Abstract
This review introduces and summarizes lyotropic liquid crystal (LLC) directed syntheses of nanostructured materials consisting of porous nanostructures and zero-dimensional (0-D), one-dimensional (1-D) and two-dimensional (2-D) nanostructures. After a brief introduction to the liquid crystals, the LLCs used to prepare mesoporous materials are discussed; in particular, recent advances in controlling mesostructures are summarized. The LLC templates directing the syntheses of nanoparticles, nanorods, nanowires and nanoplates are also presented. Finally, future development in this field is discussed.
Introduction
Nanostructured materials exhibit size and shape dependent properties and have potential applications in various fields, such as materials science, biomedical science, electronics, optics, magnetism, energy storage, electrochemistry and so on [Citation1–3]. In the past decades, many methods have been developed to synthesize nanostructured materials with controlled size, shape, dimensionality and structure [Citation4, Citation5]. Among the strategies to different nanostructures from lithographic techniques to chemical methods [Citation6, Citation7], the template method is known as a useful route to prepare porous organic polymers and various inorganic nanomaterials with different morphologies [Citation8, Citation9], such as spherical particles, hollow nanostructures, one-dimensional (1-D) nanowires, nanorods and nanotubes, and two-dimensional (2-D) ordered arrays. It is obvious that the most attractive feature of template-directed synthesis is its versatility for the transfer of structures from templates to desired materials.
The template synthesis was firstly explored by Martin [Citation10, Citation11]. He introduced membrane-based syntheses, by which the desired materials were prepared within the pores of a nanoporous membrane called ‘template’. The templates are divided into hard templates and soft templates. Hard templates usually include silica [Citation12], anodic aluminum oxide [Citation13–15] and mesoporous carbon [Citation16–20]. The soft templates have received more attention over the last decade for the generation of nanostructured materials [Citation21, Citation22], because they are more versatile and therefore more advantageous than hard templates. The soft templates are composed of soft compounds such as biomolecules [Citation23], polymer gels [Citation24, Citation25], block-copolymers [Citation26, Citation27], fibers [Citation28] and emulsions [Citation29, Citation30]. The lyotropic liquid crystal (LLC), whose lattice constants range from several nanometers to tens nanometers, may become perfect template candidate for designing novel materials.
LLC templates, including lamellar, hexagonal and cubic phases, have remarkable effects on controlling the structure and morphology of desired nanomaterials. LLC confines the reactants in the limited dimension; and the structures of long-chain order affect the nucleation and growth processes of the products, which could be applied to control the synthesis of the nanomaterials with desired morphologies. The LLC templates, as now referred to, confine the structure of nanomaterials mainly depending on the interaction between the inorganic ions and surfactants within the template. So the final morphology and morphology depended properties of the desired materials can be controlled just by changing the composition and concentration of LLC. Herein, we provide an overview of the LLC directed synthesis of inorganic nanostructures. First, a brief description is given of LC and the synthesis of porous nanostructures using LLC as templates. Then the LLC directed synthesis of other kinds of nanostructures is reviewed, such as 1-D nanowires, rods, tubes and 2-D nanoplates. Finally, a conclusion and outlook on this subject are given.
Liquid crystals (LCs)
LCs have been widely used in displays, sensors, drug delivery vehicles, photonic band gap structures, as well as in optical elements such as controllable lenses and lasers [Citation31, Citation32]. LC is anisotropic fluid, combining the flow property of ordinary liquid with the anisotropic physical parameters of crystals. They represent thermodynamically stable phases situated between the ordinary isotropic liquid and anisotropic crystalline solid, differing in their degree of order. LC phase exists between the conventional crystal phase and liquid phase, which is considered a fourth state of matter following solid, liquid and gas. It can be divided into thermotropic liquid crystal (TLC) and LLC according to the composition and formation condition. TLCs are usually formed in one compound; they exhibit different phases as a function of temperature and are used in displays. The LLC phases of surfactants show long-range periodicity with a typical period ranging from 2 to 15 nm and exhibit rich polymorphism of structures.
Because this review is limited to LLC-directed synthesis of nanostructured materials, the following discussion will be brief. It is known that in concentrated aqueous solution, the surfactant molecules aggregate into LLC phases instead of micelles. LLCs consist of water and amphiphilic molecules which include hydrophobic and hydrophilic blocks on the nanometer scale. LLC phases which form in the surfactant solution are highly sensitive to the solution conditions, including surfactant concentration, temperature, ionic strength, counterion polarizability, counterion charge and additives or co-surfactants. Some scientists have tried to control the size, shape and dimensionality of nanostructures by modulating the LLC phases [Citation33].
It is reported that LLC can be used as the perfect template for growing nanostructured materials. The advantage of the synthesis in LLC systems is that it is possible to produce the desired materials, whose nanostructures are casts of structures of the LLC phases, by exploiting the rich lyotropic polymorphism. On this basis, Mountziaris’s group successfully synthesized 0-D, 1-D and 2-D semiconducting ZnSe nanostructures using cubic, hexagonal and lamellar LLC as templates, respectively [Citation3Citation4]. The shape and size of the resulting nanostructures could be manipulated by selecting the templating phases of LC.
Although numerous excellent articles and reviews on the template synthesis have been published [Citation35, Citation36], the comprehensive reviews of LLC-directed synthesis are relatively few [Citation37, Citation38]. In 2007, Hegmann et al gave a short overview of current research in LC nanoscience [Citation39]. It highlighted the particular role of LC materials during the synthesis of nanostructures with uniform size and shape, the use of condensed LC phases for the assembly of nanoparticles, defect formation in LC-nanoparticles suspension and potential applications of colloidal LC suspensions. Most recently, Yamauchi and Kuroda have reviewed the synthesis and microstructure of mesoporous metals and described several advantages of the soft-template approach by comparing it with the hard-template approach [Citation40]. However, systematic summaries of the LLC templating synthesis of nanostructured materials and the challenges and opportunities are still rare. Here, we summarize publications on LLC-directed synthesis of inorganic nanostructured materials in two main aspects: (i) porous nanostructures and (ii) other kinds of nanostructures (0-D nanoparticles, 1-D nanowires and ribbons and 2-D nanoplates). We focus on the preparation and formation mechanism and give a brief discussion at the end of each section.
Porous nanostructures
Among the diverse techniques of surfactant-assisted synthesis of mesoporous materials, direct templating of one LLC phase was proved a very versatile approach with many advantages. Considering these advantages, one might call these systems the ‘second generation’ of mesoporous materials [Citation41]. The concept of true LLC template or nanocast [Citation42] is currently widely used in the synthesis of porous media used in catalysis or adsorption areas. This process for the production of porous materials has remarkable characteristics. First, the as-obtained materials have uniform pore size and morphology. Second, the pore size can be adjusted by changing the composition of LC.
