Abstract
A glycopolymer carrying trehalose was found to suppress the formation of amyloid fibrils from the amyloid β peptide (1–42) (Aβ), as evaluated by thioflavin T assay and atomic force microscopy. Glycopolymers carrying sugar alcohols also changed the aggregation properties of Aβ, and the inhibitory effect depended on the type of sugar and alkyl side chain. Neutralization activity was confirmed by in vitro assay using HeLa cells. The glycopolymer carrying trehalose strongly inhibited amyloid formation and neutralized cytotoxicity.
Introduction
Alzheimer's disease (AD) is a progressive dementia, and the number of patients increases exponentially with life expectancy [Citation1]. The development of an effective inhibitor of AD is urgently required. AD is characterized neuropathologically by extracellular deposition of amyloid senile plaques and neurofibrillary tangles in vulnerable AD brain regions. These plaques are primarily composed of fibrils of the amyloid β (Aβ) peptide, a small peptide composed of 39–43 amino acids [Citation2]. The most abundant forms are 40 and 42 amino acids in length [Citation3, Citation4]. These types of peptide are cleaved from a large protein called the amyloid precursor protein (APP) by a secretase enzyme [Citation5]. Production of Aβs is a normal occurrence, although its function remains unclarified. However, in AD patients the peptide forms ordered fibrillar aggregates. This process involves a secondary structure transition from a disordered random structure to an ordered β-sheet conformation [Citation6].
Therefore, it is required to develop medicinal compounds that inhibit Aβ aggregation. Preventing Aβ aggregation can be accomplished using various compounds such as dopamine [Citation7] and heparin [Citation8]. All of these agents work through similar mechanisms, reducing cytotoxicity by inhibiting and delaying aggregation of Aβ [Citation9].
A nonreducing disaccharide with an α(1-1) linkage, trehalose, is the focus of much attention in view of its inhibitory effect on protein aggregation [Citation10]. It has been reported that trehalose inhibits Huntington's disease in vivo [Citation11] and amyloid formation of proteins (insulin [Citation12] and Aβs [Citation9]) in vitro owing to the hydration of proteins by the water-like hydrogen bond. Because the inhibitory effect of trehalose is not sufficient for medicinal application, an ingenious modification is required to amplify its activity.
The biological abilities of saccharides can be amplified by multivalency [Citation13]. Many groups have reported the multivalent effect and the generation of the multivalent compounds such as glycopeptides [Citation14], glycocalixarenes [Citation15], glycodendrimers [Citation16], and glycopolymers [Citation17]. In particular, glycopolymers carrying a saccharide at the side chain exhibit a large multivalent effect and applicability as biomaterials.
We have studied various glycopolymers with biorecognition abilities, such as lectin recognition [Citation18]; hepatocyte culture; and amyloid inhibitor [Citation19]. In this report, we investigated glycopolymers carrying trehalose to generate an efficient inhibitor of Aβ aggregation in terms of the effect of amplification of trehalose hydration properties. The glycopolymers carrying trehalose and sugar alochols (lactitol and maltitol) were synthesized, and the inhibitory effect on amyloid aggregation was examined in terms of the saccharide structure and amphiphilicity of the glycopolymers.
Experimental procedure
Materials
The following reagents were used as received: amyloid β-protein (Aβ(1–42)) (Bachem AG, Switzerland), divinyl sebacate (Polyscience Inc., US), trehalose (Kanto Chemical, Japan), lactitol (Sigma-Aldrich, US), Dulbecco's modified Eagle's medium (DMEM), fetal bovine serum (FBS) (Invitrogen, US), thioflavin T (ThT), Omnipore membrane filter (0.45 μm, Millipore, USA), 3-[4,5-dimethylthiazol-2-yl]-2,5-diphenyltetrazolium bromide (MTT) (Wako Chemical, Japan), 8-anilino-1-naphtalenesulfonic acid ammonium salt (ANS), maltitol, and divinyl adipate(Tokyo Kasei, Japan). Lipase from Candida antarctica (CA) was kindly donated by Novo Nordisk Bioindustry Ltd.
Characterization
1H-NMR (300 MHz) and 13C-NMR(75 MHz) spectra were recorded on Varian Gemini-300, and the spectra were measured in D2O at room temperature. Gel permeation chromatography (GPC) was conducted using a JASCO 800 high-performance liquid chromatography instrument, on a Shodex SB-804HQ column with PBS(-) as an eluent, and molecular weight was determined using a pullulan standard. Fluorescence spectra were measured on FP-6500 (JASCO, Japan) at room temperature. The diameters of the glycopolymer were measured by dynamic light scattering (DLS) with Nano-ZS (Malvern, UK). Atomic force microscopy (AFM) was performed using SPA400 (Seiko Instrument Inc., Japan).
