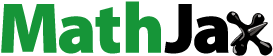
Abstract
Purpose
The aim was to evaluate the repeatability of dynamic measurement of the accommodative stimulus–response curve () at three different dioptric speeds using a modified instrument and its agreement with two other methods.
Methods
Twenty‐nine adults (23.5 ± 2.0-years) were enrolled in the study. ASRC was measured monocularly using three methods: dynamic and static measurement using a motorised Badal system mounted on an open‐field auto‐refractor (WAM‐5500, Grand Seiko Co., Ltd, Japan) and the minus lens technique. Dynamic measurements were conducted at three dioptric stimulus speeds to simulate continuous stimuli for ASRC (0.25, 0.40 and 0.55 D/s), with three repetitions for each speed. All three types of ASRCs were fitted with third‐degree polynomial equations. The slope and objective accommodative amplitude of the ASRC were analysed.
Results
The repeatability of objective accommodative amplitude worsened as the speed of the stimuli increased. The repeatability of the slope was best at a speed of 0.40 D/s and worst at 0.55 D/s. The measurement method significantly influenced the objective accommodative amplitude values and slope (both, p < 0.001). The minus lens technique yielded the highest amplitude of accommodation (6.21 ± 0.84 D) and steepest slope (1.11 ± 0.14), followed by the static Badal method (5.60 ± 0.83 D and 0.89 ± 0.09 D). The objective accommodative amplitude decreased with increasing speed during dynamic measurements. There was no difference between the slopes at 0.25 D and 0.40 D/s (p > 0.05) and the slope was lowest at 0.55 D/s.
Conclusion
values are method‐dependent and the significant differences between three methods used to determine the based on slope and accommodative amplitude indicate that these methods are non‐interchangeable. Using dynamic measurements, accommodative behaviour varies with the speed of dioptric‐change of the stimulus. A speed of 0.40 D/s appears to be the best compromise in terms of time, results and repeatability for dynamic measurement.
The accommodative stimulus–response curve (ASRC) describes the accommodative response as a function of the accommodative stimulus. This curve can be used to derive important parameters of accommodation, such as the slopeCitation1976 of the accommodative response, the accommodative error index,Citation2013 which is calculated from the area between the regression line for the linear part of the accommodative curve and the 1:1 line divided by the correlation coefficient and the accommodative error area,Citation2013 which is the area between the accommodative curve including the non‐linear region and the 1:1 line. These parameters also provide insight into potential relationships between accommodation and ocular anomalies, such as amblyopiaCitation1984 and myopia.Citation1986
Traditionally, three main methods have been used to measure the ASRC: decreasing distance series, positive lens series and negative lens series at distance. Previous studiesCitation1993 have shown that these three methods yield different ASRCs, with higher responses obtained with the positive lens compared to the negative lens method. When applying these three methods, the target is not adjusted for lens‐induced magnification and minification or changes in angular size due to changes in the fixation distance. Several authorsCitation2013 have used a Badal stimulator, which maintains a constant target size and minimises proximal accommodation; however, few studiesCitation2009 have determined the ASRC using the minus lens method with a near target despite poor stimulation of the accommodative response by negative lenses at distance. Usually, discrete 1.00 D stimulus intervals are used to save time and therefore, the fitted curve does not reflect the entire accommodative status because there are gaps between the measured stimuli.
The slope of the accommodative response has been widely used to characterise the entire ASRC. Various studiesCitation1993 have used different approaches to investigate the relationship between the ASRC slope and different refractive groups. Results are affected by various factors, such as the target's luminance,Citation1976 contrastCitation1985 and spatial frequency.Citation1983 Moreover, the ASRC slope is method‐dependent and is flattest using the negative lens method at distance, suggesting that negative‐lens‐induced blur is the least effective at driving the accommodative response. As slope is an important parameter of the accommodative function, it is critical for clinicians to know its repeatability; however, there are no reports about the repeatability of the ASRC slope measurement.
The amplitude of accommodation (AA) is another important parameter describing the accommodative system. The AA is influenced by age,Citation1912 measurement method,Citation1993 testing distance,Citation2014 target size,Citation1994 blur sensitivity,Citation1999 endpoint criterion and observer understanding,Citation1994 resulting in inconsistencies between different studies, particularly those using subjective methods. For example, the subjective push‐up test, commonly used clinically, typically overestimates the accommodative response due to the non‐linear relationship between distance and dioptres and the non‐constant angular size of the fixation target. The objective AA can be defined as the maximal accommodative response. Several studies have shown that objective measurements of the AA using dynamic retinoscopy,Citation1993 open‐field auto‐refractors or other instrumentsCitation2007 offer reliable accommodative amplitude values independent of the subjectively reported endpoint.
