Abstract
We present magnetoconductivity and magnetoluminescence measurements in sandwich devices made from films of a π-conjugated molecule and demonstrate effects of more than 30 and 50% magnitude, respectively, in fields of 100 mT at room-temperature. It has previously been recognized that the effect is caused by hyperfine coupling, and that it is phenomenologically similar to other magnetic field effects that act on electron–hole pairs, which are well-known in spin-chemistry. However, we show that the very large magnitude of the effect contradicts present knowledge of the electron–hole pair recombination processes in electroluminescent π-conjugated molecules, and that the effect persists even in almost hole-only devices. Therefore, this effect is likely caused by the interaction of radical pairs of equal charge.
Introduction
The field of organic electronics, i.e. electronic devices that are based on π-conjugated organic semiconductors, has progressed at a rapid pace, and electronics based on organic thin-film materials could soon become a mainstay of semiconductor technology. Currently, the most immanent application appears to be as displays based on organic-light emitting diodes (OLEDs) which are widely tipped to replace the current liquid crystal display (LCD) technology. Yet the future may see a wider range of application for this technology, with an entirely new generation of ultralow-cost, lightweight and even flexible electronic devices in the offing, which will perform functions traditionally accomplished using much more expensive components based on conventional semiconductor materials such as silicon. Indeed, recently there has been growing interest in spin [Citation1–3] and magnetic field effects (MFE) [Citation4–15] in these materials. We recently observed [Citation4] a large, low field magnetoresistive effect (up to 10% at 10 mT and 300 K) in OLEDs, which we dubbed organic magnetoresistance (OMAR). Other laboratories have also observed this effect [Citation16–21]. OMAR poses a significant scientific puzzle since it is, to the best of our knowledge, the only known example of large room temperature magnetoresistance in non-magnetic materials with the exception of very-high-mobility materials [Citation22, Citation23]. Moreover, the discovery of OMAR begs the question why a corresponding effect has not yet been discovered in inorganic semiconductors, whereas it appears to be ubiquitous in organic semiconductors [Citation6]. We have previously shown that the abundance of hydrogen atoms in the organic compounds is essential for the observation of OMAR [Citation24, Citation25]. The hydrogen atom, more specifically the hydrogen nucleus, has long been known to result in various magnetic field effects in organics (see [Citation26] for a review). Frankevich et al [Citation27, Citation28] and Kalinowski et al [Citation8] studied the effect of magnetic fields on photoconductivity and electroluminescence in organic devices, and explained their findings using a model based on hyperfine interaction between electron/hole spin and the hydrogen nuclei in the organic molecules.
To the best of our knowledge, the exact mechanism causing OMAR is currently not known with certainty. Three kinds of models based on spin-dynamics induced by hyperfine interaction have recently been put forward to explain OMAR. (i) Electron–hole pair (EHP) mechanism models [Citation16–18, Citation20, Citation29–31] based on concepts borrowed from the so-called magnetic-field effects in radical pair [Citation8, Citation27]. In this model the spin-dependent reaction between oppositely charged polarons to form an exciton (‘recombination’) is of central importance. (ii) The triplet-exciton polaron quenching (TEQ) model [Citation19] that is based on the spin-dependent reaction between a triplet exciton and a polaron to give an excited singlet ground state. (iii) The bipolaron mechanism [Citation32] which treats the spin-dependent formation of doubly occupied sites (bipolarons) during the hopping transport through the organic film. In this paper, we will experimentally study whether OMAR can be explained by one of the relatively well-known excitonic magnetic-field effect models, or whether it requires a new explanation based on a single carrier-type only. We will show that the experimental data clearly points to the latter.
Experimental
The fabrication of the organic sandwich devices started with glass substrates coated with 40 nm of indium-tin-oxide (ITO) purchased from Delta Technologies. The conducting polymer poly(3,4-ethylenedioxythiophene)-poly(styrenesulfonate) (PEDOT), purchased from H C Starck, Inc., was spin coated at 2000 revolutions per minute (rpm) on top of the ITO to provide an efficient hole injecting electrode. All other fabrication steps were carried out in a nitrogen glove-box. The active small molecule layer of tris-(8-hydroxyquinoline) aluminum (Alq3), obtained from HWSands Corp., was thermally evaporated in high vacuum (10−6 mbar) onto ITO or the PEDOT covered ITO substrate, yielding an organic semiconductor layer thickness of 100 nm. The cathode, either Ca (with an Al capping layer), Al, or Au was then deposited by thermal (Ca) or electron beam evaporation (Al, Au) on top of the organic thin films. The device area was 1 mm2 for all the devices.