Syntheses of glycopolymers
The glycopolymers were synthesized by enzymatic esterification of lipase CA and radical polymerization according to the literature [Citation20]. Syntheses of saccharide vinyl esters were confirmed from NMR spectra. The molecular structures and molecular weights of the polymers are summarized in figure and table 1, respectively.
Molecular weights of the glycopolymers.a
In vitro amyloid formation of Aβ(1–42) [Citation19]
Aβ(1–42) was dissolved in 0.02% ammonia solution at a concentration of 200 μM, and any aggregates formed were removed by centrifugation using a CS 120 FX (Hitachi, Tokyo, Japan) at 16 000 xg for 30 min at 4 °C. Next, the supernatant was mixed with phosphate buffer (20 mM phosphate buffer, pH 7.4, and 100 mM NaCl) to a final peptide concentration of 20 μM. The peptide solution was incubated with each glycopolymer at 37 °C.
ThT fluorescence assay
Amyloid fibril formation was evaluated on the basis of fluorescence emission of ThT using a 3-mm light-path quartz cuvette. Aβ(1–42) (20 μM) was incubated at 37 °C in 50 mM phosphate buffer, 100 mM NaCl, at pH 7.4, 100 μM glycopolymer, and 50 μL of Aβ solution was periodically added to 300 μL of aqueous solution of 5 μL of ThT (50 μM) in 50 mM glycine–NaOH. ThT fluorescence intensity was measured at an excitation wavelength of 440 nm and an emission wavelength of 482 nm. The fluorescence intensity of the control sample after 12 h incubation without a glycopolymer was used as the standard (1.0). Fluorescence intensity was taken as the average of at least three samples.
AFM measurements
Aβ(1–42) solution (20 μl) was incubated with 100 μM glycopolymer in phosphate buffer (50 mM phosphate buffer, 100 mM NaCl, at pH 7.4). An aliquot of a sample solution (5 μl) was placed on freshly cleaved mica, rinsed with deionized water, and dried. The resultant sample was scanned using SPA400.
Neutralization of Aβ(1–42) with a glycopolymer
Neutralization activity of a glycopolymer (2) was examined in vitro by HeLa cell cytotoxicity assay. HeLa cells were cultured in DMEM containing 10% FBS at 37 °C incubation in 5% CO2. An aliquot containing 1×103 cells in 100 μl of DMEM was transferred to each well of a 96-well plate coated with collagen, and DMEM was removed. Aβ(1–42) solution (30 μl, 20 μM) was preincubated with 100 μM 2 at 37 °C for 8 h in 20 mM HEPES, 100 mM NaCl, at pH 7.4, and 30 μl of the medium was added to each well. The mixed sample solution was incubated for 4 h at 37 °C. The plate was centrifuged (DU-600), and the medium was removed using an aspirator. A 100 μl aliquot of MTT solution was added to each well. The absorbance at 570 and 650 nm was measured using a plate reader. The difference in absorbance between 570 and 650 nm was plotted to measure cell viability. The absorbance of HeLa cells alone was used as the standard (100%). The experiments were performed using at least three samples.
Results
Inhibitory effect of glycopolymers on Aβ(1–42) aggregation
The aggregation behavior of Aβ(1–42) was investigated by adding trehalose additives (1, 2, and 3) (figure ). The aggregation behavior of Aβ was examined on the basis of fluorescence intensity of ThT. The fluorescence intensity of Aβ without additives (control) gradually increased, suggesting amyloid formation. The fluorescence intensity after 12 h incubation was set as 1.0, and fluorescence intensities in the presence of trehalose additives were compared with that of the standard. The addition of trehalose attenuated the aggregation properties of the peptide, in which trehalose decreased fluorescence intensity up to 60 %.
Figure 2 Effect of trehalose (1) and polyvalent trehalose (2) on Aβ (1–42) aggregation. Control (∘), 1 (•) and 2 (□).
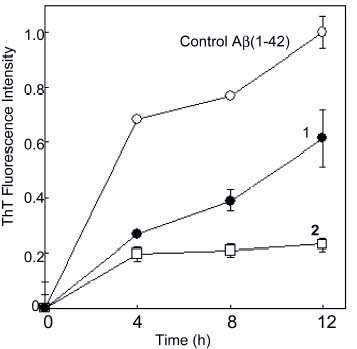
The addition of polyvalent trehalose with a short alkyl chain (2, poly(vinyladipoyl trehalose)) markedly decreased the fluorescent intensity of ThT. The fluorescence intensity of 2 was only 20% of that of control. The efficacy lasted for a long time over 15 h. These results indicate that the inhibitory effect of trehalose was amplified by multivalency. On the other hand, the polyvalent trehalose with a longer alkyl side chain (3, poly(vinylsebacoyl trehalose)) did not inhibit amyloid formation but rather induced it.