Hung and CiuffredaCitation1988 measured accommodative responses continuously, while subjects focused on a moving ramp stimulus at different velocities with a 2.00 D stimulus amplitude and found that the accommodative response was smooth and followed the target reasonably well for slow ramp stimuli (0.50 D/s). During their experiment, retinal image size was kept constant at different dioptric levels using a Badal stimulator. Mordi and CiuffredaCitation1998 empirically determined 0.20 to 0.35 D/s as the optimal ramp velocity and measured the ASRC with a limited stimulus amplitude (4.75 D) to calculate the ASRC slope in 30 adults; however, there was no evidence demonstrating the repeatability of such continuous measurement.
The ideal ASRC measurement for clinical and research applications should be quick and comprehensive to acquire the accommodative response over a large dioptric range in the minimum amount of time. Therefore, the aim of our study was to evaluate the repeatability of a continuous measurement of the ASRC using a motorised Badal system covering a wide range of dioptric stimuli and to analyse its agreement with other methods.
METHODS
Subjects
Twenty‐nine young adults (six men and 23 women) from 19 to 28 years (23.5 ± 2.0-years, mean and SD) were enrolled in the study. Subjects were free of ocular diseases and had no anisometropia (defined as 1.00 D or more difference in spherical equivalent refractive power between the two eyes), no more than 0.50-DC astigmatism in either eye, with a visual acuity of 6/6 or better and with normal subjective push‐up AA, that is, more than the minimum amplitude defined by the Duane‐Hofstetter formula (15–0.25 × age). Subjects were myopic, except for three emmetropes. The mean spherical equivalent refraction was −3.17 ± 1.64 D and −3.10 ± 1.61 D for the right and left eyes, respectively. Spherical equivalent refractive errors were corrected with soft contact lenses (ACUVUE ADVANCE, Johnson & Johnson, USA). This study was approved by the Ethics Committee of the Eye Hospital affiliated with Wenzhou Medical University and was conducted in accordance with the Declaration of Helsinki. Before the experimental procedures, all of the participants gave written informed consent and the possible consequences of the study were explained to them.
Badal stimulator
A Badal stimulator was mounted on an open‐field auto‐refractor (WAM‐5500; Grand Seiko Co., Ltd, Japan) coaxial with the measurement optics of the auto‐refractor and the fixation target. The fixation target was placed 4.5 metres in front of the subject's eye being measured, with the contralateral eye occluded. The Badal system consisted of a Badal lens (+5.71 D) and a mobile auxiliary lens (+3.33 D) that could be moved by means of a stepper motor controlled by a specific computer program (Figure 1).
Procedures
The accommodative stimulus‐response curves were measured with the open‐field auto‐refractor under monocular viewing conditions using the following different methods sequentially:
static ASRC using a Badal stimulator (right eye);
dynamic ASRC using a Badal stimulator (right eye); and
static ASRC stimulated by minus lenses (left eye).
The high‐contrast (90.5 per cent) fixation target was a three by three array of 6/12 black tumbling E letters printed on a white background with a mean luminance of 25-cd · m−2 at 4.5 metres and 8.5-cd · m−2 at 33-cm. These luminance levels were chosen to maintain pupil size above the minimum recommended size of 2.3-mm or moreCitation1989 required by the Grand Seiko auto‐refractor to obtain reliable accommodative responses. The subjects were instructed to keep the target as clear as possible during the measurements and rest for at least five minutes between measurements.
STATIC ASRC USING A BADAL STIMULATOR
The accommodative response was measured with the auto‐refractor on the subject's right eye, while the accommodative demand was increased from −1.00 D in 1.00 D steps using the Badal stimulator until the subject could not exert any further response. For each demand, at least five valid measurements were obtained and the mean was calculated. Between each measurement, the subjects rested for a minimum of five seconds to fixate on distant targets.