The samples were mounted on a closed cycle helium cryostat placed between the poles of an electromagnet. The magnetoconductance ratio was determined by measuring the change in current ΔI/I with magnetic field under constant bias voltage. For the magnetoluminescence traces, an applied ac voltage, modulated at about 1000 Hz in a square-wave between 0 V and a certain voltage level was used. The magnetoluminescence ratio was determined by measuring, using a lock-in amplifier, the change in electroluminescence intensity detected by a photomultiplier tube that was shielded from the magnetic field using a high saturation mu-shield foil.
Experimental results
Figure shows several magnetoconductivity, ΔI/I, traces measured at room temperature at different constant applied voltages in an Alq3 OLED. It is shown that magnetoconductivity values of up to 15% at 10 mT can be achieved. Figure shows ΔI/I (bold lines) and magnetic field induced change in electroluminescence intensity, ΔEL/EL (thin lines) in a similar device measured at different constant voltages. ΔEL/EL is always about twice as large as the corresponding ΔI/I. The observed ΔEL/EL can be as large as 56% at small currents. Next, we study the magnitude of the observed magnetoconductance in devices that use different cathode materials. Whereas the anode material (PEDOT) was chosen because it is known to result in near-Ohmic hole injection, we study cathode materials that are known to result in near-Ohmic electron injection (Ca) to almost no electron injection (Au), as well as an intermediate material (Al). This allows us to compare OMAR measured in well-balanced bipolar devices, which therefore have a large electroluminescence efficiency, η, to OMAR measured in effectively hole-only devices that show only a very poor η. Figure (a) shows the measured magnetoconductance ratio, ΔI/I at B=100 mT and several constant applied voltages resulting in a current flow between 1 and 100 μA in these devices as a function of η, which was determined from the ratio between emitted EL intensity and the driving current. It is shown that whereas η changes by more than two orders of magnitude, the maximum ΔI/I remains within a factor of two. In our opinion, this clearly shows that OMAR is a property of a single carrier-type, rather than requiring the presence of both electrons and holes. For completeness, the current–voltage characteristics of the devices are shown in figure (b). It is shown that the necessary driving voltage increases when going from a bipolar to a unipolar device. The lower driving voltage in bipolar devices is generally believed to be due to a cancelation of the respective space-charge fields of the electrons and holes.
Figure 1 Magnetoconductance ratio, ΔI/I in an ITO/Alq3 (≈100 nm)/Ca device measured at different constant voltages (the approximate current levels are also given) and at room temperature. The molecular structure of Alq3 and current–voltage characteristics of the device are shown as insets.
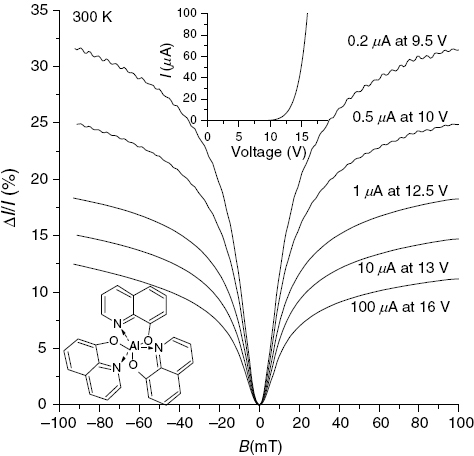
Figure 2 Magnetic field effect (MFE) on current (bold lines) and EL (thin lines) in a PEDOT/Alq3 (≈100 nm)/Ca device measured at several different constant voltages at room temperature. The current–voltage characteristics of the device is shown as an inset.
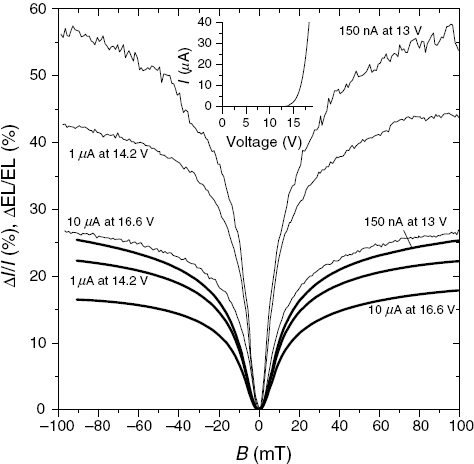
Figure 3 (a) Magnetoconductance ratio, ΔI/I at B=100 mT in several ITO/Alq3 (≈100 nm)/cathode devices with Ca, Al, or Au as the cathode as a function of the exciton/carrier ratio η. For each device, measurements at several different constant applied voltages resulting in a current between ≈1 μA and ≈100 μA are shown. (b) Current–voltage (I–V) characteristics of the devices.
