Abstract
Dental ceramic implants have shown superior esthetic behavior and the absence of induced allergic disorders when compared to titanium implants. Zirconia may become a potential candidate to be used as an alternative to titanium dental implants if surface modifications are introduced. In this work, bioactive micropatterned silica coatings were produced on zirconia substrates, using a combined methodology of sol–gel processing and soft lithography. The aim of the work was to compare the in vitro behavior of human gingival fibroblasts (HGFs) and human dermal microvascular endothelial cells (HDMECs) on three types of silica-coated zirconia surfaces: flat and micropatterned (with pillars and with parallel grooves). Our results showed that cells had a higher metabolic activity (HGF, HDMEC) and increased gene expression levels of fibroblast-specific protein-1 (FSP-1) and collagen type I (COL I) on surfaces with pillars. Nevertheless, parallel grooved surfaces were able to guide cell growth. Even capillary tube-like networks of HDMEC were oriented according to the surface geometry. Zirconia and silica with different topographies have shown to be blood compatible and silica coating reduced bacteria adhesion. All together, the results indicated that microstructured bioactive coating seems to be an efficient strategy to improve soft tissue integration on zirconia implants, protecting implants from peri-implant inflammation and improving long-term implant stabilization. This new approach of micropatterned silica coating on zirconia substrates can generate promising novel dental implants, with surfaces that provide physical cues to guide cells and enhance their behavior.
Introduction
Micro- and nano-fabricated substrates with different surface patterns have shown to be able to induce changes in morphology, orientation and alignment of adherent cells in a variety of materials for several biomedical applications. With the combination of adequate material chemistry and the right patterns, the cell contact guidance phenomenon can occur [Citation1–Citation3] in different cell types such as neurons, osteoblasts, myoblasts, fibroblasts, endothelial cells, epithelial cells, macrophages and neutrophils which are able to adjust their orientation and alignment [Citation1, Citation3] to a wide variety of topographic geometries such as pillars, fibers, pits and grooves [Citation1, Citation3]. In addition, Kolind et al have shown that micro and nanoscale surface patterns influence mesenchymal stem cell (MSC) differentiation. Changes induced in the cell shape and cytoskeleton may rearrange cell components and alter the gene/protein response leading stem cell commitment to a specific lineage. However, to understand these interactions between materials and cells much more studies are needed [Citation4].
Surface patterning can be helpful in tissue engineering approaches defining an oriented cell growth which is important in nerve and tendon repair and regeneration [Citation3], cardiac constructs [Citation5] and new bone formation. The right surface pattern allows cell orientation leading to functional tissue architectures [Citation5].
For dental implant success it is essential to have a good osseointegration, where the presence of patterned surfaces can help to achieve this goal in combination with an adequate material.
Titanium and its alloys are currently the most used metals in dental implants due to their high resistance to corrosion in a physiological environment, excellent biocompatibility and mechanical properties, thus being a choice material for intraosseous use [Citation6, Citation7]. However, recent studies have reported clinical cases of patients allergic to titanium. Several symptoms have been described such as skin rashes, implant failure, non-specific immune suppression or even facial eczema [Citation8]. Released products from titanium were found in higher concentration inside tissues that surrounded dental implants. Furthermore, these released products can combine with proteins resulting in a protein–metal complex with immunogenic characteristics [Citation8, Citation9]. In addition, titanium shows poor esthetic behavior since it has gray color, which may become a problem when visible, by tarnishing the gingiva. This can happen with time if patients have a thin gingival biotype or undergo peri-implant recession. To overcome the described problems, ceramics can be considered as an alternative, since its natural color is similar to the teeth root [Citation10–Citation12].
Among the ceramic materials, zirconia is a good candidate because it is biocompatible and shows high fracture toughness and bending strength. It is used as a dental implant material to replace anterior teeth and molars because in contrast to other ceramic materials it has a good long-term strength. Previous studies showed that zirconia ceramics have mechanical properties similar to stainless steel with a compression resistance of 2000 MPa and a resistance to traction between 900 and 1200 MPa [Citation13–Citation15]. Several biological studies in vitro have been performed and have shown that zirconia is non-cytotoxic [Citation16, Citation17].
In vivo studies demonstrated that zirconia implants have a good osseointegration under unloading and loading conditions and no adverse effects were reported when it was inserted into bones or muscles. Zirconia is a chemically inert material; therefore different strategies can be used to improve the implant bioactivity and for a higher long-term implant performance success rate. For these reasons, in this work, zirconia was chosen to be used as a model material to study the interaction between micropatterned surfaces and cells. To improve the bioactive implant properties, samples were covered with silica and a well-defined and controllable surface topography was obtained using the soft lithography technology, having reproducible patterns whose elements have sizes within the ‘micron’ range (1–10 μm) [Citation18].
The success of dental implants involves wound healing of hard and soft tissues, where endothelial cells assume a primordial role in angiogenesis and inflammation during the wound healing process [Citation19]. Furthermore, the mouth environment has several types of pathogenic bacteria that can lead to peri-implantitis. To avoid bacteria access to the newly formed bone, it is important to establish a soft tissue barrier capable of protecting the peri-implant structure [Citation20]. Our main aim was to improve the adhesion of soft tissue to the implant so that this can be used as a first barrier against infection. The strategy used to achieve this goal was to modify the implant surface topography and chemical composition and study cell behavior on the surfaces. Endothelial and fibroblast cells were chosen for the biological study since soft tissue is highly vascularized and consists of fibroblasts which maintain the structural integrity of connective tissues [Citation21, Citation22].
Taking these issues into account, micropatterned silica coatings on zirconia substrates were produced using a combined methodology of sol–gel and soft lithography with different geometric shaped patterns. The in vitro behavior of human dermal microvascular endothelial cells (HDMECs) and human gingival fibroblasts (HGFs) was compared on different silica microfabricated surfaces.