DYNAMIC ASRC USING A BADAL STIMULATOR
Subjects viewed the 4.5 metre target with their right eyes through the modified Badal system, while their left eyes were occluded. The accommodative stimulus increased continuously from −1.34 D to +11.52 D at a constant speed, which was achieved by moving the auxiliary lens. The Grand Seiko was set to high‐speed mode (continuous recording status) to collect refractive data at a temporal resolution of approximately five hertz. A laptop simultaneously recorded the synchronised accommodative stimulus (position of the auxiliary lens) and the accommodative responses through a custom‐made program. Three different speeds (0.25, 0.40 and 0.55 D/s) were used and tested in a random order. The measurements were repeated three times for each speed. A minimum of five minutes rest was provided between repetitions to fixate distant targets.
MINUS LENS STIMULATED STATIC ASRC
This method was similar to that adopted by Anderson and colleagues.Citation2008 Unlike the static and dynamic Badal methods, all measurements were obtained on the subject's left eye with the right eye occluded for practical reasons. Subjects were first instructed to fixate the three by three array of 6/12 letters at 4.5 metres and a minimum of five distance measurements were obtained. The subject was then instructed to look at the 33‐cm target and keep it as clear as possible, while a minimum of five repeated measurements were taken. The accommodative demand was increased in 1.00 D steps by adding minus lenses at the spectacle plane (13-mm) of the left eye. For each minus lens, at least five repeated measurements were obtained and the mean was calculated. Between each measurement, a minimum of five seconds of rest was provided to fixate distant targets. The power of the minus lens was increased until no additional increase in the total accommodative response was measured.
The accommodative stimulus and the accommodative response at the corneal plane were calculated using the following two equations,Citation2000 correcting for the effect of spectacle lenses on auto‐refractor readings.
In the above equations, AS and AR represent the accommodative stimulus and response, respectively; 0.013 represents the vertex distance of the trial lens in metres; DTE represents the distance in metres from the target to the eye; the lens power is the overall power of the lenses placed in front of the eye; RE cornea represents the refractive error at the corneal plane and RawAR is the spherical equivalent auto‐refractor output at the corneal plane.
Data analysis
The ASRC obtained using each method was fitted with a third‐degree polynomial equation.Citation2013 Figure 2 shows an example for a single subject.
For the static Badal stimulator and the minus lens technique, the maximum accommodative response was defined as the highest response measured and the minimum accommodative response was defined as the lowest response measured (Badal) and as the distance auto‐refraction (minimum lens). For dynamic ASRCs, the maximum and minimum accommodative responses were defined as the average of the five lowest and five highest responses measured, respectively. Objective AA was defined as the difference between the maximum and minimum responses. The slope of the ASRC curve was defined as the steepest slope of the third‐degree polynomial fit.
Statistical analyses were performed using IBM SPSS Statistics, version 19 (SPSS, Inc, Chicago, Illinois, USA). The chosen level of significance was five per cent. To assess the repeatability of three measurements for each dynamic measurement speed, the within‐subject standard deviation (Sw) was first calculated. Then, the precision, within‐subject coefficient of variation (CV) and 2.77 Sw were determined. Precision was defined as 1.96 Sw, which defines the likely limits of the difference between the measurement and the true value for 95 per cent of observations. The CV is the ratio of the Sw to the overall mean, representing the variability of a sample of measurements and 2.77 SwCitation1996 was calculated as 1.96√2 × Sw, which represents an interval within which 95 per cent of the measurements should fall. The normality of the data was confirmed by Kolmogorov–Smirnov test. Repeated measures analysis of variance (ANOVA) was used to compare the differences in the objective AA and the ASRC slope between the values collected with the static Badal stimulator method, the minus lens technique and the dynamic Badal method at three stimulus speeds. Fisher's least significant difference (LSD) post hoc test was applied for pairwise comparisons.
RESULTS
Repeatability of dynamic ASRCs under three different speeds
The repeatability of objective AA values worsened as the speed of the stimuli increased, although the differences in repeatability coefficients between slow and fast speeds were small (Table ). The repeatability of the slope was best at 0.40 D/s and worst at 0.55 D/s.
Table 1. Intra‐speed repeatability and mean value of the objective amplitude of accommodation and slope of three measurements at three speeds using a dynamic technique
Table shows the mean value of objective AA for three repetitions at the three different speeds. No significant differences were found in the objective AA or slope among three repetitions at any speed (p > 0.05).
