Abstract
The phylogenetic relationship of β-proteobacterial ammonia-oxidizing bacteria (AOB) is mainly based on comparative sequence analyses of the genes encoding the 16S rRNA (16S rDNA) and the active site polypeptide of the ammonia monooxygenase (AmoA). However, classification of AOB with different morphotypes is not possible based solely on these markers. Pyruvate kinase (PYK), which is a key enzyme in the glycolytic pathway, functions at a primary metabolic intersection. The gene sequence of PYK is known to be a phylogenetic marker for bacteria and has been used for this purpose. The phylogenetic relationships of closely related AOB, ‘Nitrosolobus’, ‘Nitrosovibrio’ and Nitrosospira, were estimated based on pyk (PYK), a housekeeping and protein-coding gene. As a result, the pyk phylogenetic tree of the AOB showed a high resolution compared with the tree based on 16S rDNA sequences. Therefore, we conclude that analysis of the PYK gene sequences could play an important role in the in-depth classification and identification of AOB at a rank below the genus level.
INTRODUCTION
Nitrification (the oxidation of ammonia to nitrate) is a key process in the global cycling of nitrogen (CitationProsser 1989). Chemoautotrophic ammonia-oxidizing bacteria (AOBs) are biocatalysts in the first step of the nitrification process, that is, the oxidation of ammonia to nitrite, which is subsequently transformed to nitrate by nitrite-oxidizing bacteria. To date, it has been verified that many AOB can be isolated from different ecosystems. The difficulty in culturing these organisms, which cannot be isolated readily from the environment, arises because of their slow growth rate, as ammonia is their sole source of energy.
β-proteobacterial AOB had been assigned to two genera, Nitrosospira (CitationHead et al. 1993, Citation1995) and Nitrosomonas. Formerly, they were separately classified by differences in their individual morphological characteristics as follows: lobate form (‘Nitrosolobus’), slender curved rods (‘Nitrosovibrio’) and spiral form (Nitrosospira). However, the identification of these three groups with different morphotypes is not possible based solely on the 16S rRNA gene (16S rDNA), which is currently the most commonly used phylogenetic marker. Therefore, we suggested the use of new markers that enable the identification of β-proteobacterial AOB in the three previously accepted genera, ‘Nitrosolobus’, ‘Nitrosovibrio’ and Nitrosospira (CitationIda et al. 2004, Citation2005). Quotation marks have been used for the generic names ‘Nitrosolobus’ and ‘Nitrosovibrio’ because these are not their official names.
A number of authors have suggested that alternative methods should be applied to clarify the phylogeny of AOB (CitationIda et al. 2004, Citation2005; CitationTeske et al. 1994). As ammonia monooxygenase subunit A and B genes are characteristic of AOB (CitationPurkhold et al. 2000; CitationRotthauwe et al. 1997), these markers have been used for the classification of AOB. However, it is not possible to completely classify the three genera using these markers. Alternative methods, using housekeeping genes, are expected to play a significant role in the phylogenetic analysis of AOB (CitationAakra et al. 2001). Unlike classifications based on protein-coding regions, although the use of phylogenetic markers using the 16S−23S rDNA intergenic spacer region (ISR) as an identification method for the species level of AOB has been reported, the identification of the closely related but phenotypically distinct taxa Nitrosospira, ‘Nitrosolobus’ and ‘Nitrosovibrio’ has not been possible using this marker (CitationAakra et al. 2001). However, such phenotypically distinct taxa should theoretically be similarly distinguished using genotypic markers (CitationPalys et al. 1997, Citation2000). Furthermore, in taxonomic studies, the concept of polyphasic taxonomy has become predominant (CitationDomig et al. 2003; CitationVandamme et al. 1996). As stated in the “Report of the ad hoc Committee for the Re-evaluation of Species Definitions in Bacteriology (2002)” (CitationStackebrandt et al. 2002), the morphotype continues to play a major role in species delineation and remains on important factor for classification. In addition, because the heterogeneity of the ISRs of the AOB results largely from insertions and deletions (CitationAakra et al. 2001), it is unlikely that ISRs can play a reliable role as molecular clocks. We previously reported the need for the use of classification markers for these three groups (CitationIda et al. 2004, Citation2005). We were able to classify them using protein-coding and housekeeping genes [glyceraldehyde-3-phosphate dehydrogenase gene (gap) and 3-phosphoglycerate kinase gene (pgk)] (CitationIda et al. 2005). In contrast to 16S rDNA, which is suitable for coarse classification, analysis of sequence data from protein-coding and housekeeping genes typically reveals clear sequence clusters that correspond to the species level (CitationCooper and Feil 2004; CitationPalys et al. 1997, Citation2000; CitationStackebrandt et al. 2002). Therefore, because of the high levels of 16S rDNA homology of these three groups, we concluded that their difference lay at a lower rank than genus (CitationIda et al. 2005).