Materials and methods
Material preparation
Zirconia sample preparation.
Dense cylindrical zirconia samples were prepared using 3 mol% Y2O3–ZrO2 (3Y-TZP) powder (TZ-3YS-E, Tosoh, Japan) and compressed by uniaxial pressing. Afterwards, samples were pre-sintered at 1100 °C with a heating rate of 5 °C min−1. The samples were polished and sintered at 1400 °C with a heating rate of 5 °C min−1 with 30 min dwelling time, followed by natural cooling inside the furnace.
Silica coating preparation.
Silica coatings were produced via a sol–gel method described elsewhere [Citation11, Citation23, Citation24]. Briefly, tetraethylorthosilicate (TEOS, Aldrich, USA) and methyltriethoxysilane (MTES, Aldrich, USA) were used as silicate precursors in a single stage process with acid catalysis. The silica solution was aged for 24 h before being applied on cylindrical zirconia substrates. Flat surfaces were produced using the dip coating technique.
Micropatterned surface production.
To produce micropatterned surfaces, two different lithographic methods were used. By photolithography, silicon masters were generated with the desired patterns (parallel grooves and pillars) in a clean room (Class 100) facility. Furthermore, polydimethylsiloxane (PDMS, Sigma, USA) was poured onto the silicon master and cured for 2 h. Negative molds with ordered geometric patterns were created with wells of 5 μm diameter, 5 μm depth and 10 μm edge-to-edge spacing. Patterns with parallel grooves were also produced with 5 μm depth and 10 μm edge-to-edge spacing. Zirconia samples were covered with silica gel and negative PDMS molds were used to stamp the pattern of interest. After 2 h the molds were removed and samples were heat treated up to 500 °C with 1 h plateau at 500 °C. The samples were characterized by scanning electron microscopy (SEM).
Biological characterization
Human gingival fibroblasts.
HGFs were purchased from Sciencell and cultured in cell culture plates with fibroblast medium (Sciencell, USA) containing 2% of fetal bovine serum (FBS, Sciencell, USA), 10 units ml−1 of penicillin, 10 μg ml−1 of streptomycin (1% of P/S solution, Sciencell, USA) and fibroblast growth supplement (1% of FGS, Sciencell, USA). Incubation was carried out in a humidified atmosphere of 95% air and 5% CO2 at 37 °C.
Human dermal microvascular endothelial cells.
HDMECs were purchased from Sciencell. The cell suspension was cultured in cell culture plates with endothelial (EC) culture basal medium (EGM-2, Lonza, USA) containing 2% of FBS (Lonza, USA), 10 units ml−1 of penicillin, 10 μg ml−1 of streptomycin (1% of P/S solution, Lonza, USA) and endothelial growth supplement (Lonza, USA). Incubation was carried out in a humidified atmosphere of 95% air and 5% CO2 at 37 °C.
Cells cultured on the material.
Zirconia silica coated samples were pre-incubated for 1 h in the respective cell culture medium, at 37 °C, in a humidified atmosphere of 95% air and 5% CO2. HGFs and HDMECs from passage 3 were seeded on four types of surfaces: cell culture treated-surface (TCP), silica flat surface and silica surfaces with pillars and parallel grooves, respectively, at a cell density of 104 cells cm−2. HGF and HDMEC cultures on the different surfaces were characterized throughout the incubation time (1, 3 and 7 days), as hereby indicated.
Biological assays.
At each time point, the metabolic activity and viability of HGFs and HDMECs were evaluated on the different silica coated surfaces using the MTT (3-(4,5-dimethylthiazol-2-yl)-2,5-diphenyltetrazolium bromide, Sigma, USA) assay. This assay was also performed in cultures growing on standard tissue-treated surfaces of polystyrene in the absence and in the presence of 1% phenol (Sigma, USA), as negative/positive controls, respectively. Cell culture plates with treated surfaces are hydrophilic and negatively charged, better for cell attachment and spreading. The positive control is in accordance with ISO 10993-5 and is frequently used as a positive control. It inhibits the cell proliferation rate and leads to cell morphological changes [Citation25]. Briefly, 10 μl of MTT solution (5 mg ml−1 in phosphate buffered saline (PBS)) was added to 100 μl of cell medium and incubated for 3 h at 37 °C. Afterwards, the solution was removed, and the formed formazan salts were dissolved in dimethylsulfoxide (200 μl, Sigma, USA). Finally, the solution was transferred to a 96-well plate, and the absorbance was read at 550 nm on an ELISA reader (Synergy HT, Bio tek, USA). The absorbance of the samples was normalized for the sample area.
To evaluate the HGF and HDMEC cell morphology on the different surfaces, immunostaining of the F-actin cytoskeleton and nucleus was carried out. Thus, cells were fixed for 15 min (4% formaldehyde, Sigma, USA) and permeabilized with 0.1% Triton X-100 for 5 min. Both types of cells were stained for F-actin with Alexa-Fluor-conjugated phalloidin (1:100 in 1% solution of bovine serum albumin and PBS, bovine serum albumin/PBS; Alexa Fluor® 488 Phalloidin, Molecular Probes, USA) for 1 h. Nuclei were stained with Dapi (Sigma, USA) diluted in PBS for 10 min. Samples were covered with Vectashield (Vector Laboratories, USA). Fluorescence images were acquired with an inverted microscope (Axiovert 200M, Zeiss). Also the cell morphology and distribution was assessed using a FEI Quanta 400FEG ESEM/EDAX Genesis X4M scanning electron microscope where cells were fixed and dehydrated in a graded series of alcohols and hexamethyldisilazane (HMDS, Ted Pella, USA). Samples were collected at days 1, 3 and 7.