Agreement of objective AA
The measurement method significantly influenced the objective AA value (F = 29.76, p < 0.001, Figure 3). Multiple comparisons using Fisher's least significant difference post hoc tests showed that all of the values differed from each other (p < 0.05) except for the dynamic ASRC measurement at 0.25 D/s (0.25 D/s‐DB) (5.42 ± 1.00 D) and the static ASRC measurement using the Badal stimulator (5.60 ± 0.83 D) (p > 0.05). The minus lens method induced the highest AA (6.21 ± 0.84 D). For the dynamic ASRC measurement, the objective AA decreased with increasing speed (5.42 ± 1.00 D, 5.13 ± 1.03 D, 4.73 ± 1.49 D for 0.25 D/s, 0.40 D/s and 0.55 D/s, respectively). The static Badal technique underestimated the objective AA by approximately 10 per cent compared to the minus lens technique.
Agreement of ASRC slopes
The ASRC slope varied significantly with the measurement method (F = 30.22, p < 0.001, Figure 4). Multiple comparisons using Fisher's LSD post hoc tests showed that all of the slopes differed from each other (p < 0.05) except for 0.25 D/s‐DB (0.82 ± 0.10) and 0.40 D/s‐DB (0.80 ± 0.13) (p > 0.05). The minus lens method produced the steepest slope (1.11 ± 0.14), followed by the Badal stimulator method (0.89 ± 0.09). The dynamic Badal method at 0.55 D/s‐DB produced the flattest ASRC slope (0.70 ± 0.30).
DISCUSSION
This study assessed the repeatability of dynamic ASRCs at three different dioptric speeds using a modified instrument, as well as the agreement of ASRCs obtained using three different methods. Two main ASRC parameters were analysed: the slope of the ASRC and the objective AA.
Comparison of the slope and objective AA of dynamic ASRCs at three speeds
The comparison of the objective AA and the slope of the dynamic ASRCs among three repetitions and three speeds showed that the mean objective AA and slope were affected by the stimulus‐changing speed but not by repetition, indicating that the accommodation system behaved differently based on the stimulus‐changing velocity but responded in a stable manner to each velocity. The objective AA and ASRC slope at 0.55 D/s were lowest compared to the other two speeds, showing that at 0.55 D/s, subjects could not maintain the accommodative response as precisely as at lower speeds. The outcomes of the AA measurements in our study were similar to other studies,Citation1971 which revealed that a higher temporal frequency of sinusoidal stimuli leads to a decrease in response amplitude. One interpretation of this result is that if the accommodative response cannot keep up with the continuously changing stimulus, for example, because of momentary distraction, more accommodative error will be generated at higher speeds, resulting in more retinal blur of the target, which in turn triggers a lower and less accurate accommodative response.Citation1956 Regarding the ASRC slope, the discrepancy between different stimuli‐changing speeds could be attributed to the difference in accommodative sensory gain,Citation1997 which determines the slope of the accommodative response. Higher speeds of dioptric stimuli may lead to less accommodative sensory gain; however, similar accommodative responses in terms of slope and objective AA were obtained at speeds of 0.25 D/s and 0.40 D/s, even though the objective AA at the slower speed was 0.29 D higher than that at the intermediate speed. Therefore, continuously ascending dioptric stimuli of 0.55 D/s or higher could be too fast for the accommodative system to respond accurately.
Comparison of objective AA and slope repeatability between dynamic ASRCs
The repeatability of the objective AA and ASRC slope varied with the dioptric speed of the changing stimuli; the highest speed showed the worst repeatability. The AA repeatability was worst at 0.55 D/s and best at 0.25 D/s, whereas the worst repeatability for the slope was obtained at 0.55 D/s and the best at 0.40 D/s.
Several optometric methods are available to measure AA and several studies have assessed their repeatability. Antona and colleaguesCitation2008 studied the repeatability of three subjective AA methods and demonstrated that the push‐up technique exhibited poorer repeatability and a higher coefficient of repeatability (COR, ±4.76 D) than the minus lens method (±2.52 D), which was similar to a study by Rouse, Borsting and Deland;Citation2002 however, Rosenfield and CohenCitation1996 found the same COR (±1.50 D) for both the push‐up and minus lens methods. Leon, Medrano and RosenfieldCitation2012 reported that dynamic retinoscopy exhibited higher repeatability with a lower COR (±0.61 D) than the minus lens method (±2.01 D). In our study, the precision (1.96 Sw) of the intermediate (0.68 D) and slow (0.67 D) speed approached the COR of dynamic retinoscopy in the study of Leon, Medrano and Rosenfield,Citation2012 implying that the repeatability of dynamic ASRC measurements at those two speeds was comparable to dynamic retinoscopy for the measurement of objective AA.