Silica-based mesoporous materials
Microporous and mesoporous inorganic materials have been widely used as catalysts and sorption media for their large surface areas. In 1990, Kato synthesized porous SiO2 with controlled pore size by the reaction between the layered polysilicate kanemite and Cn-TMA surfactants [Citation43]. Then in 1992, the preparation of ordered mesoporous silicates (MCM-41) based on the LC-template mechanism was reported [Citation44]. The pore diameter could be varied by changing the chain length of the cationic surfactant. The diffraction and microscopy results were similar to those from the LLC phase reported by Ekwall [Citation45, Citation46], which was produced in surfactant–water mixture; these similarities implied the presence of LC template. In this LLC template mechanism (figure ), the inorganic material occupies the continuous solvent (water) regions to create inorganic walls between the surfactant cylinders. The stable mesoporous molecular sieve is produced after removal of the organic template by calcination.
Figure 1 Schematic drawing of the LC templating synthesis of mesoporous materials. (Reprinted with permission from [Citation44], Nature Publishing Ltd ©1992.)
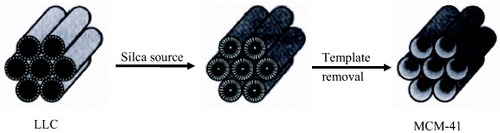
Then the same group further explained the LC template mechanism in detail [Citation47]. In order to support the LC template mechanism, it was demonstrated that the structures and pore dimensions of mesoporous materials were intimately linked to the properties of the surfactants, such as surfactant chain length and solution chemistry. There might be two possible mechanistic pathways for the formation of MCM-41. In the first case, the surfactants aggregated into rods which existed in a hexagonal arrangement in solution [Citation48]. The silicate in the reaction mixture was then precipitated around these hexagonal micelle arrays to produce the inorganic structure. In another case, the silicate affected the formation of LC phase. The desired MCM-41 materials, obtained from the resultant silicate, would encase hexagonal structure in these two cases. LC template synthesis was widely applied to prepare a variety of mesoporous materials. In 1995, it was reported that each of the three LC phases exhibited by the nonionic surfactants could be used to direct the synthesis of silica with a nanostructure that was a direct cast of the supramolecular architecture of LLC [Citation42]. Coleman and Attard reported the template synthesis of silica mesostructures with pore size of 3 nm from the ordered LC [Citation49]. The previous synthesis used low surfactant concentrations and the template mechanism, which was thought a cooperative process involving the interaction of inorganic ions with discrete surfactant aggregates [Citation50–53]. Furthermore, it is suggested that the LC phases, not the micelle aggregates, directed the formation of the mesoporous materials due to the absence of regular mesostructures in materials prepared with low concentration of nonionic surfactants [Citation54]. The preparation of mesoporous silica from LC template affords the remarkable advantages over the other routes. It allows precise control over the structure of the resulting inorganic solid due to the knowledge of LC structures. Since the hydrothermal process is not necessary for the preparation of mesoporous silicates, the phase behavior of reaction can be monitored at any time before polycondensation. More importantly, this approach allows the incorporation of aluminum into the framework.
In addition, many types of silica-based mesoporous materials have been successfully synthesized through the LLC templating process in polymers or oligomer surfactant systems [Citation55]. Göltner et al described the preparation of mesoporous silica from LLC polymer templates [Citation56]. These LLC templates can be used for the synthesis of a broader range of nanostructured solids than previously demonstrated. It is anticipated that the synthesis of mesoscopically ordered sulfides and oxides other than silicates should also be possible.
Mesoporous metals and alloys
Beside the silica-based mesoporous materials, mesoporous metals and alloys hold promise for a wide range of potential applications. Controlling the structure of metals at mesoscale (2–50 nm) is crucial to improve their performance as the fuel cell electrodes, and it may also assist in the miniaturization of optical and electronic devices for data transmission, storage and computation [Citation57].
The synthesis of ordered mesoporous metals and their mesoporous composites still remains a challenge because metals have high surface energies that favor low surface areas. The mesoporous metals have advantages over the classical metal colloids with respect to the stability and handling. The high surface area is advantageous in catalytic applications. The combination of high surface area with tailed architecture, consisting of regular pores and a continuous electron conduction pathway, would be favorable in fuel cells, batteries or sensors. Traditionally, mesoporous metals have been produced by use of mesoporous silica as a hard template.
The generation of nanostructures using LLC mesophases is not only restricted to the silicate, but also suitable for the preparation of ordered, porous metal and alloy nanostructures. In 1997, Attard’s group firstly reported that the mesoporous Pt could be produced by the chemical reduction of metal salts dissolved in the aqueous domains of a hexagonal LLC phase. In particular, they showed that the reduction of platinum salts in this system led to Pt whose nanostructure was a cast of LLC phase architecture. Such granular nanostructured metals could be directly used in catalysis, but they were of limited relevance for the electrochemical or sensor devices in which the metal was required as a thin film [Citation58].
The ability to predetermine the film’s nanostructure through different LLC phases will provide new method to obtain the optimal nanostructures. The mesoporous Pt film with a controlled nanostructure can be easily electrodeposited from LLC plating mixtures. For the film, the geometric disposition of the channels is determined by the architecture of the LC phase, and the diameters of the pores are determined by the length of the alkyl chain of the surfactant used in the plating mixture. It is shown that the electrodeposition of metals from appropriate salts, dissolved in the aqueous domains of the LLC phases of nonionic surfactants, produces metal films with defined porous nanostructures, high specific surface areas, electrical connectivity, fast electrolyte, and good mechanical and electrochemical stability [Citation42]. Moreover, Attard’s group prepared a variety of nanostructured materials through this LC template method, including mesoporous tin films [Citation59], mesoporous Ni/Ni oxide [Citation60], nanostructured Pd/Pt films [Citation61], nanostructured Pt films [Citation62] and mesoporous Pt/Ru alloy [Citation63].
It is proved that electrodeposition of metals from the H1 hexagonal phase of nonionic LLC phases can produce films containing regular arrays of uniform pores whose dimensions are determined by those of the micelles in the LLC phase. Bartlett and Simon extended this idea and demonstrated that they could electrochemically deposit the mesoporous films of two metals, one on top of the other, by which they produced a single nanostructure with the pores running continuously through the two metal layers [Citation64]. Figure is the schematic representation of the templating process. Firstly, the surfactant molecules assemble into the cylindrical micelles which pack into a hexagonal LLC phase, which is then applied to direct the electrodeposition of metal films. The metal salt and electrolyte are dissolved into the aqueous component of the mixture, and the metal nanoparticles begin to deposit from the electrode surface around the surfactant micelles. Once deposition is finished, the surfactant is removed by washing to leave the metal film with a regular hexagonal array of uniform pores. The thickness of the film could be directly controlled by the total charge passed.
Figure 2 Schematic representation of the templating process used to deposit the H1−e metal films. The cylinders represent the micellar rods in the LLC phase. (Reprinted with permission from [Citation64], American Chemical Society © 2003.)

Although the metals from some groups of elements are readily synthesized by this template method (such as groups 9 and 10), few successful attempts are reported for other groups (e.g. groups 11 and 12). In addition, only a limited number of surfactants were investigated. So it is important to find the guidelines for selecting the metal precursors, the surfactants and other free parameters (concentration, temperature and potential of electrodeposition, electrode material and geometry, etc) among the vast number of possible LC templating recipes. LC templating procedures for the metals from groups 11 (Cu, Ag, Au) and 12 (Zn, Cd), and other metals (Ni and Sn) were included for comparison [Citation65]. Table 1 shows the investigated metal/surfactant combinations.