To assess the RNA expression of some HGF and HDMEC characteristic genes, a reverse-transcription polymerase chain reaction (RT-PCR) was performed. The total RNA was extracted from the cell culture using the RiboPure™ Kit (Life Technologies, USA) according to the manufacturer´s instructions. RT-PCR amplification (Titan One Tube RT-PCR system, Roche, New Jersey, USA) was performed for 30 cycles. RT reaction mixtures consisted of RNA extracted, Titan RT-PCR buffer, dithiothreitol (DTT), deoxynucleoside triphosphate, primers for each gene tested, avian myeloblastosis virus RT (AMV-RT) and water, in a total volume of 25 μl. Total RNA was reverse transcribed with AMV reverse transcriptase, at 50 °C for 30 min, followed by a 2 min denaturation at 94 °C. The cDNAs were then amplifiled with recombinant Taq-DNA polymerase under the following conditions: 30 cycles of denaturation (94 °C per 30 s), annealing (58 °C per 30 s), elongation (68 °C per 45 s), followed by a prolonged elongation of 7 min at 68 °C. Table shows the primer sequences used for PCR amplification. The PCR products were separated by 2% agarose gel electrophoresis, and visualized by ethidium bromide staining. The images of the gel were captured with a camera and analyzed with Image J software. A semi-quantitative assessment of gene expression was performed and data were normalized with the density of glyceraldehyde-3-phosphate dehydrogenase (GAPDH). Samples were collected at day 7.
Table 1. Primers for PCR amplification.
The in vitro capillary tube-like formation assay was performed using the BD Matrigel Matrix (BD Biosciences, Franklin Lakes, New Jersey, USA) on samples of glass substrates covered with micropatterned silica coatings. HDMECs were cultured on samples with supplemented endothelial (EC) culture basal medium (EGM-2, Lonza, USA) at a concentration of 104 cells cm−2. After 6 days of culture, the medium was removed and 500 μl of Matrigel were added and incubated for 60 min at 37 °C. Afterwards, 500 μl of EC culture medium were added to each well of a 24-well culture plate. After 3 days of culture with Matrigel, capillary tube-like formation was assessed under an inverted microscope (Axiovert 200M, Zeiss, Germany). Phase contrast images were obtained.
Hemolysis assay and hemostatic studies.
Twenty milliliters of blood were collected by venipunction from four healthy human volunteers into tubes with sodium citrate as an anticoagulant to perform hemolysis assays and hemostatic studies.
Hemolysis assay. The hemolysis assay was performed according to the Standard Practice for Assessment of Hemolytic Properties of Materials from the American Society for Testing and Materials (ASTMF756-00, 2000). The hemoglobin concentration was measured using an automatic blood cell counter (Sysmex K1000; Sysmex, Hamburg, Germany). Subsequently, the blood was diluted with PBS, pH 7.4, to obtain a concentration of 8 g dl−1 of hemoglobin. A calibration curve for the hemoglobin concentration was performed. The different material samples (zirconia, silica flat surface, silica grooves and silica pillars) and the negative control (polypropylene) were placed in contact with blood, in duplicate (265 mg of biomaterial with 400 μl of diluted blood). During the incubation the samples were gently agitated every 60 min for 3 h. Afterwards, blood samples were centrifuged at 1600g for 10 min and the supernatant was carefully removed for spectroscopic analysis. The absorbance of the supernatant was measured at 540 nm and the hemolysis percentage was calculated according to hemoglobin concentrations estimated by the calibration curve.
Hemostatic studies. Blood was centrifuged at 200g for 10 min in order to obtain platelet-rich plasma (PRP). The four materials and negative control were incubated at 37 °C with PRP for 2 h, in duplicate. Thereafter, platelet counting was performed in the supernatant using an automatic blood cell counter (Sysmex K1000; Sysmex, Hamburg, Germany), in order to evaluate the difference in platelet adherence to the biomaterials. The incubated biomaterials were rinsed four times with PBS, fixed with Karnovsky solution and dehydrated in graded series of alcohols (70, 90 and 100%). Finally, the samples were mounted on aluminum stubs using double-side adhesive tape and sputter coated with a gold/palladium thin film. The SEM evaluation was performed using a high-resolution environmental SEM combined with x-ray microanalysis and electron backscattered diffraction analysis (Quanta 400 FEG ESEM/EDAX Genesis X4M) to evaluate platelet adhesion and activation. Moreover, this PRP was centrifuged in order to obtain platelet-poor plasma to evaluate the activated partial thromboplastin time (APTT) and prothrombin time (PT).
Bacterial culture.
Streptococcus sobrinus DSM20742 (DSMZ, Germany) bacteria were harvested onto brain heart infusion (BHI, Liofilchem, Italy) culture plates at 37 °C. After 48 h, bacteria cells were re-suspended in physiologic saline solution (0.9% NaCl in PBS) to obtain a concentration of 1.5 × 108 colony forming unit cells per ml, according to the McFarland standard, using a densitometer (BioMerieux, France). The materials tested (zirconia, silica flat surface, silica with grooves and silica with pillars) were incubated with 1 ml of the bacterial suspension at 37 °C for 90 min in triplicate. Following incubation, materials were rinsed two times with PBS in order to remove non-adherent or loosely adherent bacteria. Thereafter, the samples were transferred to sterile borosilicate glass tubes with PBS and sonicated for 1 s at 20 kHz (MS 73 probe, Sonopuls HD 2200, Bandelin, Germany). Finally, serial dilutions of the sonicated solutions were inoculated onto BHI (Liofilchem, Italy) culture plates at 37 °C under microaerofilic conditions. After 24 h, the number of adherent bacteria was counted. Bacteria morphology and distribution were assessed using a FEI Quanta 400FEG ESEM/EDAX Genesis X4M SEM where bacteria were dehydrated in a graded series of alcohols.