Dynamic versus static ASRCs under the Badal system
Measurements of static accommodation generated higher AA and steeper slopes than dynamic measurements even in the same Badal system, except for the similar objective AA obtained at 0.25 D/s with the static method. In other words, accommodation could be exerted more precisely with discontinuous stimuli. First, for static stimuli, the accommodative response has sufficient time to fully mature and reach a steady‐state level; however, with the constant vergence changes during dynamic measurements, accommodation was unable to reach a steady state, resulting in decreased slope and AA values. Second, accommodative adaptation,Citation1999 which decreases the accommodative lag over a sustained period of near fixation, may exist at a single stimuli during static measurement. In addition, the slope of Badal stimulator in our study (0.89 ± 0.09) was slightly higher than Lin and Jiang's resultsCitation2013 obtained with a Badal stimulator (0.73 ± 0.06). This discrepancy may be due to the difference in the definition of the slope and the different fixation target used; we used the steepest slope of the accommodative response curve and E letters, while Lin and Jiang used the slope of the regression line over a stimulus range from zero to 5.00 D and a Maltese cross as the fixation target.
Minus lens technique
The minus lens technique produced the highest objective AA and steepest slope compared with the other two methods. Compared to the minus lens technique, static measurement with the Badal system underestimated the AA and slope by 10 per cent and 20 per cent, respectively. Several factors may be responsible for this discrepancy. First, although they were looking through a Badal optical system, the subjects knew that the target was 4.5 metres away. This might have caused a blur‐proximal conflict, resulting in a lower slope compared with the minus lens technique. Second, target minification by the minus lens might decrease the lag of accommodation, because smaller targets would be unresolved without accurate accommodation.Citation2005 Third, the minus lens technique was performed at 33-cm, where the proximal cues of the fixation target play a non‐negligible role in accommodation;Citation1990 however, another conflicting cue for accommodation accompanied by minification is changing the apparent distance of the target,Citation1987 which would relax the response. During the minus lens measurement, no subject reported any impression of the target moving away, implying that the effect of the apparent distance was not obvious.Citation1912
In our subjects with a mean age of 23.5-years, the objective AA obtained using the minus lens technique was 0.41 D lower than the predicted amplitude measured by Anderson and colleaguesCitation2008 with a similar procedure. This small difference might be partly due to the use of different fixation targets and a shorter fixation distance for far vision refraction (4.5-metres in our study versus 6.0 metres, that is, a difference of ~0.05 D). Furthermore, the slope induced by the minus lens method at a close distance (1.11 ± 0.14) was much higher than that of the negative lens series conducted at distance by Abbott, Schmid and StrangCitation1998 (0.79 ± 0.14) and Yeo, Yang and TangCitation2006 (highest in emmetropes, 0.40 ± 0.31). This discrepancy could have been caused by different methods used to calculate the slope, that is, the steepest slope of the third‐degree polynomial fit in our study and the slope of the regression line in the two other studies. Moreover, additional proximal cues could induce a greater accommodative response.
Finally, the minus lens technique with a near target provided the highest estimate of the accommodative response among those three ASRC methods. Momeni‐Moghaddam and colleaguesCitation2014 showed that the minus lens technique with a near target generates a higher subjective AA than with a distance target, consistent with our results.
Limitations
There were several limitations to our study. First, for the same subject, we performed different methods on different eyes because of the physical constraints of our Badal system mounted on the Grand Seiko, which might have caused bias in our study; however, Vincent and colleaguesCitation2015 reported that young subjects with minimal anisometropia and astigmatism, which describes the sample in this study, exhibited very small differences in the accommodation response between fellow eyes for a range of viewing conditions assessed with an open‐field autorefractor. Second, the non‐randomisation of the order of the three methods may have led to a fatigue effect in the results and consequently the lower AA measured with the minus lens technique compared to Anderson and colleagues.Citation2008 Third, the size of our fixation target might be considered large (6/12) compared to other accommodation studies, which often use 6/9;Citation1998 however, Tan and O'LearyCitation1985 have shown that the monocular accommodative response to Snellen letters at near and far was highest for our target size. Fourth, for the minus lens technique, to counterbalance accommodative pupil miosis, we had to decrease the lighting of the near target compared to the distant target to ensure that the pupil size was larger than the minimum recommended size (2.3-mm) for measurements with the Grand Seiko WAM‐5500, as performed in previous studies;Citation1998 however, this difference should not have affected the accommodative responses of the subjects. Furthermore, to avoid accommodative fatigue, the repeatability of the static ASRC measurements was not tested, precluding a comparison of the repeatability of the dynamic and static ASRC measurements. Finally, monocular measurements ignore the possible impact of convergence factors accompanied with binocular fixation on accommodation and does not completely reflect natural viewing conditions.