Table 1 The investigated metal/surfactant combinations and as-obtained structures. (Reprinted with permission from [Citation65], Wiley-VCH Verlag GmbH & Co. KGaA © 2006.)
In summary, transmission electron microscopy (TEM) images showed that Cd, Ni, Sn and Cu had mesoporous structures, and the structures of Cd, Ni and Sn were stable, whereas in the case of Cu, the structure corroded and collapsed rapidly. In contrast, no evidence of mesostructures was found for Ag, Au and Zn. Au and Ag may tend to precipitate with some surfactants, and the interaction between the metal and the surfactant may be too weak to prevent displacement of the surfactant rods during film growth. Besides, Zn film without mesoporous structure could be obtained due to the fairly high formation rate.
The particular templating mixtures, in which a stable mesoporous metal film is formed, are summarized in table 1. The first condition is the formation of a regular LC phase. Despite the necessary formation of the regular LC phase, the process of the film growth and the lattice formation are also crucial. Lattice formation should be slow and controlled. The Zn film was not mesoporous because the phase separation occurred as a result of the fairly high formation rate. Third, the inorganic species must have a tendency to condense to the extended frameworks. For both Ag and Au, it might well be that their interactions with the surfactants were too weak to form the extended frameworks. Finally, the mesoporous structure had to retain after the surfactant removal. In order to prevent phase separation, the inorganic species must not form a stable crystalline phase with high lattice energy. In addition, in order to avoid the collapse during surfactant removal, the film formation must still result in a stable framework and the interaction between surfactant and framework must not be too strong. For the nonionic surfactants, the extraction process was the best way to remove the surfactant while preserving the mesoporous structure. Otherwise, the thickness of the walls between the pores and the redox chemistry of deposited metal were also important in preserving the mesoporous structure. If the metal was oxidized upon surfactant removal, the structure might collapse [Citation54, Citation66]. Thus systematic knowledge is established about whether the metal, available in the formation of mesoporous electrodes for electrochemical and electroanalytical method, can be templated using LLC.
It is obvious that this approach creates a novel avenue for the production of mesoporous metals or related alloys. In principle, the LLC template method is applicable to a wide variety of metals through the electrochemical process because the approach involves just a simple replication of well-ordered LLC. Therefore, several additional examples have been reported for Ni/Co alloys [Citation67], 3-D nanoporous Pt film [Citation68], Co [Citation69], Pd [Citation70] and Rh [Citation71]. In a word, mesostructured metal is typically achieved by reducing a suitable precursor dissolved in the aqueous domain of LLC phase. Removal of the surfactant affords the material with a porous nanostructure, which is a direct cast of the architecture of LC phase in which it is formed.
Mesoporous semiconductors
Although the electrodeposition from LC phases is an efficient route to the adherent films of nanostructured metals, its use in the production of semiconducting films is not immediately obvious. The ability to electrodeposit semiconducting films with well-defined mesoporous architectures is of importance for understanding the fundamental physics of low dimensional structures and, potentially, for applications in sensors, optical devices and solar cells. Attard’s group prepared highly ordered mesoporous materials, such as inorganic oxides and metals, using LLC phases of polyoxyethylene surfactants [Citation42, Citation57–59, Citation62]. This novel approach to nanostructured semiconductors will provide the unique system in which the relationship between nanoarchitecture and quantum size effect could be explored. In 2001, they reported the electrodeposition of mesoporous Se film from the LC phase [Citation72]. It is known that solid Se has four major allotropic modifications, a vitreous, two monoclinic and a hexagonal (so-called metallic) phase. The hexagonal Se is a semiconductor due to the ordered arrangement of Se chains facilitating electronic conduction. Beside careful control of electrodeposition conditions (e.g. temperature and current density) and careful selection of substrate material, the LC plating mixture also requires significant modifications, because the surfactant limits the temperature range and deposition potential as well as the composition and concentration of the components. The successful production of semiconducting Se films suggested that the LC template could be employed in the production of other elemental semiconductors (e.g. nanostructured Te films [Citation73]) as well as II–VI semiconductors (e.g. CdSe, CdTe films [Citation74, Citation75]) and related materials (e.g. PbSe). The pore diameters and the topology of the films can be controlled by adjusting the experimental parameters.
Another interesting work was preparation of mesoporous ZnS hollow microspheres [Citation76] using double direct templating methods that combined the hexagonal LLC template with the colloidal template. The hexagonal LLC was applied as a soft template for the mesostructure and the colloidal template for the hollow morphology. On the basis of the experimental results, the formation process of mesoporous hollow ZnS microspheres is illustrated with figure . In the initial stage, upon mixing the reactants, a hexagonal LLC forms. This hexagonal phase plays dual roles including defining the formation of ZnS spatially and preventing aggregation of the colloids. The LLC directs the mineralization of ZnS on the surface of a silica or polystyrene colloidal template. Removal of the templates results in a periodically mesoporous ZnS hollow capsule. It is speculated that this approach for patterning the surface of a colloid in the nanometer scale might be a starting point for obtaining novel ‘patchy’ microspheres with interesting self-assembly.
Figure 3 Schematic formation process of the mesoporous hollow microspheres. (Reprinted with permission from [Citation76], American Chemical Society © 2005.)
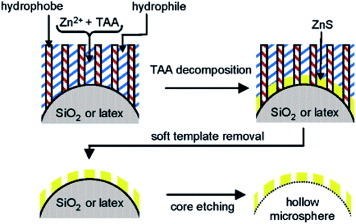
Since Attard’s first introduction of the synthesis of mesoporous silica using the LC phase template, this approach has been applied to synthesize different mesoporous materials. LC phase was achieved using the lyotropic property of the oligo (ethylene oxide) in water and with a low concentration of the additives. For most of them, it was established that the metal salt concentration must be low to maintain the LC phase during further processing of the mixture. As an exception, Dag et al utilized a lyotropic lithium triflate-silicate liquid as a supramolecular template for a ‘one-pot’ synthesis of a novel ionically conducting nanocomposite material [Citation77]. A new LC mesophase was discovered, that consisted of transition metal salt and nonionic surfactants or pluronics at high metal salt concentrations, which were different from the conventional surfactant LLC [Citation78]. Afterwards, they investigated many new salts of binary systems of two different nonionic surfactants [Citation79] and prepared the mesostructured CdS (meso-CdS) materials through the reaction between H2S and cadmium nitrate in C12EO10LLC system [Citation80]. In addition, the effect of ions on the LC mesophase [Citation81] and phase separation in LC mesophase [Citation82] of different transition metal salt of surfactants was investigated. Recently, they discovered another LLC phase formed by the mixture of two surfactants with [Zn(H2O)6](NO3)2 in the presence of a minimum concentration H2O [Citation83]. Subsequently, the LC mesophases formed by transition metal salts and pluronic copolymer were applied as a template to produce mesostructured binary and ternary metal sulfide films [Citation84].