In the present study, we attempted to demonstrate that the use of another housekeeping gene as a phylogenetic marker for classifying closely related AOB is also effective. We elucidated the phylogenetic relationships of AOB revealed by the gene sequences of pyruvate kinase (PYK: ATP-pyruvate 2-O-phosphotransferase, EC 2.7.1.40) (pyk). Pyruvate kinase catalyzes the essentially irreversible transphosphorylation from phosphoenolpyruvate (PEP) and ADP to pyruvate and ATP (CitationMuñoz and Ponce 2003). As PYK is a key enzyme in the glycolytic pathway, and the product, pyruvate, occurs in a number of metabolic pathways, this enzyme functions at a primary metabolic intersection (CitationMuñoz and Ponce 2003). Pyruvate kinase has been detected in most organisms tested for its presence and carries out the same function in all organisms tested. CitationPotter and Fothergill-Gilmore (1991) concluded that it fulfills the requirements of a phylogenetic marker. CitationPalys et al. (2000) have used pyk as a phylogenetic marker to successfully identify bacteria at the species level. The genes that encode proteins essential for central metabolism, such as pyk, and that are ubiquitous in most organisms are referred to as “core housekeeping genes” (CitationCooper and Feil 2004). As genes of this class typically evolve at a moderate rate, they can accurately distinguish taxa that, because of their extreme uniformity, cannot be classified according to their 16S rDNA sequences (CitationCooper and Feil 2004). The interesting data that we obtained appear to show that classification using this genotypic marker accurately describes evolutionary relationships along with the previously used gap and pgk genes (CitationIda et al. 2005). We carried out a phylogenetic analysis of pyk, which is important enzymatically and has been confirmed to act as a phylogenetic marker with high resolution for closely related AOB taxa. Simultaneously, we supplied evidence that supported our previous results related to the use of housekeeping genes.
MATERIALS AND METHODS
Pure cultures of AOB
lists the 16 AOB used in the present study, their abbreviations and the origins of their pure cultures (CitationIda et al. 2004, Citation2005; CitationPotter and Fothergill-Gilmore 1991; CitationSatoh et al. 2003; CitationTakahashi et al. 2001; CitationTomiyama et al. 2001). The AOB were cultured using HEPES medium under conditions previously described (Satoh et al. 2003).
Template preparation, PCR cloning and cycle sequencing
DNA isolation, polymerase chain reaction (PCR) amplification and sequencing of the PCR products were carried out as reported in a previous study (CitationIda et al. 2005).
Primer design
Three sets of primers for the pyk genes were designed using the Nitrosomonas europaea ATCC19718 genomic sequence (CitationChain et al. 2003) in the National Center for Biotechnology Information (NCBI) database. The primer sequences are listed in . The PYK genes from AOB were amplified by PCR from chromosomes using the primer pairs PGKF5–PYKR4, PYKF1–FBAR1 and PYKF2–FBAR2.
