Abstract
A TiCl4/ethylbenzoate/MgCl2 Ziegler–Natta catalyst was pretreated with chemically different poisoning compounds to investigate their effects on the catalyst activity and stereospecificity for propylene polymerization. The poisoning power on the activity was in the order of methanol > acetone > ethyl acetate. A kinetic analysis using the stopped-flow method revealed that addition of the poisoning materials decreased the activity through the reduction of the number of active sites, whereas the catalyst isospecificity was hardly affected by these materials.
Introduction
Highly active MgCl2-based Ziegler–Natta catalysts have been used for the majority of global polyolefin production during the past several decades since their discovery in the 1950s. Because of commercial use, much effort has devoted to ensuring a continuous improvement in the catalyst activity and stereospecificity through the discovery of increasingly suitable electron donors [Citation1]. The function of such donors in the MgCl2-supported catalysts is known to induce electronic and steric effects on active species by coordinating on the catalyst surface in the vicinity of the active species, leading to a marked increase in the stereospecificity of the catalyst through the selective poisoning of nonstereospecific sites or the transformation of aspecific sites to isospecific ones [Citation2–5]. The selective poisoning leads to a decrease in the number of active centers. On the other hand, the transformation is accompanied by an increase in the chain propagation rate, for which we reported that the chain propagation rate constant of isospecific sites was several times higher than that of aspecific sites [Citation6, Citation7]. Thus, the coordination of the electron donors can be regarded as affecting the catalyst activity in the directions of both activation and deactivation (normally the deactivation effect by the donors is stronger than the activation effect, leading to a decrease in the total activity). However, up to now, the fundamental mechanisms concerning the activation and deactivation behavior of the active sites have not yet been fully elucidated and are still open to discussion as a consequence of the complexity of the catalytic system, particularly in the absence of precise kinetic studies on the number of active sites and their chain propagation rate constants.
Recently, the stopped-flow technique has been demonstrated to be one of the most powerful methods for studying the kinetic and stereospecific natures of the active sites on the heterogeneous Ziegler–Natta catalysts used for propylene polymerization [Citation8]. The polymerization reaction proceeds within an extremely short period (ca. 0.2 s), exhibits quasi-living characteristics, where the states of the active sites are kept constant without time-dependent changes, and no side reactions including chain transfer and the deactivation of the active sites occur during polymerization. Because of these outstanding properties, direct information on the nature of active sites and the polymerization mechanism can be obtained [Citation6, Citation9–11].
In the present study, a TiCl4/ethylbenzoate/MgCl2 catalyst prepared via a chemical route from magnesium ethoxide was pretreated by several chemically different poisoning materials such as an alcohol, ketone and ester. Simple and sterically small molecules were selected as examples of poisoning compounds to clarify the mechanism of site deactivation without notable steric effects from these poisoning materials, which may not generate isospecific sites or even transforming aspecific sites into isospecific ones. A precise kinetic study on the effect of the poisoning materials was carried out using the stopped-flow polymerization combined with gel permeation chromatography (GPC) analysis.
Experimental
Chemicals
Research-grade propylene of (donated by Chisso Corp.) was used without further purification. Methanol, acetone and ethyl acetate were used as the representative alcohol, ketone and ester, respectively, which have the least steric hindrance to minimize steric effects from the poisoning materials. Ethyl benzoate (EB) as an internal donor, and anhydrous-grade methanol, acetone and ethyl acetate were used as the poisoning materials (purchased from Wako Pure Chemical Industries, Ltd.) after dehydration using a molecular sieve. Anhydrous magnesium ethoxide (Mg(OEt)2), titanium tetrachloride (TiCl4) (both donated by Toho Titanium Co., Ltd.), triisobutylaluminium (TIBA, donated by Tosoh Akzo Corp.) and nitrogen (purchased from Uno Sanso Co.) were used without further purification. Heptane and toluene were used as solvents and were purified by passing them through the 13X column of a molecular sieve.