The LLC phases can be used as versatile nanoscale templates for the production of inorganic materials with high specific surface areas and uniform pore structures. The nanoarchitecture of the pores and pore dimensions can be precisely controlled through the suitable choice of surfactants with the hydrophobic additives as the swelling agents. In addition, the range of pore architectures accessible through the electrodeposition process is extensive and only limited by the LLC polymorphism of the chosen surfactant/water system.
LLC template synthesis of mesostructured materials has attracted much attention over the past decade. Yamauchi et al reviewed recent developments in the preparation of mesoporous metals and related alloys. They discussed the evolution and microstructure of mesoporous metals from LLCs, as well as potential applications of mesoporous metals produced with LLC template [Citation40]. In this review, beside the fabrication and formation process of mesoporous metals and alloys, the silica-based mesoporous materials and mesoporous semiconductor are also discussed in detail. The synthesis is more difficult for mesoporous metals/alloys and chalcogenides than for silicates. Silica-based oxides have been widely investigated, but there are only few reports on LLC-template synthesis of other mesoporous oxides.
In addition, the size and structure of the resulting materials can be easily controlled by choosing an appropriate template. The LLC templating synthesis of mesoporous materials is a real cast process, so the exploration of new LLC is of much importance for the preparation of new types of mesoporous inorganic materials. The compositions of currently used LLCs are relatively single, the large block copolymers might also be applied to design novel LLC mesophases with large mesopores. The novel LC mesophases developed by Dag et al, that consist of transition metal salts and non-ionic surfactants or pluronics, may be used in templating synthesis of new inorganic mesostructures, although only mesostructured binary and ternary metal sulfide films have been produced by this new kind of templates. Fabrication of novel nanostructures through developing new kinds of LLCs is still an interesting research field.
Other nanostructures
Although LLC-template syntheses of porous nanostructures have been extensively investigated, few studies focused on the preparation of other kinds of nanostructures. The successful examples of 0-D, 1-D and 2-D nanostructures are summarized as follows.
Nanoparticles
Stabilized clusters and colloids of Pd with nanometer-scale dimensions are of particular interest as catalyst for organic and inorganic reactions [Citation85]. In order to prevent aggregation and absorb the stabilizers on the nanoparticles, an alternative method for generating stabilized metal nanoparticles was established through synthesizing them in or on nanoporous supports. A new type of Pd nanoparticle composite was prepared by ion exchange in the hexagonal LLC phases and subsequent reduction. The resulting composite contained Pd particles with size ranging from 4 to 7 nm and exhibited high catalytic activity. Although this new template approach was initially demonstrated with Pd, the concept could be applied to other transition metals or lanthanides to produce the nanostructured metal ion composites and nanoparticles such as Cu2+ and Zn2+ nanostructured polymers and composites.
The synthesis of metal sulfide and selenide quantum dots and their assembly into ordered structures have been widely studied due to their technologically importance. However, the emphasis in the past has been put on preparing nanocrystals with narrow size distributions, not superlattice structures. In the previous studies, only oblong or cubic crystallites or mesoporous reticulated structures have been obtained in preformed LCs. Braun’s group reported the first successful template synthesis of periodic nanostructured inorganic materials which copied directly the symmetry and dimensionality of the organic precursor. As early as in 1996, Braun described the growth of stable semiconductor-organic superlattices based on CdS and CdSe templates in the LC phase from nonionic organic amphiphilic molecules, water and precursor ions for the inorganic semiconductor [Citation86]. They attributed the superlattice morphology of nanocomposite to a templating effect of the hexagonal mesophase. Organic–inorganic composite materials have novel properties and structures significantly enhanced over those of either the inorganic or organic phase alone. It is anticipated that these materials can be tailored through the electronic properties of organic amphiphiles for photosynthetic and photocatalytic applications.
Subsequently, various nanoscale semiconducting superlattices including CdS, CdSe and ZnS have been generated by direct templating in LLC [Citation87]. The semiconductor growth process copies the symmetries and characteristic dimensions of LLC by avoiding the growth of inorganic compositions within regularly spaced hydrophobic regions. The morphology of features in the nanostructured solids and their dimensions could be controlled through the amphiphilic molecular structure and water content in LC. When these materials including Ag2S, CuS, HgS and PbS grow in LLC, they do not contain any features commensurate with the symmetries and dimensions of the precursor LC. It is proposed that the interaction between the polar segments in template molecules and the precipitated inorganic compositions and precursor ions is necessary for direct templating. Mineralization in LLC results in the formation of previously unattainable CdS nanostructures. Growth of CdS was studied in three different LC phases [Citation88]. Semiconductor growth in a hexagonal LC yielded a nanostructure with hexagonal symmetry, a lamellar LC yielded thin sheets, and a cubic LC (consisting of spherical micelles) yielded hollow spheres. All the three nanostructures appeared to be linked to the LC matrix in which they grow. The ability to define the structure of hard inorganic materials, such as a semiconductor with soft organic structures at room temperature, provides a new methodology for the formation of interesting nanostructures, which will produce new properties.
In addition, Bi [Citation41], β-FeOOH [Citation89], Ag [Citation90], PbS [Citation41, Citation91] and BiOCl [Citation92] nanoparticles have been successfully synthesized with this method.
1-D nanostructures
1-D nanostructures such as nanowires, nanorods and nanotubes are currently the focus of much attention because of their special properties compared with the 0-D quantum dots. It is quite noteworthy that a ‘soft’ LC phase could direct the formation of 1-D inorganic nanostructures.
The LC template synthesis of group IIB-VI semiconductor nanowires received much attention for the special properties of the products. Ordered ZnS nanowires with a diameter of about 5 nm were obtained using hexagonal LLC under γ-irradiation [Citation93]. Most importantly, several close-packed nanowires aggregated to form the bundles which duplicated the hexagonal structure of close-packed inverted micelles formed by amphiphilic molecules. Besides, CdS nanowires [Citation94] and uniform ZnS nanorods [Citation95] were also successfully prepared using the LLC template method.
Yan’s group demonstrated for the first time the fabrication of crystalline Ag nanowire arrays with a high aspect ratio by the electrodeposition from a reverse hexagonal LC phase containing 1-D aqueous channels [Citation96]. It was proved that a high electric field during electrodeposition helped to form the reverse hexagonal LC phase, which was believed essential for the production of crystalline metal nanowires with high aspect ratio. Figure illustrates possible improved alignment of LC phase during electrodeposition. This process combines the formation of a soft LC phase with electrodeposition, which is fast, reproducible, inexpensive and versatile for the fabrication of metal nanowire arrays with high density and large aspect ratio. In addition, the Ag ribbons consisting of close-packed Ag nanoparticles were prepared in lamellar LLCs [Citation97]. Yan et al demonstrated that Cu2O nanowires (figures and ) with high aspect ratio could be readily electrodeposited from reverse hexagonal LLC phases. They also showed that the hexagonal LC with improved alignment played an important role in the formation of nanowires [Citation98].