Polyvalent sugar alcohols (4–7) were also investigated for their inhibitory effect on amyloid formation (figure ). Poly(vinyladipoyl lactitol) (4) strongly inhibited amyloid formation from 0 to 8 h, but amyloid formation rapidly proceeded after 8 h incubation. Poly(vinylsebacoyl lactitol) (5) induced amyloid formation. Polyvalent maltitol(6 (poly(vinyladipoyl maltitol) and 7 (poly(vinylsebacoyl maltitol)) also changed the time course curve of ThT fluorescence intensity, and the effects on amyloid formation were much smaller than those of polyvalent trehalose (2 and 3) and polyvalent maltitol (6 and 7).
Figure 3 Effect of glycopolymer (3, 4, 5 and 6) on Aβ(1–42) aggregation. Control (∘), 3 (▴), 4 (▪), 5 (X), 6 (✦), and 7 (◊).
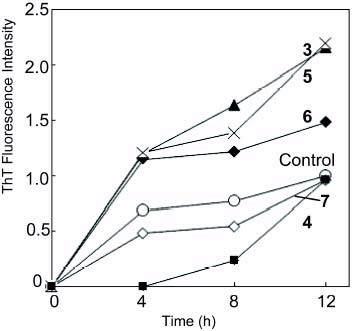
Polyvalent trehalose with a short alkyl chain (2) showed the strongest inhibition effect on Aβ(1–42) aggregation.
Morphology of Aβ(1–42) observed by AFM
The inhibitory effect on amyloid formation was also investigated by AFM (figure ). Aβ(1–42) formed amyloid fibrils of 30–120 nm diameter and 300 nm-2 μm length owing to aggregation properties. The addition of 2 totally changed the morphology, and amyloid fibrils were not observed. Nonuniform round aggregates of 40–120 nm diameter were observed. The addition of 3 also changed the morphology into round aggregates, but fibril formation with 30–150 nm in width was still observed, which corresponded to the ThT fluorescence intensity. The round aggregates with 2 and 3 resembled the morphology of the glycopolymer itself. Therefore, the round aggregates in figures (b) and (c) were a mixture of Aβ and glycopolymers. The glycopolymers had affinities with Aβ(1–42) owing to trehalose and the amphiphilic properties of the polymer, which induced the formation of round aggregates.
Neutralization effect of glycopolymer with Aβ(1–42)
Polyvalent trehalose of 2 was evaluated for its activity of neutralizing Aβ(1–42) in HeLa cells (figure ). Aβ was incubated in advance and added to HeLa cells. When the cells were incubated with Aβ alone, Aβ exhibited cytotoxicity reducing MTT activity by about 50%. On the other hand, coincubation of Aβ with a polyvalent trehalose (2) decreased cytotoxicity, indicating protection against Aβ(1–42). The cell survival rate was nearly 100% compared with that without additions. Moreover, the incubation with 2 alone did not show cytotoxicity. These results showed that polyvalent trehalose can be an effective inhibitor of Aβ aggregation.
Amphiphilic properties of glycopolymers
The glycopolymers formed a self-assembling structure in aqueous solution owing to the amphiphilic structure of the polymer, and the size of the glycopolymer in aqueous solution was measured by DLS (table 2). The diameters of the polyvalent saccharides (2–7) depended on alkyl chain length and the type of saccharides. The diameters of 2 and 4 were small, approximately 30–40 nm, suggesting the compaction of the polymer. Other glycopolymers (3, 5, 6 and 7) formed larger aggregates with diameters over 100 nm. The order of the diameters is 4<2≪5≪3, 6≪7.
The diameters of glycopolymers in aqueous solution.
The inhibitory effect on amyloid formation determined from ThT fluorescence intensity was plotted against the diameter of the glycopolymers (figure ). The glycopolymers with small diameters (0–50 nm, 2 and 4) inhibited amyloid formation, and ThT fluorescence intensities in the presence of the glycopolymers were below 0.4. The glycopolymer with medium diameters (approximately 100 nm) of 3, 5 and 6 rather enhanced amyloid fibril formation with ThT fluorescence intensities above 1.0. The glycopolymer with a larger diameter (over 400 nm) (7) did not show a marked difference from the control without sugar additives.