Catalyst preparation
In the current study, a monoester-type catalyst TiCl4/EB/MgCl2 was prepared by a chemical reaction without mechanical treatment (ball milling). TiCl4 was reacted with a mixture of (Mg(OEt)2) and EB in the following procedure: (Mg(OEt)2) (40 g) and TiCl4 (80 ml) in toluene (320 ml) were heated to 90 °C, mixed with EB (12 ml) and reacted at 90 °C for 2 h. The mixture was washed with toluene and treated again with TiCl4 (80 ml) in toluene (320 ml) at 90 °C for 2 h. Finally, the catalyst obtained was washed with heptane several times and then used as to form a heptane slurry. The Ti content was determined by titration to be 3 wt.%.
Slurry propylene polymerization
Slurry polymerization was conducted to obtain preliminary information on the deactivating effect of the poisoning materials. It was performed in a 300 ml round-bottom glass reactor equipped with a magnetic stirrer. The required amounts of heptane (200 ml) and catalyst (0.095 mmol) were added to the reactor and then heated to 30 °C along with saturated propylene at atmospheric pressure. A specific amount of a poisoning material was introduced into the catalyst vessel and reacted with the catalyst for 1 min followed by the injection of TIBA (Al/Ti molar ratio: 30) to start polymerization. After 10 min, the reaction was terminated by venting the reactor and by adding acidic ethanol. The product was washed with water several times and then evaporated and dried under vacuum at 60 °C for 6 h.
Stopped-flow polymerization
The stopped-flow polymerization of propylene and the determination of the kinetic parameters were carried out in accordance with the method reported previously [Citation6, Citation8, Citation12–15]. The propylene polymerization was typically performed with a catalyst (ca. 1.0 g) and TIBA (14 mmol, Al/Ti molar ratio: 30) in heptane at 30 °C for 0.1, 0.15 and 0.18 s. A heptane slurry (100 ml) of the catalyst and a solution of TIBA in heptane (100 ml) saturated with 1 atm of propylene were placed into two vessels. Methanol as a poisoning material was introduced into the catalyst vessel and reacted with the catalyst for 1 min before starting polymerization (poisoning material/Ti molar ratio=0.1).
The propagation rate constant kp and active-site concentration [C∗] were determined by the following equations [Citation15];
(1)
(2)
where
and Y are the number-average molecular weight of the polymer, the molecular weight of the monomer, the monomer concentration, the polymerization time, the chain-transfer rate constant and the polymer yield, respectively.
GPC characterization
The molecular weight and its distribution for the polypropylene obtained in this study were determined by gel permeation chromatography (GPC, Senshu SSC-7100) with polystyrene gel columns (Tosoh TSK-GEL G3000HHR and TSK-GEL G5000HHR) at 140 °C using o-dichlorobenzene (ODCB) as a solvent.
13C NMR spectroscopy
13C NMR spectra were recorded on a Varian Gemini 300 spectrometer at 120 °C using 20% (w/v) solutions in 1,2,4-trichlorobenzene. 10% (v/v) benzene- d6 was added for use as the internal lock, and hexamethyldisiloxane was used as the reference (internal chemical shift).
Results and discussion
In the first series of propylene polymerizations, slurry polymerizations were conducted after pretreatment of the catalyst with the poisoning materials for 1 min. The results are listed in table 1. At a poisoning material/Ti molar ratio of 0.1 (entries 1–4), the catalyst activity decreased substantially in the following order: ethyl acetate>acetone>methanol, while there was no significant change in the mmmm pentad. The results clearly demonstrated that the catalytic activity was greatly affected by the chemical nature of the poisoning materials, and that all the materials poisoned the active sites independently of their stereospecificity.
Activity and tacticity of the PP without and with poisoning materialsa.