For statistical analysis, results were expressed as arithmetic mean ± standard deviation (SD) and triplicate experiments were performed. Analysis of results was carried out using the Graphpad Prism and statistical analysis was carried out using one-way ANOVA, with a significance level of p < 0.05.
Results and discussion
Recent studies showed that textured surfaces at the micro- and nano-scale are important to modulate and understand cell/material interactions. They can be used to achieve a higher long-term implant success rate and to produce functional biomedical devices (such as biosensors) [Citation18]. Considerable research has been directed toward controlling the spatial organization of cells in defined microarchitectures. A multitude of techniques have been described to control two-dimensional cellular alignment on micro- and nanostructured surfaces produced either by chemical or topographic patterning [Citation26–Citation28].
Recently, Pelaez-Vargas et al [Citation11] proposed a new method to produce micropatterned silica thin films on zirconia substrates through a synergy of sol–gel chemistry and soft lithography technology. The aim of the work was to explore zirconia ceramics as a competitive alternative material for dental implant application, with a modified surface to guide tissue growth, diminish bacterial adhesion and eliminate the use of titanium having disadvantages such as gingival tarnishing problems. Pelaez-Vargas et al [Citation11, Citation29] characterized the samples with several techniques. Using a combination of SEM and energy-dispersive x-ray spectroscopy techniques it was verified that the ZrO2 polycrystalline ceramics used were homogeneous and finely equiaxed. Furthermore, the additive (Y2O3) was uniformly distributed on zirconia [Citation11]. Silica coating was also characterized by Fourier transform infrared spectroscopy where it was possible to confirm the existence of SiO2 lattice. X-ray photoelectron spectroscopy analyses also confirmed through the elemental percentage analysis of the surface that it was composed of 63.5% of O and 36.5% of Si [Citation30]. Contact angles were determined for all types of the studied surfaces using the sessile drop method. Patterned silica surfaces presented hydrophobic behavior, while the flat silica surface was hydrophilic showing that the surface wettability of the samples was greatly influenced by the patterns [Citation29, Citation30]. Pelaez-Vargas et al [Citation11] performed in vitro studies with the osteoblastic cell line MG-63 and showed that cells were able to adhere and proliferate. Furthermore, the surface topography modulated their morphology where it was observed that cells were able to elongate and migrate throughout the patterns. A reproducible cell response was achieved, nevertheless these observations cannot be extrapolated to all types of cells because it depends on their origins, size and functions [Citation11].
The same methodology (sol–gel and soft lithography) was used in this work aiming to study the influence of different shape topographies on cells that are present in soft gingival tissues.
In this study, silica coated surfaces were analyzed by SEM, and it was observed that parallel grooves and pillar features of PDMS molds were reproduced with success on zirconia silica coating samples (figure ). Pillar shapes were reproduced in silica coatings with ∼5 μm diameter and ∼10 μm edge–edge spacing (figure (b)). Patterns with parallel shaped grooves were also reproduced with ∼5 μm width and ∼10 μm edge-to-edge spacing (figure (c)).
Figure 1 SEM images of the silica coated zirconia substrate. (A) Flat silica coating; micropatterned silica coatings with pillars (B) and parallel grooves (C). To evaluate the cell viability and metabolic activity of HGFs and HDMECs on the surfaces, the MTT assay was used. Data showed that the metabolic activity increased with culture time for both types of cells on all surfaces tested. Scale bar = 50 μm.
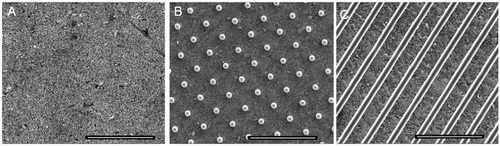
MTT values were markedly higher on pillar surfaces after 7 days of culture compared to other surfaces (figure ).
Figure 2 MTT activity assay for HGFs (A) and HDMECs (B) on flat, grooved and pillar surfaces. Negative and positive control results are also shown. Values reported are the mean ± SD. Assays were performed after 1, 3 and 7 days of culture; ∗ indicates significant statistical difference (p < 0.05; n = 3).
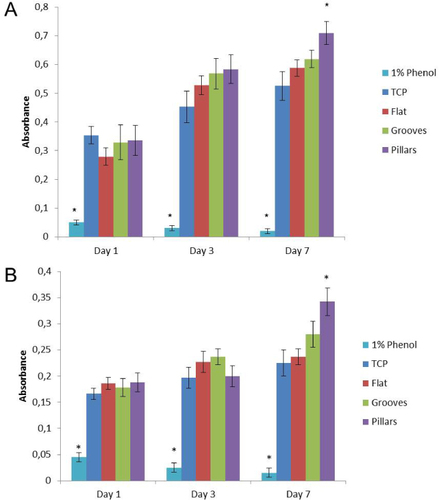
A semi-quantitative RT-PCR analysis was performed to evaluate the expression of phenotype markers for HGFs and HDMECs on all the studied surfaces (figure ). Cultures were assessed for the genes fibroblast-specific protein-1 (FSP-1) and collagen-type 1 (COL-1) and genes expressed by fibroblast cells and von Willebrand factor (vWF) and CD31 as characteristic endothelial cell markers. Expression of these genes was evaluated at day 7, the same time point where the higher metabolic activity and cell viability was achieved. HDMECs were able to express vWF and CD31 for all the surfaces. Regarding HGF culture, these cells expressed FSP-1 and COL1 for all the surfaces with a significantly higher expression in pillar surfaces (figure (b)).
Figure 3 RT-PCR analysis of fibroblast and endothelial-associated markers: FSP-1, COL-1, CD31 and vWF, after 7 days of cell culture on TCP, flat, grooved and pillar surfaces. Panels (A) and (C) are representative agarose gel of PCR products. Panels (B) and (D) show densiometric analyses of PCR products, normalized to the corresponding GAPDH and β-actin value. Values reported are the mean ± SD; ∗, statistically significantly different (p < 0.05; n = 3).