Figure 4 Schematic of the proposed enhanced alignment of reverse hexagonal liquid-crystalline phase during nanowire electrodeposition. (Reprinted with permission from: [Citation96], Wiley-VCH Verlag GmbH & Co. KGaA © 2002.)
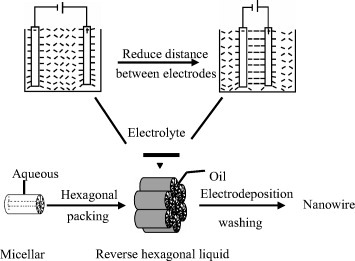
Figure 5 Polarized light micrographs of surfactant AOT systems (×400). AOT/p-xylene/H2O reverse hexagonal liquid crystal before (a) and after (b) doping of Cu2+ ions. (Reprinted with permission from [Citation98], American Chemical Society © 2002.)
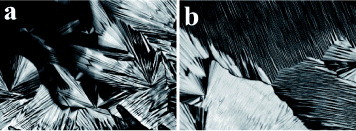
Figure 6 SEM images of cuprite nanowires electrodeposited from reverse hexagonal LC phase AOT (1.5 M)/p-xylene/CuCl2 (0.1 M) at deposition potential of −1.1 V with electrode distance of 0.5 mm for different deposition times: (a) 1 h, (b) 2 h and (c) 2 h, at different magnifications. (Reprinted with permission from [Citation98], American Chemical Society © 2002.)
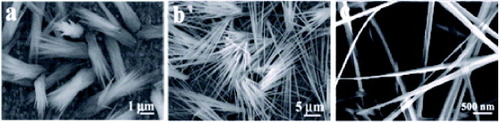
A single cationic, anionic or nonionic surfactant was used as templating agent in most of the previous syntheses. Kijima’s group reported the growth of AgBr and SnO2 microwires using mixed surfactant nematic phases as a reaction medium for the first time [Citation99]. The same group reported that the reduction of metal salts, confined to lyotropic mixed surfactant LCs, resulted in the first synthesis of Pt, Pd and Ag nanotubes of 6–7 nm outer diameter. The structure model for the precursory LC and formation mechanism are illustrated in figure . The present Pt nanotubes are formed by a mixed surfactant templating mechanism. The rod like micelles in an aqueous medium are combined with much larger surfactant molecules to form an aqueous solution of the mixed surfactant cylindrical micelles as described above. On cooling the mixed micellar solution converts into a hexagonal LC. Finally, the Pt species confining to the aqueous region of the molecular assemblies, are reduced to platinum atoms. The mixed surfactant LC template method can be extended to the synthesis of a wide range of 1-D inorganic or organic materials [Citation100].
Figure 7 Schematic models for the formation of Pt nanotubes in the mixed surfactant templating system: (a) Mixed (C12EO9/Tween 60) and (b) single (C12EO9) surfactant cylindrical rod like micelles. (c) Pathway from micellar solution to metal nanotubes by the reduction of metals salts confined to the aqueous shell of mixed-surfactant cylindrical micelles. The metal salts and water molecules are omitted from the models. (Reprinted with permission from [Citation100], Wiley-VCH Verlag GmbH & Co. KGaA © 2004.)
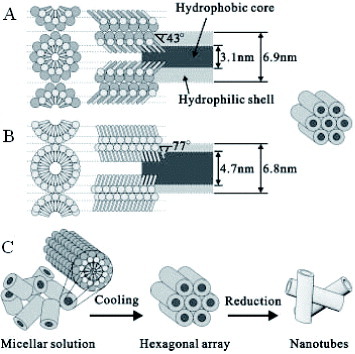
2-D nanostructures
Beside the successful synthesis of 1-D nanostructured materials [Citation99, Citation100], Kijima’s group also prepared different Pt nanosheets in single or mixed surfactant LLC. In their report, growth of metal particles on the LLC, adsorbed at the solid/solution interface, would be greatly advantageous not only for elucidating the formation mechanism of metal nanotubes but also for producing unknown nanostructured metals. Early in 2005, they described a new methodology to fabricate single-crystalline Pt nanosheets by the chemical reduction of a Pt salt based on the interfacial directed growth of Pt metals within LLC at a graphite/solution interface [Citation101]. Next, the nanogroove-network-structured single-crystalline Pt nanosheets were obtained by the reduction of Na2PtCl6 confined to the mixed LLCs using NaBH4 as the reductant. The nanosheets were composed of pseudo 2-D irregular Pt networks, in which Pt nanoleaves were loosely interconnected with crystallographic alignment to form an irregular network of nanogrooves [Citation102]. Recently, Kijima et al synthesized nanohole-structured single-crystalline Pt nanosheets by the borohydride reduction of Na2PtCl6 confined to the LLCs [Citation103]. All these results suggest that the hexagonal LLCs consisting of triple-PEO-chain-based surfactant cylindrical micelles are available for the formation of Pt nanosheets with a 2-D nature. The present approach could be extended to prepare a wide range of novel nanostructures of noble metals using various LLC.
In addition to platinum, other noble metals were also reported. 2-D Au nanostructure is a typical example. The synthesis methods of 2-D Au nanostructure mainly include ultraviolet irradiation, photoreduction, microwave-polyol, and chemical reduction in aqueous solution. However, the yield is usually low and the size of the particles is small for these methods. As a comparison, the LLC template synthesis of 2-D Au nanoplates shows great advantages.
Chen’s group established a new and simple method to prepare Au nano- and micro-plates from LLC [Citation104]. Subsequently, they extended their research to obtain products of controllable shape and size [Citation105]. Single-crystalline Au nano- and micro-plates with triangular or hexagonal shapes were synthesized by reducing HAuCl4 in LLC with the addition of a small amount of capping agents. Figure illustrates a possible mechanism for Au nanocrystal growth. First, the Au atoms and/or small clusters are formed in the LLC aqueous domain as the nascent crystal nuclei (I in figure ). The CTAB (cetyl trimethylammonium bromide) molecules with adsorbed Au nuclei have to arrange side by side near the hydrophobic/hydrophilic interface (II in figure ) because CTAB molecules cannot move freely in the solution phase due to the interdigitation of the alkyl chains in the hydrophobic PPO (poly(p-phenylene oxide)) cores inside the cylinders of LLC. The LLC structure directs the formation of Au nuclei in water domains resulting in a long-range ordered arrangement of the nuclei. Next, anisotropic growth proceeds from these nuclei. Although the Au products do not replicate the long-range ordered structure of hexagonal phase, the template effect indeed occurs at the first stage, which is important for the formation of flat product. Anyhow, the LLC phase provides an ideal reaction environment to control the shape of Au particles.
Figure 8 Schematic growth mechanism of Au microplates from P123(45%)–CTAB(1.0%)–HAuCl4 (0.03 M) system: (a) cross section of the hexagonal phase, (b) side view of two adjacent cylinders and (c) growth process. (Reprinted by permission from [Citation105], American Chemical Society © 2005.)
