peaks were observed, and the easy axis of magnetization was the c-axis. This is consistent with the result obtained for magnetic grain orientation using a static field of 12 T. When one focuses on the XRD patterns of the β- and γ-planes, the positions of reflection peaks, which are based on (h00) or (0k0), were very close for both planes. However, a slight difference of approximately 0.3° was observed between the (200) and (020) peak positions. The difference coincided well with that obtained from a simulation by the Rietveld method [Citation10]. That is, the main peaks at the β- and γ-planes were derived from the (020) and (200) reflections, respectively. The magnetic susceptibilities for the three crystallographic axes was related as χ c> χ a> χ b. Even in the Y247 system without the twin microstructure and with small paramagnetic anisotropy, the modulated oval field aligned all the crystallographic axes, namely, triaxial grain orientation was achieved.
To evaluate the degree of in-plane orientation of thus obtained Y247, a pole graph was recorded. Figure shows a contour mapping of the pole figure of the (0113) peak. Twofold symmetric reflection spots were clearly observed, confirming the achievement of triaxial orientation. The full-width at half-maximum of these spots was 2.4° for the b∗-direction, indicating that the degree of in-plane orientation was 2.4°. From a practical point of view, a degree of in-plane orientation of within 3° is superior to those for the a-axis growth region of the melt-solidified bulks of RE123 (5–10°) [Citation11] and RE123 films grown on metal tape with several buffer layers (∼4°) [Citation12]. Therefore, one can recognize that this magnetic orientation technique using the modulated oval field is a novel triaxial orientation process for the production of high-Tc superconductor materials.
Figure 6 Contour pole figure mapping of the (0113) peak for magnetically oriented Y247 powder in the modulated oval field.
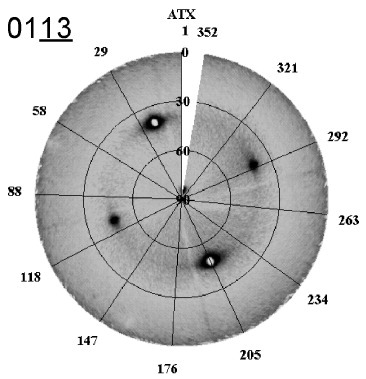
A serious problem in the above grain-orientation technique at the current stage is that the excellent triaxial orientation was achieved in epoxy resin with high viscosity. Therefore, to improve practicality of this process, the fabrication of quasi-single-crystalline bulk samples and thick films is required. Also it is indispensable to use a colloidal solution [Citation13–15] containing Y247 powder, in which the viscosity is much smaller. This is a serious practical problem to overcome, and, in practice, the use of other modulated oval field techniques, the choice of appropriate RE ions [Citation5] in RE247 and the optimization of grain orientation conditions will be required. Furthermore, if the grain orientation technique using the modulated oval field can be applied to the RE123 phase, there is a strong possibility that this magneto-scientific technique will become a practical production method for bulk magnets and superconducting cables. Of course, one should take into account that a twin microstructure is formed in the general sintering of RE123 and development a method suppressing the disappearance of the in-plane magnetic anisotropy induced by the twin microstructure.
Conclusions
The easy axes of magnetization and magnetic anisotropies at room temperature of the magnetically oriented powders of RE123, RE-doped Bi2212 and RE-doped BiCa222 depend on the type of RE ion. The magnetic role of each RE ion was qualitatively almost the same for the three compounds, and the magnetic anisotropies generated by heavy RE ions were much larger than those generated by light RE ions. The appropriate choice of RE dopant leads to not only a change in the direction of the easy axis but also a minimization of the magnetic field required for grain orientation. Furthermore, we demonstrated the triaxial orientation of Y247 without the twin microstructure in epoxy resin using a modulated oval field. According to the pole figure of the (0113) peak, a high degree of in-plane orientation of less than 3° was achieved, which was smaller than that of coated RE123 conductors. Therefore, there is a strong possibility that a magnetic triaxial orientation technique will be used in the production of superconducting bulks and thick films if a grain orientation technique based on a colloidal process is developed.
Acknowledgments
This work was partly supported by Grants-In-Aid for Scientific Research (20900114, 18760051) and the Global COE Program from MEXT, Japan and the Murata Science Foundation.
References
- KimuraTKimuraFYoshinoM 2006 Langmuir 22 3464 http://dx.doi.org/10.1021/la053479n
- DimosDChaudhariPManhartJ 1990 Phys. Rev. B 41 4038 http://dx.doi.org/10.1103/PhysRevB.41.4038
- KimuraT 2003 Polym. J. 35 823 http://dx.doi.org/10.1295/polymj.35.823
- KimuraFKimuraT 2008 Sci. Technol. Adv. Mater. 9 024212 http://dx.doi.org/10.1088/1468-6996/9/2/024212
- IshiharaAHoriiSUchikoshiTSuzukiT SSakkaYOginoHShimoyamaJKishioK 2008 Appl. Phys. Express 1 031701 http://dx.doi.org/10.1143/APEX.1.031701
- FerreiraJ MMapleM BZhouHHakeR RLeeB WSeamanC LKuricM VGuertinR P 1988 Appl. Phys. A 47 105 http://dx.doi.org/10.1007/BF00619708
- LivingstonJ DHartH RWolfW P 1988 J. Appl. Phys. 64 5806 http://dx.doi.org/10.1063/1.342211
- ChikazumiSTajimaKToyamaK 1971 J. Physique. Coll. 32 C1
- FukushimaTHoriiSOginoHUchikoshiTSuzukiT SSakkaYIshiharaAShimoyamaJKishioK 2008 Appl. Phys. Express 1 111701 http://dx.doi.org/10.1143/APEX.1.111701
- IzumiFIkedaT 2000 Mater. Sci. Forum 321–324 198
- NakashimaTShimoyamaJTazakiYIshiiYHoriiSKishioK 2006 IEEE Trans. Appl. Supercond. 16 1047 http://dx.doi.org/10.1109/TASC.2005.864360
- IijimaYKanekoNHanyuSSutohYKakimotoKAjimuraSSaitoT 2006 Physica C 445–448 509 http://dx.doi.org/10.1016/j.physc.2006.04.045
- HoriiS et al 2003 Japan. J. Appl. Phys. 42 7018 http://dx.doi.org/10.1143/JJAP.42.7018
- OkamotoTHoriiSUchikoshiTSuzukiT SSakkaYFunahashiRAndoNSakuraiMShimoyamaJKishioK 2006 Appl. Phys. Lett. 89 081912 http://dx.doi.org/10.1063/1.2338749
- SuzukiT SUchikoshiTSakkaY 2006 Sci. Technol. Adv. Mater. 9 356 http://dx.doi.org/10.1016/j.stam.2006.01.014