CONCLUSION
The significant differences observed between the three methods used to measure ASRC parameters, including the slope and AA, indicate that these three methods are non‐interchangeable. During dynamic measurements, accommodation varies with the dioptric‐changing speed of the stimulus. For the dynamic ASRC, a speed of 0.40 D/s shortens the measurement time by 20 seconds compared to 0.25 D/s, results in a similar slope but a slightly lower objective AA (0.29 D) and has better repeatability of slope but similar repeatability of objective AA. Therefore, a speed of 0.40 D/s appears to be the best compromise in terms of time, results and repeatability for dynamic measurement of the ASRC.
ACKNOWLEDGEMENTS
This study was supported by the International S&T Cooperation Program of China (Grant No. 2014DFA30940) and was partially funded by Essilor International S.A and by the National Health and Family Planning Commission of the People's Republic of China (Grant No. 201302015).
Additional information
Funding
REFERENCES
- Johnson CA. Effects of luminance and stimulus distance on accommodation and visual resolution. J Opt Soc Am 1976; 66: 138–142.
- Mcbrien NA, Millodot M. The effect of refractive error on the accommodative response gradient. Ophthalmic Physiol Opt 1986; 6: 145–149.
- Gwiazda J, Thorn F, Bauer J et al. Myopic children show insufficient accommodative response to blur. Invest Ophthalmol Vis Sci 1993; 34: 690–694.
- Abbott ML, Schmid KL, Strang NC. Differences in the accommodation stimulus response curves of adult myopes and emmetropes. Ophthalmic Physiol Opt 1998; 18: 13–20.
- Yeo AC, Kang KK, Tang W. Accommodative stimulus response curve of emmetropes and myopes. Ann Acad Med Singapore 2006; 35: 868–874.
- Lin H, Jiang BC. Accommodative responses under different stimulus conditions. Optom Vis Sci 2013; 90: 1406–1412.
- Chauhan K, Charman WN. Single figure indices for the steady‐state accommodative response. Ophthalmic Physiol Opt 1995; 15: 217–221.
- Ciuffreda KJ, Hokoda SC, Hung GK et al. Accommodative stimulus/response function in human amblyopia. Doc Ophthalmol 1984; 56: 303–326.
- Mcbrien NA, Millodot M. The relationship between tonic accommodation and refractive error. Invest Ophthalmol Vis Sci 1987; 28: 997–1004.
- Seidel D, Gray LS, Heron G. Retinotopic accommodation responses in myopia. Invest Ophthalmol Vis Sci 2003; 44: 1035–1041.
- Anderson HA, Glasser A, Stuebing KK et al. Minus lens stimulated accommodative lag as a function of age. Optom Vis Sci 2009; 86: 685–694.
- Ciuffreda KJ, Rumpf D. Contrast and accommodation in amblyopia. Vision Res 1985; 25: 1445–1457.
- Ciuffreda KJ, Hokoda SC. Spatial frequency dependence of accommodative responses in amblyopic eyes. Vision Res 1983; 23: 1585–1594.
- Duane A. Normal values of the accommodation at all ages. JAMA 1912; 59: 1010–1013.
- Rutstein RP, Fuhr PD, Swiatocha J. Comparing the amplitude of accommodation determined objectively and subjectively. Optom Vis Sci 1993; 70: 496–500.
- Momeni‐moghaddam H, Wolffsohn JS, Azimi A et al. Effect of target distance on accommodative amplitude measured using the minus lens technique. Clin Exp Optom 2014; 97: 62–65.
- Atchison DA, Capper EJ, Mccabe KL. Critical subjective measurement of amplitude of accommodation. Optom Vis Sci 1994; 71: 699–706.
- Rosenfield M, Cohen AS. Push‐up amplitude of accommodation and target size. Ophthalmic Physiol Opt 1995; 15: 231–232.