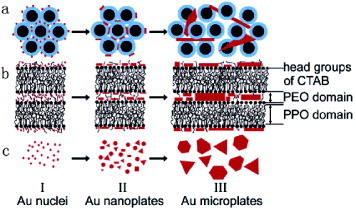
Afterwards, based on the previous study on Au nanoplates, more studies were conducted to better understand the templating and capping effects for synthesizing anisotropic Au nanocrystals. Chen et al fabricated both Au nanoplates with larger sizes and nanobelts in different LLC phases [Citation106]. Also, Au nanoplates mixed with spherical nanoparticles were prepared in lecithin lamellar LCs containing non-ionic surfactants as reductant. The formation of a few nanoplates was attributed to the confinement of the ordered LLC structure and the adsorption of lecithin head-groups on the surfaces of Au particles, which was similar to the results of the hexagonal phase [Citation107]. Recently, the same group also synthesized single-crystalline Au nanoplates in a lamellar LLC. The width of Au nanoplates can be varied from several hundreds nanometers to a few microns by adjusting reaction conditions [Citation108]. The formation analysis indicated that the growth of Au nanoplates was confined in the lamellar structures of LLCs during the spontaneous self-aggregation and growth to large plates, which was found to be crucial to the formation of final plates. All these results suggest an effective way to produce anisotropic Au nanoplates as well as a clue to synthesize other anisotropic noble metal nanocrystals.
LLCs have been successfully used as templates for the synthesis of mesoporous materials, which are called ‘true LLC templates’. Based on the summary of the LLC synthesis of 0-D nanoparticles, 1-D nanowires/nanotubes/nanobelts and 2-D nanostructured materials, it is obvious that the self-organized LC here formed the nanoreactors or directed medium. Here, the formation of the 0-D, 1-D or 2-D nanostructures is mainly due to the confining effect of the LLC mesophases but not a ‘true LLC template’. The morphology of resulting inorganic products is greatly influenced by the geometric properties of the nanoreactors. It is possible to adjust the size, shape and dimensionality of these reactors by controlling the LC phase. Consequently, the size and shape of the inorganic nanoparticles grown inside them can be controlled. For example, the lamellar LC, which had at least one dimension on the nanometer scale, would be applied as an ideal microenvironment for the synthesis of 1-D nanobelts. However, such synthesis is accidental, and the morphology and size of the resultant materials is often unpredictable.
Summary and outlook
The LLC template syntheses of nanomaterials exhibit many incomparable advantages over other methods. A rich variety of nanostructured materials, such as porous oxide, metal/alloy and other 0-D, 1-D, 2-D materials, can now be rationally designed.
In section 3, we overviewed the LLC-template synthesis of mesoporous inorganic materials, including silica-based mesoporous materials, mesoporous metals and alloys and some mesoporous semiconductors. The syntheses of mesoporous metals/alloys and chalcogenides is more difficult than of silicates, but is has been elucidated. There are only few reports on LLC-template synthesis of other mesoporous oxides. Probably other oxide nanomaterials are not as stable as silica-based mesoporous materials. Crystalline oxides might be more susceptible to hydrolysis, redox reactions or phase transformations accompanied with thermal breakdown of the structural integrity, which makes it much more difficult to remove the templates and create porous structures. The other crystalline oxides are chemically, thermally and mechanically more robust. This obstacle may be overcome in the coming years by continued research effort in this rapidly developing field. In addition, the size and structure of the resulting materials can be easily controlled by simply choosing the appropriate template. So the exploration of new LLCs is of much importance for the preparation of new type of mesoporous inorganic materials. The compositions of currently used LLC are relatively single; the large block copolymers may also be used to design novel LLC mesophases with large mesopores.
LLCs have been successfully used as true templates for the synthesis of mesoporous structures with a uniform pore size and shape. As summarized in section 4, LLCs have also been used for the synthesis of 0-D nanoparticles, 1-D nanowires/nanotubes/nanobelts and 2-D nanostructured materials. The LLC phase itself has an increasing use as a nanoreactor or directing agent for the syntheses of nanomaterials with uniform size and shape.
With great progresses being made in LLC-directed synthesis of nanostructured inorganic materials, there are fascinating new opportunities for inorganic and materials chemists, who should remain very active in this field.
Acknowledgments
This work is supported by the National Natural Science Foundation of China (Grant No. 20671057) and Doctoral Foundation of Shandong Province (2007BS04042).
References
- BruchezMMoronneMGinPWeissSAlivisatosA P 1998 Science 281 2013 http://dx.doi.org/10.1126/science.281.5385.2013
- CarusoFCarusoR AMohwaldH 1998 Science 282 1111 http://dx.doi.org/10.1126/science.282.5391.1111
- GleiterH 2000 Acta Mater. 48 1 http://dx.doi.org/10.1016/S1359-6454(99)00285-2