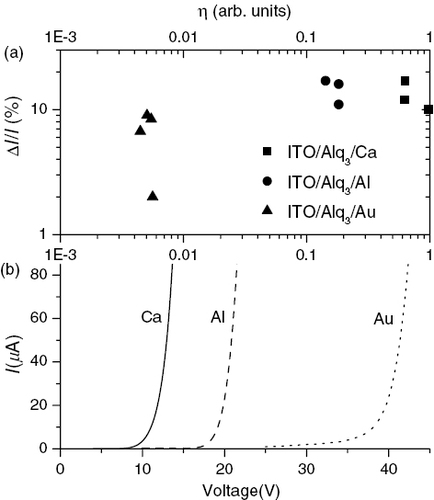
Discussion
First, we show that the largest observed magnitude of ΔEL/EL≈56% exceeds the maximum possible value predicted by the electron–hole pair model. We have previously shown [Citation33] through a very basic calculation that the maximum effect predicted by the electron–hole pair mechanism equals ΔEL/EL=50%. The predicted ΔEL/EL depends mainly on the ratio r≡kS/kT between the formation rate of singlet excitons and triplet excitons, kS and kT, respectively. If kS>kT, ΔEL/EL is negative and vice versa. Furthermore, ΔEL/EL=50% corresponds to the limit kS=0. Of course it can be argued that our measured effect is not much larger than the maximum possible value. This is correct, but the result given in [Citation33] clearly shows that the requirement for observing such large effects is that kS≪kT, which would lead to essentially non-luminescent devices. This is because the ratio between luminescent singlet excitons and non-luminescent is given by r/(r+3) [Citation1]. This clearly contradicts the well-known fact that Alq3 is one of the most efficient OLED materials. As a matter of fact, Baldo et al [Citation34] have measured the excitonic singlet to triplet ratio in Alq3 to be equal to 25% within the experimental uncertainty, which is the value predicted for kS≈kT. Therefore, the claim that the singlet formation rate is much smaller than that for triplets is clearly in contradiction with published measurements.
The most direct argument against an excitonic origin of OMAR follows from the data shown in figure (a) where a large OMAR effect is also observed in effectively hole-only devices using a Au cathode, where the number of exciton forming events is more than 100 times smaller than in well-balanced devices using a Ca cathode. However, we have to note that preliminary results in electron-only Alq3 devices show a greatly reduced OMAR magnitude. More study is therefore necessary, and we will present a detailed study of both electron and hole only devices in a forthcoming publication. Taken at face-value, our results imply that in Alq3 the OMAR effect is related to the hole transport, and apparently no OMAR effect is connected with the electron transport.
Bobbert et al [Citation32] have recently put forward a hyperfine mechanism that acts on a single carrier type: in this mechanism they treat hopping of polarons and singlet bipolaron formation, in the presence of the random hyperfine fields of the hydrogen nuclei and an external magnetic field. Employing Monte Carlo simulations including on-site and longer range Coulomb repulsion they show how this leads to positive and negative magnetoresistance. This mechanism appears promising, since, amongst other things, this model is able to obtain the detailed line shape of the OMAR traces.
Conclusion
We studied magnetoconductivity and magnetoluminescence in sandwich devices made from films of a π-conjugated small molecule, namely Alq3. We observed magnetoconductance of more than 30% magnitude and magnetoluminescence of more than 50% magnitude in fields of 100 mT at room temperature. We also studied devices made with different electron-injecting electrode materials which result in a varying concentration of minority carriers in the devices. We showed that the maximum OMAR magnitude is almost independent of the minority carrier concentration. In our opinion, these observations demonstrate that OMAR cannot be explained by the relatively well-known excitonic magnetic-field effect models, but requires a new explanation based on a single carrier-type only.
Acknowledgment
This work was supported by NSF grant no ECS 07-25280.