Figure 6 Correlation between diameter of glycopolymer and aggregation properties of Aβ(1–42). The diameter of the glycopolymer was plotted against ThT fluorescence intensity after 8 h incubation.
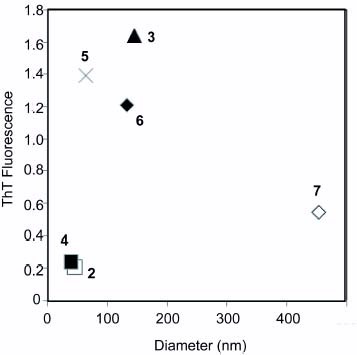
The amphiphilic property of the glycopolymer (2) was also investigated using a hydrophobic indicator of 1-anilinonaphthalene-8-sulfonic acid (ANS). The fluorescence intensity of ANS showed rather hydrophobic properties with a peak of 470 nm (figure ).
Discussion
Glycopolymer additives changed the amyloid formation of Aβ(1–42). The extents of inhibitory effect were in the order of 2>4≫7≫6>5>3. Glycopolymers 2, 4 and 7 inhibited amyloidosis, but glycopolymers 3, 5 and 6 rather induced it. The best inhibitor was polyvalent trehalose of 2. These results indicate that the inhibitory effect of the saccharides was amplified by a multivalency-like protein-saccharide interaction. Trehalose is a unique disaccharide with a clam shell structure [Citation21], which induces the water-like hydrogen bonding with lipids [Citation22] and proteins [Citation23] to preserve the biological activities. The hydrogen bonding formation of trehalose with water clusters was also reported [Citation24]. The biological activities of trehalose are different from those of other saccharides with open structures such as lactose and maltose. Although the modification of trehalose might partially change the structure, the multivalent trehalose of 2 did not cause the loss of the ability of hydrogen-bonding formation with ambient biomolecules and water. The glycopolymer 2 rather induced the alignment of trehalose to facilitate the hydrogen bonding with Aβ(1–42), resulting in an efficient inhibitory effect.
At the same time the inhibitory effect was greatly affected by the alkyl chain length of the glycopolymers. For example, polyvalent trehalose with a short alkyl chain (2) showed a strong inhibitory effect, but polyvalent trehalose with a longer alkyl chain (3) induced amyloid formation. It has been reported that amyloid formation is induced by surfactants of amphiphilic molecules [Citation25–27]. Not only the saccharide structure of the polymer but also amphiphilicity plays important roles in the amyloid formation and inhibition. Interestingly, the inhibitory effect on amyloid formation correlated to the diameter of the glycopolymers owing to amphiphilicity. The glycopolymers with small diameters(2 and 4) reflected the hydration properties of saccharides and showed the effective inhibition activity. The glycopolymer 4 did not inhibit the aggregation of Aβ for a long time (over 8 h), but the polymer inhibited the aggregation until 8 h of incubation. The glycopolymers with diameters of about 100 nm (3, 5 and 6) showed a hydrophobic cavity inside the polymer micelle, and the hydrophobic interaction with Aβ was stimulated, resulting in the aggregation of the peptide. The glycopolymer with a larger diameter (7) did not exhibit the markedly strong inhibitory effect on Aβ. The amphiphilic properties of 2 were also investigated using a hydrophobic indicator of ANS. Although glycopolymer 2 showed the strongest inhibitory effect on Aβ aggregation owing to the hydration properties of trehalose, the fluorescence intensity of ANS showed rather hydrophobic properties with a peak of 470 nm. These data indicate that the glycopolymer has a densely packed trehalose layer at the periphery for the hydration of Aβ. The amphiphilic structures of the glycopolymers are still under investigation to develop drugs for the inhibition of Aβ aggregation.
Trehalose preserves biological activities owing to its special physical chemical properties of hydration. Our results indicate that the polyvalent trehalose can amplify the hydration properties, which is utilized for not only the inhibition of Aβ aggregation, but also for treatment of other conformation diseases. In addition, the glycopolymers with trehalose show amphiphilic properties, which can be utilized as a novel surfactant or an interface-reforming reagent.
Conclusions
The glycopolymers were analyzed as an amyloid inhibitor. The glycopolymer with trehalose and sugar alcohols changed the aggregation properties of Aβ(1–42). The inhibitory effect on Aβ aggregation depended on the type of saccharide and amphiphilicity. Poly(vinyladipoyl trehalose) showed the strongest inhibitory effect on amyloid formation, and the morphology of Aβ changed from fibrils to round aggregates. The cytotoxicity of Aβ was completely neutralized by the polymer added. The glycopolymer with trehalose was a novel inhibitor of Aβ aggregation.