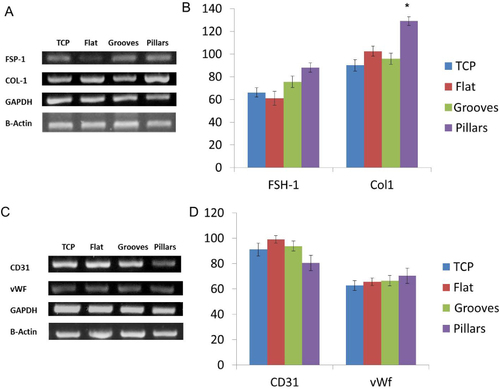
Collagen type 1 is a very important protein that is present in the extracellular matrix and shows different chemistry and physical cues that can dictate cell response. It is not only the diffusible factors that are important for cell behavior but also the adhesive and mechanical interactions with the material [Citation2, Citation31]. Cell behavior can be modulated by the surface roughness and topography via integrin receptors. Integrin expression can be different according to the cell state of differentiation or the type of surface where cells are seeded. The activation of integrin receptors leads to a series of reactions (signal transduction cascades) [Citation2]. The activation of signaling pathways can lead to different synergistic responses. For example, it was described that surface roughness increases MG63 expression levels of prostaglandins which are necessary for normal osteoblast differentiation [Citation31].
We hypothesized that a well-defined geometrical surface could promote cell organization and guided growth depending on the geometric pattern in question and the cell type. Furthermore, previous studies showed that the cell shape and size can influence the MSC differentiation fate [Citation32]. Microtextured substrates with different geometries influence cytoskeletal dynamics and motility as well as the focal adhesion localization/distribution [Citation3, Citation33]. The degree of cytoskeletal tension affects cell regulatory mechanisms of mechanically stimulated differentiation, not yet explained [Citation32, Citation34]. Furthermore, previous studies showed that cytoskeletal tension regulates the cell shape and promotes higher metabolic activity as well as overexpression of some proteins required for cell functionality [Citation35].
Also, previous studies on endothelial cells showed that this type of cell responds to ridge-groove surfaces through alignment (contact guidance), elongation, reduced proliferation and enhanced migration compared with smooth surfaces [Citation2, Citation36]. However, Uttayarat et al [Citation3] cultured bovine aortic endothelial cells on micro- to nanogrooved models of poly(dimethylsiloxane) surfaces and showed that cell proliferation was similar on smooth surfaces compared to grooved surfaces. It was also shown that the alignment along the pattern was related to the alignment of focal adhesion and actin filaments [Citation3, Citation36]. Regarding the pillar pattern surface, Nematollahi et al [Citation37] showed that epithelial cells cultured on pillar substrates exhibited a lower proliferation and slower cell migration when compared with smooth surfaces. Nevertheless, Kaivosoja et al [Citation38] showed that pillars could cause stretching of the cell cytoskeleton and enhanced extracellular signal-regulated kinases expression, which regulates osteogenesis. Pillar surfaces improved osteogenesis and cell proliferation. The influence of micropatterning is still poorly understood and there is no consensus on the best shape topography for cell adhesion, proliferation and differentiation. Taking these issues into account in this work we decided to study the influence of different shape topographies on cell behavior using the same technique to produce the shapes. The best results were achieved on the pillar surface in MTT and PCR assays, so we assume that pillar surface geometry stimulates endothelial cell and fibroblast regulation throughout the mechanical/physical stimulus, improving the cell metabolic activity and collagen type 1 expression.
Cell morphology and adhesion were evaluated on the first day of culture. Both types of cells had similar behavior at this time-point. They were more spread on silica flat surfaces compared with micropatterned surfaces. HDMECs seem to be more spread on parallel groove surfaces compared with pillars, but both types of cells showed several lamellopodia extensions directed toward pillars (figure ). Cells preferentially grew on the top of the pillars and ridges, on the first day of culture.
Figure 4 SEM images showing HFG and HDMEC morphology, after 1 day of culture on flat, grooved and pillar surfaces. Scale bar = 20 μm.
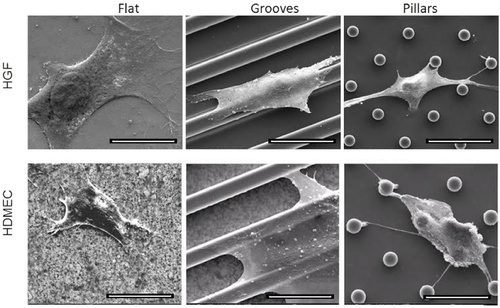
After 7 days of culture, HGFs and HDMECs were randomly distributed on silica flat and pillar surfaces with no clear growth alignment. On the other hand, on micropatterned surfaces with parallel grooves both types of cells grew in a unidirectional alignment according to the surface. HGFs and HDMECs were clearly more elongated when compared with cells seeded on the other surfaces (figure ).
Figure 5 Inverted fluorescence microscopy images of HFGs and HDMECs after 7 days of culture, on flat, grooved and pillar surfaces. Scale bar = 50 μm.
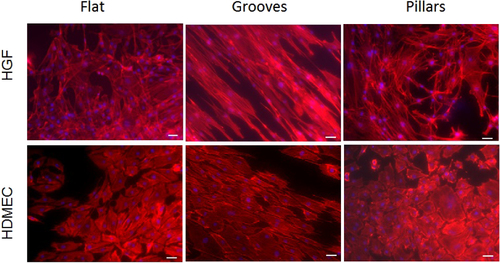
Matrigel induced a well-defined capillary like tube formation for HDMECs in all tested surfaces, after 3 days of culture (figure ). On flat surfaces, cells presented a higher density of capillary-like tubes, but with a random distribution and a shorter length, when compared to HDMECs seeded on micropatterned surfaces, where an increased length of capillary-like tubes was observed (figure ). On surfaces with parallel grooves, cells showed enhanced alignment and organization (figure (b), see the white arrow).