References
- WohlgenanntMTandonKMazumdarSRamaseshaSVardenyZ V 2001 Nature 409 494 http://dx.doi.org/10.1038/35054025
- DediuVMurgiaMMatacottaF CTalianiCBarbaneraS 2002 Solid State Commun. 122 181 http://dx.doi.org/10.1016/S0038-1098(02)00090-X
- XiongZ HWuDVardenyZ VShiJ 2004 Nature 427 821 http://dx.doi.org/10.1038/nature02325
- FrancisT LMermerOVeeraraghavanGWohlgenanntM 2004 New J. Phys. 6 185 http://dx.doi.org/10.1088/1367-2630/6/1/185
- MermerOVeeraraghavanGFrancisTWohlgenanntM 2005 Solid State Commun. 134 631 http://dx.doi.org/10.1016/j.ssc.2005.02.044
- MermerOVeeraraghavanGFrancisTShengYNguyenD TWohlgenanntMKohlerAAl-SutiMKhanM 2005 Phys. Rev. B 72 205202 http://dx.doi.org/10.1103/PhysRevB.72.205202
- MermerOVeeraraghavanGFrancisT LWohlgenanntM 2003 Preprint cond-mat/0312204
- KalinowskiJCocchiMVirgiliDMarcoP DFattoriV 2003 Chem. Phys. Lett 380 710 http://dx.doi.org/10.1016/j.cplett.2003.09.086
- KalinowskiJCocchiMVirgiliDFattoriVMarcoP D 2004 Phys. Rev. B 70 205303 http://dx.doi.org/10.1103/PhysRevB.70.205303
- DavisA HBussmannK 2004 J. Vac. Sci. Technol. A 22 1885 http://dx.doi.org/10.1116/1.1759347
- YoshidaYFujiiAOzakiMYoshinoKFrankevichE L 2005 Mol. Cryst. Liquid Cryst. 426 19 http://dx.doi.org/10.1080/15421400590890642
- SalisGAlvaradoS FTschudyMBrunschwilerTAllenspachR 2004 Phys. Rev. B 70 085203 http://dx.doi.org/10.1103/PhysRevB.70.085203
- GarditzCMucklA GColleM 2005 J. Appl. Phys. 98 104507 http://dx.doi.org/10.1063/1.2132512
- PrigodinV NRajuN PPokhodnyaK IMillerJ SEpsteinA J 2002 Adv. Mat. 14 1230 http://dx.doi.org/10.1002/1521-4095(20020903)14:17<1230::AID-ADMA1230>3.0.CO;2-5
- RajuN PSavrinTPrigodinV NPokhodnyaK IMillerJ SEpsteinA J 2003 J. Appl. Phys. 93 6799 http://dx.doi.org/10.1063/1.1556120
- PrigodinVBergesonJLincolnDEpsteinA 2006 Synth. Metals 156 757 http://dx.doi.org/10.1016/j.synthmet.2006.04.010
- OdakaHOkimotoYYamadaTOkamotoHKawasakiMTokuraY 2006 Appl. Phys. Lett. 88 123501 http://dx.doi.org/10.1063/1.2185256
- IwasakiYOsasaTAsahiMMatsumuraMSakaguchiYSuzukiT 2006 Phys. Rev. B 74 195209 http://dx.doi.org/10.1103/PhysRevB.74.195209
- DesaiPShakyaPKreouzisTGillinW PMorleyN AGibbsM R J 2007 Phys. Rev. B 75 094423 http://dx.doi.org/10.1103/PhysRevB.75.094423
- HuBWuY Nat. Mater.
- BloomF LWagemansWKemerinkMKoopmansB Phys. Rev. Lett. at press
- XuRHusmannARosenbaumT FSaboungiM-LEnderbyJ ELittlewoodP B 1997 Nature 390 57 http://dx.doi.org/10.1038/36306
- ChienC LYangF YLiuKReichD HSearsonP C 2000 J. Appl. Phys. 87 4659 http://dx.doi.org/10.1063/1.373123
- NguyenT DShengYRybickiJVeeraraghavanGWohlgenanntM 2007 J. Mat. Chem. 17 1995 http://dx.doi.org/10.1039/b617541d
- NguyenT DShengYWohlgenanntMAnthopoulosT D Synth. Met. at press
- SteinerU EUlrichT 1989 Chem. Rev. 89 51 http://dx.doi.org/10.1021/cr00091a003
- FrankevichELymarevASokolikIKaraszFBlumstengelSBaughmanRHoerholdH 1992 Phys. Rev. B 46 9320 http://dx.doi.org/10.1103/PhysRevB.46.9320
- FrankevichEZakhidovAYoshinoKMaruyamaYYakushiK 1996 Phys. Rev. B 53 4498 http://dx.doi.org/10.1103/PhysRevB.53.4498
- IkomaTItoFOgiwaraTAkiyamaKTero-KubotaS 2005 Chem. Lett. 34 1424 http://dx.doi.org/10.1246/cl.2005.1424
- OgiwaraTIkomaTAkiyamaKTero-KubotaS 2005 Chem. Phys. Lett. 411 378 http://dx.doi.org/10.1016/j.cplett.2005.06.066
- ItoFIkomaTAkiyamaKWatanabeATero-KubotaS 2005 J. Phys. Chem. B 109 8707 http://dx.doi.org/10.1021/jp0453212
- BobbertP ANguyenT Dvan OostFKoopmansBWohlgenanntM Phys. Rev. Lett. at press
- ShengYNguyenT DVeeraraghavanGMermerOWohlgenanntMQiuSScherfU 2006 Phys. Rev. B 74 045213 http://dx.doi.org/10.1103/PhysRevB.74.045213
- BaldoM AO'BrienD FThompsonM EForrestS R 1999 Phys. Rev. B 60 14422 http://dx.doi.org/10.1103/PhysRevB.60.14422