- Rosenfield M, Abraham‐cohen JA. Blur sensitivity in myopes. Optom Vis Sci 1999; 76: 303–307.
- Win‐hall DM, Ostrin LA, Kasthurirangan S et al. Objective accommodation measurement with the Grand Seiko and Hartinger coincidence refractometer. Optom Vis Sci 2007; 84: 879–887.
- Anderson HA, Hentz G, Glasser A et al. Minus‐lens‐stimulated accommodative amplitude decreases sigmoidally with age: a study of objectively measured accommodative amplitudes from age 3. Invest Ophthalmol Vis Sci 2008; 49: 2919–2926.
- Hung GK, Ciuffreda KJ. Dual‐mode behaviour in the human accommodation system. Ophthalmic Physiol Opt 1988; 8: 327–332.
- Mordi JA, Ciuffreda KJ. Static aspects of accommodation: age and presbyopia. Vision Res 1998; 38: 1643–1653.
- Winn B, Pugh JR, Gilmartin B et al. The effect of pupil size on static and dynamic measurements of accommodation using an infra‐red optometer. Ophthalmic Physiol Opt 1989; 9: 277–283.
- Mutti DO, Jones LA, Moeschberger ML et al. AC/A ratio, age and refractive error in children. Invest Ophthalmol Vis Sci 2000; 41: 2469–2478.
- Bland JM, Altman DG. Measurement error. BMJ 1996; 313: 744.
- Kasai T, Unno M, Fujii K et al. Dynamic characteristics of human eye accommodation system. Osaka University Technical Report 1971; 21: 569–586.
- Campbell FW, Westheimer G. Dynamics of accommodation responses of the human eye. J Physiol 1960; 151: 285–295.
- Heath GG. The influence of visual acuity on accommodative responses of the eye. Am J Optom Arch Am Acad Optom 1956; 33: 513–524.
- Jiang BC. Integration of a sensory component into the accommodation model reveals differences between emmetropia and late‐onset myopia. Invest Ophthalmol Vis Sci 1997; 38: 1511–1516.
- Antona B, Barra F, Barrio A et al. Repeatability intraexaminer and agreement in amplitude of accommodation measurements. Graefes Arch Clin Exp Ophthalmol 2008; 247: 121–127.
- Rouse MW, Borsting E, Deland PN. Reliability of binocular vision measurements used in the classification of convergence insufficiency. Optom Vis Sci 2002; 79: 254–264.
- Rosenfield M, Cohen AS. Repeatability of clinical measurements of the amplitude of accommodation. Ophthalmic Physiol Opt 1996; 16: 247–249.
- Leon AA, Medrano SM, Rosenfield M. A comparison of the reliability of dynamic retinoscopy and subjective measurements of amplitude of accommodation. Ophthalmic Physiol Opt 2012; 32: 133–141.
- Rosenfield M, Gilmartin B. Accommodative error, adaptation and myopia. Ophthalmic Physiol Opt 1999; 19: 159–164.
- Schmid KL, Hilmer KS, Lawrence RA et al. The effect of common reductions in letter size and contrast on accommodation responses in young adult myopes and emmetropes. Optom Vis Sci 2005; 82: 602–611.
- Zylbermann R, Landau D, Berson D. The influence of study habits on myopia in Jewish teenagers. J Pediatr Ophthalmol Strabismus 1993; 30: 319–322.
- Rosenfield M, Gilmartin B. Effect of target proximity on the open‐loop accommodative response. Optom Vis Sci 1990; 67: 74–79.
- Rosenfield M, Ciuffreda KJ, Hung GK. The linearity of proximally induced accommodation and vergence. Invest Ophthalmol Vis Sci 1991; 32: 2985–2991.
- Rosenfield M, Ciuffreda KJ, Ong E et al. Proximally induced accommodation and accommodative adaptation. Invest Ophthalmol Vis Sci 1990; 31: 1162–1167.
- Kruger PB, Pola J. Dioptric and non‐dioptric stimuli for accommodation: target size alone and with blur and chromatic aberration. Vision Res 1987; 27: 555–567.
- Vincent SJ, Collins MJ, Read SA et al. The short‐term accommodation response to aniso‐accommodative stimuli in isometropia. Ophthalmic Physiol Opt 2015; 35: 552–561.
- Tan RK, O'leary DJ. Steady‐state accommodation response to different Snellen letter sizes. Am J Optom Physiol Opt 1985; 62: 751–754.