- TrindadeTO’BrienPPickettN L 2001 Chem. Mater. 13 3843 http://dx.doi.org/10.1021/cm000843p
- BradCChenXNarayananREl-SayedM 2005 Chem. Rev. 105 1025 http://dx.doi.org/10.1021/cr030063a
- OzinG A 1992 Adv. Mater. 4 612 http://dx.doi.org/10.1002/adma.19920041003
- AppenzellerT 1991 Science 254 1300 http://dx.doi.org/10.1126/science.254.5036.1300
- HentzeH PAntoniettiM 2001 Curr. Opin. Solid State Mater. Sci. 5 343 http://dx.doi.org/10.1016/S1359-0286(01)00008-0
- TiemannM 2008 Chem. Mater. 20 961 http://dx.doi.org/10.1021/cm702050s
- MartinC R 1994 Science 266 1961 http://dx.doi.org/10.1126/science.266.5193.1961
- MartinC R 1996 Chem. Mater. 14 1739 http://dx.doi.org/10.1021/cm960166s
- GiersigMUngTLizMarzanL MMulvaneyP 1997 Adv. Mater. 9 570 http://dx.doi.org/10.1002/adma.19970090712
- ZhouYHuangJShenCLiH 2002 Mater. Sci. Eng. A 335 260 http://dx.doi.org/10.1016/S0921-5093(01)01938-4
- LiDThompsonS RBergmannGLuJ 2008 Eng. Adv. Mater. 20 4575 http://dx.doi.org/10.1002/adma.200801455
- OrikasaHInokumaNOkuboSKitakamiOKyotaniT 2006 Chem. Mater. 18 1036 http://dx.doi.org/10.1021/cm0521368
- XiaYMokayaR 2005 J. Mater. Chem. 15 3126 http://dx.doi.org/10.1039/b502558c
- LeeJHanSHyeonT 2004 J. Mater. Chem. 14 478 http://dx.doi.org/10.1039/b311541k
- DongARenNTangYWangYZhangYHuaWGaoZ 2003 J. Am. Chem. Soc. 125 4976 http://dx.doi.org/10.1021/ja029964b
- LiWLuAWeidenthalerCSchüthF 2004 Chem. Mater. 16 5676 http://dx.doi.org/10.1021/cm048759n
- SchüthF 2003 Angew. Chem. Int. Ed. 42 3604 http://dx.doi.org/10.1002/anie.200300593
- BommelKFriggeriAShinkaiS 2003 Angew. Chem. Int. Ed. 42 980 http://dx.doi.org/10.1002/anie.200390284
- KishidaMTagoTHatsutaTWakabayashiK 2000 Catal. Lett. 1108
- ZhouHFanTLiXDingJZhangDLiXGaoY 2009 Eur. J. Inorg. Chem. 211 http://dx.doi.org/10.1002/ejic.200800764
- ShchukinD GYaremchenkoAFerreiraMKhartonV 2005 Chem. Mater. 17 5124 http://dx.doi.org/10.1021/cm050672u
- SchattkaJ HShchukinDJiaJAntoniettiMCarusoR 2002 Chem. Mater. 14 5103 http://dx.doi.org/10.1021/cm021238k
- SmarslyBAntoniettiM 2006 Eur. J. Inorg. Chem. 1111 http://dx.doi.org/10.1002/ejic.200501003
- Soler-IlliaG JdeA ACrepaldiE LGrossoDSanchezC 2003 Curr. Opin. Colloid Interface Sci. 8 109 http://dx.doi.org/10.1016/S1359-0294(03)00002-5
- HeJKunitakeTWatanabeT 2005 Chem. Commun. 795 http://dx.doi.org/10.1039/b416316h
- LiWCoppensM 2005 Chem. Mater. 17 2241 http://dx.doi.org/10.1021/cm048486w
- ZhangHWuJZhouLZhangDQiL 2007 Langmuir 23 1107 http://dx.doi.org/10.1021/la062542l
- BahadurB 1992 Liquid Crystals Application and Uses Singapore World Scientific p 4
- DemusDGoodbyJGrayGSpiessHVillV 1998 Handbook of Liquid Crystals Berlin, Germany Wiley-VCH Wenham p 731
- DillingerTBraunP 2004 Chem. Mater. 16 2201 http://dx.doi.org/10.1021/cm0349194
- KaranikolosG NAlexandridisPMalloryRPetrouAMountziarisT J 2005 Nanotechnology 16 2372 http://dx.doi.org/10.1088/0957-4484/16/10/063
- ArigaKHillJ PLeeM VVinuACharvetRAcharyaS 2008 Sci. Technol. Adv. Mater. 9 014109 http://dx.doi.org/10.1088/1468-6996/9/1/014109
- PalmqvistA E C 2003 Curr. Opin. Colloid Interface Sci. 8 145 http://dx.doi.org/10.1016/S1359-0294(03)00020-7
- AntoniettiM 2006 Phil. Trans. R. Soc. A 364 2817 http://dx.doi.org/10.1098/rsta.2006.1857
- YingJ YMehnertC PWongM S 1999 Angew. Chem., Int. Ed. Engl. 38 56 http://dx.doi.org/10.1002/(SICI)1521-3773(19990115)38:1/2<56::AID-ANIE56>3.0.CO;2-E
- HegmannTQiHMarxM 2007 J. Inorg. Organomet. Polym. Mater. 17 483 http://dx.doi.org/10.1007/s10904-007-9140-5
- YamauchiYKurodaK 2008 Chem. Asian J. 3 664 http://dx.doi.org/10.1002/asia.200700350
- GoltnerC GAntoniettiM 1991 Adv. Mater. 9 431 http://dx.doi.org/10.1002/adma.19970090516
- AttardG SGlydeJ CGöltnerC G 1995 Nature 378 366 http://dx.doi.org/10.1038/378366a0
- YanagisawaTShimizuTKurodaKKatoC 1990 Bull. Chem. Soc. Japan 63 988 http://dx.doi.org/10.1246/bcsj.63.988
- KresgeCRothWVartuliJBeckJ 1992 Nature 359 710 http://dx.doi.org/10.1038/359710a0
- EkwallP 1971 Advances in Liquid Crystals vol 1 G HBrown New York Academic
- EkwallPMandellLFontellK 1969 Liquid Crystals G HBrown London Gordon and Breach p 325
- BeckJ SVartuliJ CRothW JLeonowiczM EKresgeC TSchmittK DChuC T WOlsonD HSheppardE W 1992 J. Am. Chem. Soc. 114 10834 http://dx.doi.org/10.1021/ja00053a020
- TiddyG J T 1980 Phys. Rep. 57 1 http://dx.doi.org/10.1016/0370-1573(80)90041-1
- ColemanNAttardG S 2001 Micropor. Mesopor. Mater. 44 73 http://dx.doi.org/10.1016/S1387-1811(01)00170-6
- PostorinoPRicciMSoperKNeilsonG 1993 Nature 366 668 http://dx.doi.org/10.1038/366668a0
- PostorinoPSoperA 1994 J. Chem. Phys. 101 4123 http://dx.doi.org/10.1063/1.467462
- EnderbyJHoweR 1973 Chem. Phys. Lett. 21 109 http://dx.doi.org/10.1016/0009-2614(73)80025-9
- AttardG SEdgarMGöltnerC G 1998 Acta. Mater. 46 751 http://dx.doi.org/10.1016/S1359-6454(97)00256-5
- YangPZhaoDMargoleseD IChmelkaB FStuckyG D 1999 Chem. Mater. 11 2813 http://dx.doi.org/10.1021/cm990185c
- WarrenS CMessinaL CSlaughterL SKampermanMZhouQGrunerS MDisalvoF JWiesnerU 2008 Science 320 1748 http://dx.doi.org/10.1126/science.1159950
- GöltnerCHenkeSWeissenbergerMAntoniettiM 1998 Angew. Chem., Int. Ed. Engl. 37 613 http://dx.doi.org/10.1002/(SICI)1521-3773(19980316)37:5<613::AID-ANIE613>3.0.CO;2-G