Figure 6 Matrigel assay images of capillary-like tube formation of HDMECs after 3 days of culture, on flat (a), grooved surfaces (b) and pillars (c). The direction of the parallel microtopographic features is indicated by the white arrow. Scale bar = 100 μm.
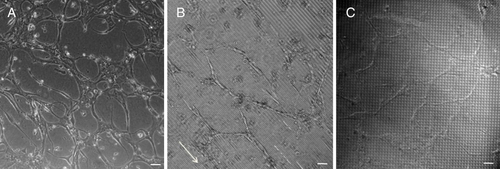
Surfaces with parallel grooves clearly allowed an oriented cell growth, being the most adequate patterns to be used for guided tissue application. Pattern alterations in cell metabolic activity and the level of COL1 and FSP-1 gene expression were observed on pillar surfaces suggesting that this type of surface could be used for different applications, where an increased tissue growth or specific protein stimulation could be achieved.
After insertion, the dental implant surface also interacts with the patient's blood and influences fibrin network formation and the acute inflammatory process. The implant topography (on a micro and nanoscale) and chemistry will affect the peri-implant and bone growth. Titanium is the first choice material for dental implants, but it has been reported as a thrombogenic material [Citation39]. However, zirconia is a bioinert material with minimal ion release that has been pointed out as a promising alternative for dental implants. Taking into account these issues, the in vitro toxicity of zirconia and silica micropatterned materials to human erythrocytes was evaluated in this work. According to ASTM (Standard Practice for Assessment of Hemolytic Properties of Materials, American Society for Testing and Materials Designation: ASTM: F 756-00), if the hemolysis percentage is below 2% the material is considered as non-hemolytic, if between 2 and 5% it is considered as slightly hemolytic and if higher than 5% it is considered as hemolytic. Zirconia samples presented a hemolysis percentage of 0.15 ± 0.04% very similar to silica flat and micropatterned (grooves and pillars) surfaces which had the percentages of 0.16 ± 0.04, 0.15 ± 0.05 and 0.16 ± 0.03%, respectively. All samples showed no hemolytic activity, since the percentage of hemolysis was less than 1% and similar to that found in the negative control (0.16 ± 0.03%).
Platelet adhesion and activation and coagulation cascade activation assessments are very important to evaluate the biomaterial blood compatibility, namely to evaluate the probability of thrombus formation. As shown in figures and the platelet counts were similar in PRP incubated with different biomaterials, including the polypropylene used as a non-thrombogenic control material. SEM evaluation of the material surface showed that platelets adhere to all materials, in small groups and limited to small areas of the sample surface (figure ). We can also see that platelets presented a flattened morphology with some pseudopodial processes. This extended morphology of platelets has been demonstrated as an indicator of platelet activation; however, similar results were found in all tested materials and in polypropylene used as a non-thrombogenic control material. Moreover, a screening for coagulation cascade activation was performed by measuring TP and APTT in the plasma samples incubated with the different materials, and no alterations were found (figures (A) and (B)). These results suggest that zirconia and silica with different morphologies are blood compatible and non-thrombogenic materials, as the results are similar to that found for polypropylene material.
Figure 7 Evaluation of platelet adhesion and activation by SEM. Polypropylene (A), (B), zirconia (C), (D), flat silica (E), (F) and silica with grooves (G), (H) and with pillars (I), (J). (A), (C), (E), (G), (I) Scale bar = 100 μm; (B), (D), (F), (H), (J) scale bar = 20 μm.
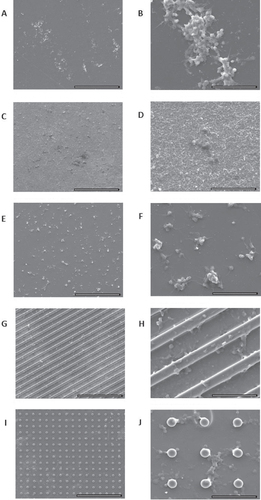
Figure 8 Platelet counts (A); APTT and PT (B), (C) for negative control (polypropylene membrane), zirconia, silica flat and micropatterned (grooves and pillars) surfaces.
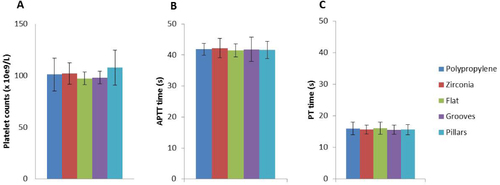
Dental caries and periodontal diseases have been related to bacterial accumulation. The early colonizer bacteria are the first to adhere to enamel or to implants and provide attachment substrates for the next colonizers, influencing the subsequent biofilm formation. This type of bacteria species plays an important role in dental biofilm formation which is decisive in determining oral health or disease [Citation40]. Several Streptoccoccus species such as S. sanguinis, S. oralis, S. gordonii, S. mitis and S. sobrinus constitute 60–80% of the early colonizers [Citation41]. For this reason S. sobrinus was chosen to test the bacteria adhesion to zirconia and silica micropatterned surfaces. Our results showed that after 90 min of incubation, bacteria were able to adhere and grow on all types of surfaces, maintaining their morphology and organizing themselves in small clusters (figure ). S. sobrinus was able to colonize the top of ridges and pillars, being present in a higher number in the space between pillars since they had more area available to grow (figure ). Zirconia samples showed significantly higher values of adherent bacteria when compared to silica flat and grooved surfaces (figure ) what might be related to the higher porosity and irregular morphology previously observed in zirconia samples [Citation11]. Moreover, previous studies showed that bacteria adhere preferentially to more irregular surface morphologies, in order to maximize the bacteria surface area. Furthermore, more roughness surface areas leave a higher surface area available for their adhesion and protection from shear forces [Citation36]. Nevertheless, Rimondini et al [Citation42] have reported an in vivo study showing that zirconia stabilized with yttria accumulated less bacteria when compared to titanium. Silica flat and micropatterned cover did not significantly increase the number of adherent bacteria compared to the zirconia substrate. These last results, once more, reinforced the beneficial potential use of the developed materials for dental implant applications.