Acknowledgments
This work was supported by a Grant-in-Aid for Young Scientists (B), JST seeds, and Shibuya Foundation.
References
- SelkoeD J 2001 Physiol. Rev. 81 741
- SelkoeD J 1994 Annu. Rev. Nuerosci. 17 489 http://dx.doi.org/10.1146/annurev.ne.17.030194.002421
- GlennerG GWongC W 1984 Biochem. Biophys. Res. Commun. 122 1131 http://dx.doi.org/10.1016/0006-291X(84)91209-9
- GlennerG GWongC W 1984 Biochem. Biophys. Res. Commun. 120 885 http://dx.doi.org/10.1016/S0006-291X(84)80190-4
- SinhaSLieberburI 1999 Proc. Natl Acad. Sci. USA 96 11049 http://dx.doi.org/10.1073/pnas.96.20.11049
- ThompsonL K 2003 Proc. Natl Acad. Sci. USA 100 383 http://dx.doi.org/10.1073/pnas.0337745100
- OpazoC et al 2002 J. Biol. Chem. 277 40302 http://dx.doi.org/10.1074/jbc.M206428200
- YoshiikeYTanemuraKMurayamaOAkagiTMurayamaMSatoSSunXTanakaNTakashimaA 2001 J. Biol. Chem. 276 32293 http://dx.doi.org/10.1074/jbc.M010706200
- JanusC 2003 CNS Drugs 17 457 http://dx.doi.org/10.2165/00023210-200317070-00001
- LiuRBarkhordarianHEmadiSParkC BSierksM R 2005 Neurobiol. Disease 20 74 http://dx.doi.org/10.1016/j.nbd.2005.02.003
- TanakaMMachidaYNiuSIkedaTJaneN RDoiHKurosawaMNekookiMNukinaN 2004 Nat. Med. 10 148 http://dx.doi.org/10.1038/nm985
- AroraAHaCParkC-B 2004 FEBS Lett. 564 121 http://dx.doi.org/10.1016/S0014-5793(04)00326-6
- MammenMChoiS-KWhitesidesG M 1998 Angew. Chem. Int. Ed. 37 2754 http://dx.doi.org/10.1002/(SICI)1521-3773(19981102)37:20<2754::AID-ANIE2754>3.0.CO;2-3
- LundquistJ JDebenhamS DTooneE J 2000 J. Org. Chem. 65 8245 http://dx.doi.org/10.1021/jo000943e
- DondroniAKlebanMHuXMarraABanksH D 2002 J. Org. Chem. 67 4722 http://dx.doi.org/10.1021/jo020178z
- RoyRKimJ M 1999 Angew. Chem. Int. Ed. 38 369 http://dx.doi.org/10.1002/(SICI)1521-3773(19990201)38:3<369::AID-ANIE369>3.0.CO;2-1
- KobayashiAAkaikeTKobayashiKSumitomoH 1986 Makromol. Chem. Rapid. Commun. 7 645 http://dx.doi.org/10.1002/marc.1986.030071005
- MiuraYIkedaTKobayashiK 2003 Biomacromolecules 4 410 http://dx.doi.org/10.1021/bm025714b
- MiuraYYasudaYYamamotoKKoikeMNishidaYKobayashiK 2007 Biomacromolecules 8 2129 http://dx.doi.org/10.1021/bm0701402
- MiuraYWadaNNishidaYMoriHKobayashiK 2004 J. Polym. Sci. A Polym. Chem. 42 4598 http://dx.doi.org/10.1002/pola.20385
- BrownG MRohreD CBerkingBBeeversC AGouldR OSimpsonR 1972 Acta Crystallogr. B 28 3145 http://dx.doi.org/10.1107/S0567740872007654
- LambruschiniCReliniARidiACordoneLGliozziA 2000 Langmuir 16 5467 http://dx.doi.org/10.1021/la991641e
- Sola-PennaMMeyer-FernandesJ R 1998 Arc. Biochem. Biophys. 360 10 http://dx.doi.org/10.1006/abbi.1998.0906
- PagnottaS ERicciM ABruniFMcLainSMagazuS 2008 Chem. Phys. 345 159 http://dx.doi.org/10.1016/j.chemphys.2007.07.052
- LiYGaoMWangY 2006 J. Phys. Chem. B 110 18040 http://dx.doi.org/10.1021/jp063176h
- CaoMHanYWangJWangY 2007 J. Phys. Chem. B 111 13436 http://dx.doi.org/10.1021/jp075271b
- HamillA CWangS CLeeC T Jr 2007 Biochemistry 46 7694 http://dx.doi.org/10.1021/bi700233k