- AttardG SCorkerJ MGöltnerC GHenkeSTemplerR H 1997 Angew. Chem., Int. Ed. Engl. 36 1315 http://dx.doi.org/10.1002/anie.199713151
- AttardG SBartlettP NColemanN R BElliottJ MOwenJ RWangJ 1997 Science 278 838 http://dx.doi.org/10.1126/science.278.5339.838
- WhiteheadA HElliottJ MOwenJ RAttardG S 1999 Chem. Commun. 331 http://dx.doi.org/10.1039/a808775j
- NelsonPAttardG SOwenJ R 2002 Chem. Mater. 14 524 http://dx.doi.org/10.1021/cm011021a
- GuerinSAttardG S 2001 Electrochem. Commun. 3 544 http://dx.doi.org/10.1016/S1388-2481(01)00211-9
- ElliottJAttardG SBartlettP NColemanN R BMerckelD A SOwenJ R 1999 Chem. Mater. 11 3602 http://dx.doi.org/10.1021/cm991077t
- AttardG SLeclercS A AManiguetSRussellA ENandhakumarIBartlettP N 2001 Chem. Mater. 13 1444 http://dx.doi.org/10.1021/cm000850d
- BartlettP NSimonE 2003 Chem. Mater. 15 2962 http://dx.doi.org/10.1021/cm0210400
- BenderFMankelowR KHibbertD BGoodingJ J 2006 Electroanalysis 18 1558 http://dx.doi.org/10.1002/elan.200603585
- SchüthF 2001 Chem. Mater. 13 3184 http://dx.doi.org/10.1021/cm011030j
- YamauchiYYokoshimaTMommaTOsakaTKurodaK 2004 J. Mater. Chem. 14 2935 http://dx.doi.org/10.1039/b406265e
- ParkSBooHLeeSKimKKimHChungT 2008 Electrochim. Acta 53 6143 http://dx.doi.org/10.1016/j.electacta.2007.12.029
- BartlettP NBirkinP NGhanemM AdeGrootPSawickiM 2001 J. Electrochem. Soc. 148 C119 http://dx.doi.org/10.1149/1.1342178
- BartlettP NGollasBGuerinSMarwanJ 2002 Phys. Chem. Chem. Phys. 4 3835 http://dx.doi.org/10.1039/b201845d
- BartlettP NMarwanJ 2003 Micropor. Mesopor. Mater. 62 73 http://dx.doi.org/10.1016/S1387-1811(03)00394-9
- NandhakumarIElliottJ MAttardG S 2001 Chem. Mater. 13 3840 http://dx.doi.org/10.1021/cm010484d
- GabrielTNandhakumarI SAttardG S 2002 Electrochem. Commun. 4 610 http://dx.doi.org/10.1016/S1388-2481(02)00390-9
- LiXNandhakumarI SGabrielTAttardG SMarkhamM LSmithD CBaumbergJ JGovenderKO’BrienPSmyth-BoyleD 2006 J. Mater. Chem. 16 3207 http://dx.doi.org/10.1039/b603911a
- NandhakumarI SGabrielTLiXAttardG SMarkhamM LSmithD CBaumbergJ J 2004 Chem. Commun. 1374 http://dx.doi.org/10.1039/b403423f
- WolosiukAArmaganOBraunP 2005 J. Am. Chem. Soc. 127 16356 http://dx.doi.org/10.1021/ja054927g
- DagÖVermaAOzinGKresgeC 1999 J. Mater. Chem. 9 1475 http://dx.doi.org/10.1039/a900955h
- ÇelikÖ DagÖ 2001 Angew. Chem., Int. Ed. Engl. 40 3800 http://dx.doi.org/10.1002/1521-3773(20011015)40:20<3799::AID-ANIE3799>3.0.CO;2-I
- DagÖSamarskayaOTuraCGünayAÇelikÖ 2003 Langmuir 19 3671 http://dx.doi.org/10.1021/la020740g
- DagÖ AlayoğluSTuraCÇelikÖ 2003 Chem. Mater. 15 2711 http://dx.doi.org/10.1021/cm0341538
- DagÖAlayoğluSUysalÌ 2004 J. Phys. Chem. B 108 8439 http://dx.doi.org/10.1021/jp049716x
- AlbayrakCGültenGDagÖ 2007 Langmuir 23 855 http://dx.doi.org/10.1021/la0622119
- AlbayrakCSoyluADagÖ 2008 Langmuir 24 10592 http://dx.doi.org/10.1021/la8022453
- TürkerYDagÖ 2008 J. Mater. Chem. 18 3467 http://dx.doi.org/10.1039/b804344b
- DingJ HGinD L 2000 Chem. Mater. 12 22 http://dx.doi.org/10.1021/cm990603d
- BraunP VOsenarPStuppS I 1996 Nature 380 325 http://dx.doi.org/10.1038/380325a0
- BraunP VOsenarPTohverVKennedyS BStuppS I 1999 J. Am. Chem. Soc. 121 7302 http://dx.doi.org/10.1021/ja9833725
- BraunP VStuppS I 1999 Mater. Res. Bull. 3 463 http://dx.doi.org/10.1016/S0025-5408(99)00030-6
- O’SullivanE CWardA JBuddT 1994 Langmuir 10 2985 http://dx.doi.org/10.1021/la00021a023
- ZhangGChenXZhaoJChaiYZhuangWWangL 2006 Mater. Lett. 60 2889 http://dx.doi.org/10.1016/j.matlet.2006.02.009
- GuoRLiuT 1997 Colloids Surf. A 123–124 587 http://dx.doi.org/10.1016/S0927-7757(96)03780-6
- DillingerT MBraunP V 2002 Scr. Mater. A 44 1893 http://dx.doi.org/10.1016/S1359-6462(01)00813-2
- JiangXXieYLuJZhuLHeWQianY 2001 Chem. Mater. 13 1213 http://dx.doi.org/10.1021/cm0006143
- LiYWanJ HGuZ 1999 Acta Phys.-Chim. Sin. 15 1
- ZhangDQiLChengHMaJ 2002 J. Colloid Interface Sci. 246 413 http://dx.doi.org/10.1006/jcis.2001.8081
- HuangLWangHWangZMitraABozhilovK NYanY 2002 Adv. Mater. 14 61 http://dx.doi.org/10.1002/1521-4095(20020104)14:1<61::AID-ADMA61>3.0.CO;2-Y
- QiLGaoYMaJ 1999 Colloids Surf. A 157 285 http://dx.doi.org/10.1016/S0927-7757(99)00053-9
- HuangLWangHWangZMitraA PZhaoDYanY 2002 Chem. Mater. 14 876 http://dx.doi.org/10.1021/cm010819r
- KijimaTIkedaTYadaMMachidaM 2002 Langmuir 18 6453 http://dx.doi.org/10.1021/la0118154
- KijimaTYoshimuraTUotaMIkedaTFujikawaDMouriSUoyamaS 2004 Angew. Chem., Int. Ed. Engl. 43 228 http://dx.doi.org/10.1002/anie.200352630
- KawasakiHUotaMYoshimuraTFujikawaDSakaiGAnnakaMKijimaT 2005 Langmuir 21 11468 http://dx.doi.org/10.1021/la051247i
- SakaiGYoshimuraTIsohataSUotaMKawasakiHKuwaharaTFujikawaDKijimaT 2007 Adv. Mater. 19 237 http://dx.doi.org/10.1002/adma.200601127
- KijimaT et al 2009 Adv. Funct. Mater. 19 545 http://dx.doi.org/10.1002/adfm.200800966
- WangLChenXZhanJSuiZZhaoJSunZ 2004 Chem. Lett. 33 720 http://dx.doi.org/10.1246/cl.2004.720
- WangLChenXZhanJChaiYYangCXuLZhuangWJingB 2005 J. Phys. Chem. B 109 3189 http://dx.doi.org/10.1021/jp0449152
- WangLChenXChaiYHaoJ 2007 Colloids Surf. A 293 95 http://dx.doi.org/10.1016/j.colsurfa.2006.07.012
- WangLChenXSunZChaiY 2007 Can. J. Chem. Eng. 85 598 http://dx.doi.org/10.1139/V07-068
- SunZChenXWangLZhangGJingB 2008 Colloid Surf. A 326 23 http://dx.doi.org/10.1016/j.colsurfa.2008.05.006