In the case of methanol, it is generally accepted that TiCl4 readily reacts with alcohol to produce HCl and alkoxide complexes that are inactive for propylene polymerization [Citation16]. In addition, HCl reacts with TIBA to reduce its activation power, and the titanium chloride alkoxides produced on the catalyst surface might have a negative effect on the catalyst activity [Citation16]. Ethyl acetate, the donor might coordinate on the MgCl2 support though its oxygen atom, similarly to monoester-type donors such as EB. Therefore, the catalytic activity was found to be little affected. Acetone might interact with the catalyst surface in a similar fashion to ethyl acetate, and it also reacted with the Ti-alkyl or Ti-polymer to form bulky alkoxy-Ti chlorides [Citation17], resulting in an apparent decrease in catalytic activity.
In the second series of polymerizations, kinetic studies were carried out using a combination of the stopped-flow technique and GPC analysis with the aim of quantifying the effect of the poisoning materials in terms of the number of active sites and the chain propagation rate constant. Again, the catalysts were pretreated with methanol for 1 min before starting the polymerization because methanol showed the most prominent deactivation effect. Figure shows the dependence of the polymer yields on the polymerization time with and without methanol. A linear correlation was observed within 0.2 s indicating that the catalyst possessed constant activity without deactivation and late activation even after adding methanol, suggesting that the reaction of methanol with the catalyst was completed within the 1 min pretreatment. From figure , the two molecular weights are proportional to the polymerization time, which confirms the quasi-living nature of the polymerization with and without methanol. Furthermore, the molecular weight was unchanged after the pretreatment with methanol.
Figure 1 Yield of the polypropylene plotted for the polymerization time without and with a methanol pretreatment.
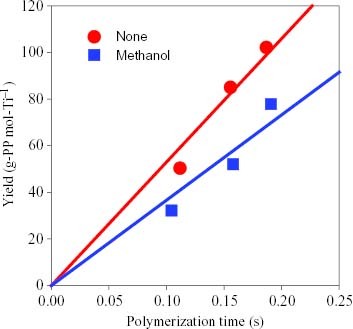
Figure 2 Number-average molecular weight
of the polypropylene without and with methanol pretreatment plotted against polymerization time.
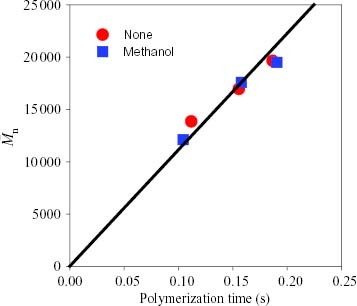
From the kinetic parameters as reported in table 2, the value of kp was hardly changed while the number of [C∗] markedly decreased from 0.40 to 0.28 mol.%, i.e., there was a 30% reduction compared with the reference polymerization without the pretreatment. It is well-known that heterogeneous Ziegler–Natta catalysts contain a great variety of active sites and the chain propagation rate constant of different types of active sites significantly increases as their isospecificity increases [Citation18, Citation19]. In this regard, the value of kp could have only changed in the case that there was some selective poisoning of the different types of active sites. For example, the selective poisoning of aspecific or isospecific sites must lead to a higher or lower kp, respectively. Thus, a constant value of kp was indicative of nonselective poisoning, which was supported by the nearly constant mmmm pentad in the slurry polymerization, as shown in table 1. Using the method of the stopped-flow polymerization, we were able to attribute the poisoning effect of methanol on the catalyst performance to only the decrease in the number of active centers.
Kinetic parameters for propylene polymerization obtained without and with methanola.
Finally, the result of stopped-flow polymerization suggested that the active-site concentration was sensitive to the methanol. The reaction taking place at a methanol/Ti molar ratio of 0.1 would result in the reduction of the activity by about 10% if TiCl3OCH3, the product of the reaction, was not active in the polymerization. However, the actual observed decrease in the activity was as large as 30%, which might be explained by the same reason as for the corresponding result of the slurry polymerization, i.e., the negative effect of the bulky alkoxy-Ti chlorides [Citation17].