Conclusions
In this work, reproducible materials were produced using a combined methodology of sol–gel and soft lithography. Different surface topographies with pillar and groove patterns were obtained on silica thin films coating zirconia substrates. HGFs and HDMECs were able to adhere, migrate and proliferate on the micropatterned coatings. The micropatterned surfaces were able to modulate the cell response, playing a crucial role in orientation of cell growth, metabolic activity and expression of proteins, which are important for the cell functionality. Zirconia and silica with different morphologies were shown to be blood compatible. Silica coatings reduce bacterial adhesion compared to zirconia substrates. Our results suggest that the newly developed materials can improve dental implants.
Acknowledgments
The authors would like to thank FCT—Fundação para a Ciência e Tecnologia (Portugal) for grant FCT/PTDC/CTM/100120/2008 ‘Bonamidi’ and NSEC (Nanoscale Science and Engineering Center, USA). This work was also financed by FEDER funds through the Programa Operacional Factores de Competitividade—COMPETE and by Portuguese funds through FCT—Fundação para a Ciência e a Tecnologia in the framework of the project PEstC/SAU/LA0002/2011.
References
- ZhouFYuanLHuangHChenH 2009 Phenomenon of ‘contact guidance’ on the surface with nano-micro-groove-like pattern and cell physiological effects Chin. Sci. Bull. 54 3200 3205 3200–5 10.1007/s11434-009-0366-1
- BettingerC JZhangZGerechtSBorensteinJ TLangerR 2008 Enhancement of in vitro capillary tube formation by substrate nanotopography Adv. Mater. 20 99 103 99–103 10.1002/adma.200702487
- UttayaratPToworfeG KDietrichFLelkesP ICompostoR J 2005 Topographic guidance of endothelial cells on silicone surfaces with microto nanogrooves: orientation of actin filaments and focal adhesions J. Biomed. Mater. Res. A 75 668 680 668–80 10.1002/jbm.a.30478
- KolindKLeongK WBesenbacherFFossM 2012 Guidance of stem cell fate on 2D patterned surfaces Biomaterials 33 6626 6633 6626–33 10.1016/j.biomaterials.2012.05.070
- GerberichB GBhatiaS K 2013 Tissue scaffold surface patterning for clinical applications Biotechnol. J. 8 73 84 73–84 10.1002/biot.201200131
- ÖzcanMHämmerleC 2012 Titanium as a reconstruction and implant material in dentistry: advantages and pitfalls Materials 5 1528 1545 1528–45 10.3390/ma5091528
- WernerS 2009 The effect of microstructured surfaces and laminin-derived peptide coatings on soft tissue interactions with titanium dental implants Biomaterials 30 2291 2301 2291–301 10.1016/j.biomaterials.2009.01.004
- SiciliaA 2008 Titanium allergy in dental implant patients: a clinical study on 1500 consecutive patients. Clin. Oral Impl. Res. 19 823 835 823–35 10.1111/j.1600-0501.2008.01544.x
- EgusaHKoNShimazuTYataniH 2008 Suspected association of an allergic reaction with titanium dental implants: a clinical report J. Prosthet. Dent. 100 344 347 344–7 10.1016/S0022-3913(08)60233-4
- GahlertMRöhlingSWielandMSprecherC MKnihaHMilzS 2009 Osseointegration of zirconia and titanium dental implants: a histological and histomorphometrical study in the maxilla of pigs Clin. Oral Impl. Res. 20 1247 1253 1247–53 10.1111/j.1600-0501.2009.01734.x
- Pelaez-VargasA 2011 Isotropic micropatterned silica coatings on zirconia induce guided cell growth for dental implants Dent. Mater. 27 581 589 581–9 10.1016/j.dental.2011.02.014
- AdatiaN DBayneS CCooperL FThompsonJ Y 2009 Fracture resistance of yttria-stabilized zirconia dental implant abutments J. Prosthodont. 18 17 22 17–22 10.1111/j.1532-849X.2008.00378.x
- ManiconeP FRossi IommettiPRaffaelliL 2007 An overview of zirconia ceramics: basic properties and clinical applications J. Dent. 35 819 826 819–26 10.1016/j.jdent.2007.07.008
- SundhAMolinMSjögrenG 2005 Fracture resistance of yttrium oxide partially-stabilized zirconia all-ceramic bridges after veneering and mechanical fatigue testing Dent. Mater. 21 476 482 476–82 10.1016/j.dental.2004.07.013
- AttWGrigoriadouMStrubJ 2007 ZrO2 threeunit fixed partial dentures: comparison of failure load before and after exposure to a mastication simulator J. Oral Rehabil. 34 282 290 282–90 10.1111/j.1365-2842.2006.01705.x
- DionI 1994 Physico-chemistry and cytotoxicity of ceramics J. Mater. Sci.: Mater. Med. 5 18 24 18–24 10.1007/BF00121148
- JossetYOum'HamedZZarrinpourALorenzatoMAdnetJ-JLaurent-MaquinD 1999 In vitro reactions of human osteoblasts in culture with zirconia and alumina ceramics J. Biomed. Mater. Res. 47 481 493 481–93 10.1002/(SICI)1097-4636(19991215)47:4%3C481::AID-JBM4%3E3.0.CO;2-Y
- Pelaez-VargasA 2012 Micropatterned coatings for guided tissue regeneration in dental implantology Cell Interaction GowderS (Intech) ch 11