Conclusions
We examined the effects of poisoning materials on the catalytic performance of a MgCl2-based Ziegler–Natta catalyst using methanol, acetone, and ethyl acetate as the least bulky poisoning agents. Methanol exhibited the strongest deactivation power followed by acetone and ethyl acetate due to its highest reactivity in forming titanium chloride alkoxide, which was inactive for polymerization. A precise kinetic study on the effect of the methanol pretreatment using the stopped-flow method revealed that the deactivation was only due to the decrease in the number of active centers caused by methanol, was insensitive to the type of active sites, and maintained a constant kp value and the stereoregularity of the obtained polymers both without and with methanol pretreatment.
Acknowledgments
The authors gratefully acknowledge the Thailand Research Fund (TRF) and the Royal Golden Jubilee program for the financial support. The authors also thank Toho Titanium Co., Ltd., Chisso Corp., Asahi Chemical Industry Co., Ltd., Mitsubishi Chemical Corp. and Tosoh Akzo Corp., for their support.
References
- BoorJ 1979 Ziegler–Natta Catalysts and Polymerizations New York Academic
- SogaKSanoTYamamotoKShionoT 1982 Chem. Lett. 11 425 http://dx.doi.org/10.1246/cl.1982.425
- KashiwaN 1983 Transition Metal Catalyzed Polymerization: Alkenes and Dienes R PQuirk New York Harwood Academic p 379
- SacchiM CTrittoILocatelliP 1988 Eur. Polym. J. 24 137 http://dx.doi.org/10.1016/0014-3057(88)90140-1
- TrittoISacchiM CLocatelliPZannoniG 1988 Macromolecules 21 384 http://dx.doi.org/10.1021/ma00180a016
- MatsuokaHLiuBNakataniHTeranoM 2001 Macromol. Rapid Commun. 2 326 http://dx.doi.org/10.1002/1521-3927(20010301)22:5<326::AID-MARC326>3.0.CO;2-G
- SogaKOhgizawaMShionoT 1989 Macromol. Chem. Rapid Commun. 10 503 http://dx.doi.org/10.1002/marc.1989.030100911
- LiuBMatsuokaHTeranoM 2001 Macromol. Rapid Commun. 22 1 http://dx.doi.org/10.1002/1521-3927(20010101)22:1<1::AID-MARC1>3.0.CO;2-T
- LiuBMatsuokaHTeranoM 2001 Macromol. Symp. 165 3 http://dx.doi.org/10.1002/1521-3900(200103)165:1<3::AID-MASY3>3.0.CO;2-R
- MatsuokaHLiuBNakataniHNishiyamaITeranoM 2002 Polym. Int. 51 781 http://dx.doi.org/10.1002/pi.942
- NishiyamaILiuBMatsuokaHNakataniHTeranoM 2003 Macromol. Symp. 193 71 http://dx.doi.org/10.1002/masy.200390065
- TeranoMKataokaTKeiiT 1989 J. Mol. Catal. 56 203 http://dx.doi.org/10.1016/0304-5102(89)80184-1
- MoriHSaitoHTeranoM 1998 Macromol. Chem. Phys. 199 55
- MoriHSaitoHYamahiroMKonoHTeranoM 1998 Macromol. Chem. Phys. 199 613 http://dx.doi.org/10.1002/(SICI)1521-3935(19980401)199:4<613::AID-MACP613>3.0.CO;2-H
- MoriHTeranoM 1997 Trends Polym. Sci. 5 314
- GaroffTLiskolaESormunenP 1987 Transition Metals and Organometallics as Catalysts for Olefin Polymerization WKaminsky HSinn BerlinSpringer p 197
- ZambelliAOlivaLAmmendolaP 1986 Gazz. Chim. It. 259 116
- MoriHTashinoKTeranoM 1996 Macromol. Chem. Phys. 197 895 http://dx.doi.org/10.1002/macp.1996.021970311
- SogaKOhgizawaMShionoT 1984 Macromol. Chem. Rapid Commun. 10 503