- AnNSchedleAWielandMAndrukhovOMatejkaMRausch-FanX 2010 Proliferation, behavior, and cytokine gene expression of human umbilical vascular endothelial cells in response to different titanium surfaces J. Biomed. Mater. Res. A 93 364 372 364–72
- MyshinH LWiensJ P 2005 Factors affecting soft tissue around dental implants: a review of the literature J. Prosthet. Dent. 94 440 444 440–4 10.1016/j.prosdent.2005.08.021
- MouleA JLiHBartoldP M 1995 Donor variability in the proliferation of human dental pulp fibroblasts Aust. Dent. J. 40 110 114 110–4 10.1111/j.1834-7819.1995.tb03125.x
- DarveauR P 2010 Periodontitis: a polymicrobial disruption of host homeostasis Nature Rev. Micro. 8 481 490 481–90 10.1038/nrmicro2337
- DuránACondeACoedoA GDoradoTGarcíaCCeréS 2004 Sol–gel coatings for protection and bioactivation of metals used in orthopaedic devices. J. Mater. Chem. 14 2282 2290 2282–90 10.1039/b401370k
- GarciaCCereSDuranA 2004 Bioactive coatings prepared by sol–gel on stainless steel 316L J. Non-Cryst. Solids 348 218 224 218–24 10.1016/j.jnoncrysol.2004.08.172
- IlleperumaR P 2012 Immortalized gingival fibroblasts as a cytotoxicity test model for dental materials J. Mater. Sci., Mater. Med. 23 753 762 753–62 10.1007/s10856-011-4473-6
- AubinH 2010 Directed 3D cell alignment and elongation in microengineered hydrogels Biomaterials 31 6941 6951 6941–51 10.1016/j.biomaterials.2010.05.056
- LuJRaoM PMacDonaldN CKhangDWebsterT J 2008 Improved endothelial cell adhesion and proliferation on patterned titanium surfaces with rationally designed, micrometer to nanometer features Acta Biomater. 4 192 201 192–201 10.1016/j.actbio.2007.07.008
- NikkhahMEdalatFManoucheriSKhademhosseiniA 2012 Engineering microscale topographies to control the cell–substrate interface Biomaterials 33 5230 5246 5230–46 10.1016/j.biomaterials.2012.03.079
- Pelaez-VargasAGallego-PerezDCarvalhoAFernandesM HHansfordD JMonteiroF J 2013 Effects of density of anisotropic microstamped silica thin films on guided bone tissue regeneration—in vitro study J. Biomed. Mater. Res. B, Appl. Biomater. 101B 762 769 762–9 10.1002/jbm.b.32879
- CarvalhoA 2012 Micropatterned silica thin films with nanohydroxyapatite micro-aggregates for guided tissue regeneration Dent. Mater. 28 1250 1260 1250–60 10.1016/j.dental.2012.09.002
- D'LimaD DLemperleS MChenP CHolmesR EColwellC WJr 1998 Bone response to implant surface morphology J. Arthroplasty. 13 928 934 928–34 10.1016/S0883-5403(98)90201-7
- KilianK ABugarijaBLahnB TMrksichM 2010 Geometric cues for directing the differentiation of mesenchymal stem cells Proc. Natl Acad. Sci. USA 107 4872 4877 4872–7 10.1073/pnas.0903269107
- von der MarkKParkJBauerSSchmukiP 2010 Nanoscale engineering of biomimetic surfaces: cues from the extracellular matrix Cell Tissue Res. 339 131 153 131–53 10.1007/s00441-009-0896-5
- SuntersA 2010 Mechano-transduction in osteoblastic cells involves strain-regulated estrogen receptor α-mediated control of insulin-like growth factor (IGF) I receptor sensitivity to ambient IGF, leading to phosphatidylinositol 3-kinase/AKT-dependent Wnt/LRP5 receptor-independent activation of β-catenin signaling J. Bio. Chem. 285 8743 8758 8743–58 10.1074/jbc.M109.027086
- MendonçaG 2009 The effects of implant surface nanoscale features on osteoblast-specific gene expression Biomaterials 30 4053 4062 4053–62 10.1016/j.biomaterials.2009.04.010
- AnselmeKDavidsonPPopaAGiazzonMLileyMPlouxL 2010 The interaction of cells and bacteria with surfaces structured at the nanometre scale Acta Biomater. 6 3824 3846 3824–46 10.1016/j.actbio.2010.04.001
- NematollahiMHamiltonDJaegerNBrunetteD 2009 Hexagonal micron scale pillars influence epithelial cell adhesion, morphology, proliferation, migration, and cytoskeletal arrangement J. Biomed. Mater. Res. A 91 149 157 149–57 10.1002/jbm.a.32202
- KaivosojaE 2013 Cell adhesion and osteogenic differentiation on threedimensional pillar surfaces J. Biomed. Mater. Res. A 101 842 852 842–52 10.1002/jbm.a.34378
- TrainiTCaputiSGherloneEDegidiMPiattelliA 2013 Fibrin clot extension on zirconia surface for dental implants: a quantitative in vitro study Clin. Impl. Dent. Relat. Res. at press
- LiJ 2004 Identification of early microbial colonizers in human dental biofilm J. Appl. Microbiol. 97 1311 1318 1311–8 10.1111/j.1365-2672.2004.02420.x
- AvilaMOjciusD MYilmazÖ 2009 The oral microbiota: living with a permanent guest DNA Cell Biol. 28 405 411 405–11 10.1089/dna.2009.0874
- RimondiniLCerroniLCarrassiATorricelliP 2002 Bacterial colonization of zirconia ceramic surfaces: an in vitro and in vivo study Int. J. Oral Maxillofac. Impl. 17 793 798 793–8