Phylogenetic analysis and DNA sequence accession numbers
The sequences obtained were analyzed and assembled in the overlapped region using the Genetyx Ver. 7.0 program package (Genetyx Corporation, Tokyo, Japan). This program was used to deduce the amino acid sequences and to carry out sequence alignment
Table 1 List of the ammonia-oxidizing bacteria used in this study
Table 2 Nucleotide sequences of polymerase chain reaction primers
RESULTS AND DISCUSSION
Gene sequences of PYK from AOB
Although in most bacteria only one form of PYK occurs, two isozymes, Type I (PykF) and Type II (PykA), have been confirmed in E. coli and Salmonella typhimurium by CitationMuñoz and Ponce (2003), who constructed a phylogenetic tree obtained from 50 aligned PYK sequences from different sources and identified six different clusters. In addition, the homology value between PykF and PykA was low: PykF and PykA belonged to cluster III and cluster VI, respectively, and displayed very different allosteric properties. Only one PykA gene was located next to the PGK gene, based on information about the genome sequence of N. europaea ATCC19718 (CitationChain et al. 2003). Indeed, all the pyk sequences from the AOB obtained in the present study belonged to the PykA gene in cluster VI (data not shown). PykA requires divalent cations (Mg2+) for activity, exhibits synergic effects in relation to PEP and is activated by adenosine monophosphate (AMP) (CitationMuñoz and Ponce 2003). The nucleotide sequences of pyk from the 16 AOB were determined in their entirety. The full length of pyk from Nitrosomonas spp., ‘Nitrosolobus’ spp., Nitrosospira spp. and ‘Nitrosovibrio’ spp. was 1491 bp (496 aa), 1455 bp (484 aa), 1455 bp (484 aa) and 1437 bp (478 aa), respectively. In general, most PYK forms consist of four identical subunits composed of approximately 500 amino acid residues (CitationMuñoz and Ponce 2003), and all the pyk sequences obtained in the present study were close to this size. The PYKs deduced from the pyk sequences of AOB displayed numerous conserved functional regions. These regions were compared to the sequence of E. coli's Type II PYK (shown in ), in which the active site of E. coli's Type II PYK showed four positively charged side-chains, Arg-36 (R), Lys-75 (K), Arg-77 and Lys-223, and three negatively charged residues, Asp-70 (D), Glu-225 (E) and Asp-252 (E. coli Type II PYK numbering etc.) (CitationMuñoz and Ponce 2003). Furthermore, Asp-70, Ser-196
Figure 1 Partial deduced amino acid sequences of pyruvate kinase (PYK) from ammonia-oxidizing bacteria (AOB). The AOB sequences were compared to the sequences of Escherichia coli type II PYK (Ec). *Homogeneity among all the species. Functionally important amino acid residues are indicated with white letters (black background).
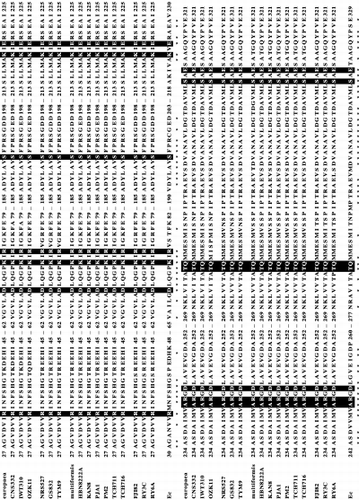
Figure 2 Phylogenetic tree inferred from comparison of pyk sequences of the β-proteobacterial ammonia-oxidizing bacteria (AOB). The tree was generated using the neighbor-joining method, and Escherichia coli type II pyk was defined as an outgroup organism. Bars represent 10% sequence divergence. Numbers indicate bootstrap values (1000 resamplings; %). Bootstrap values above 90% (•) and 70% (⧫), and accurate values are shown. Accession numbers are indicated in parentheses and based on the present officially approved nomenclature. Morphotypic and genotypic-related groups of organisms were designated as follows: Nitrosomonas cluster, Nitrosospira cluster, Nitrosolobus cluster and Nitrosovibrio cluster, and Nitrosomonas, Nitrosospira, ‘Nitrosolobus’ and ‘Nitrosovibrio’, respectively.
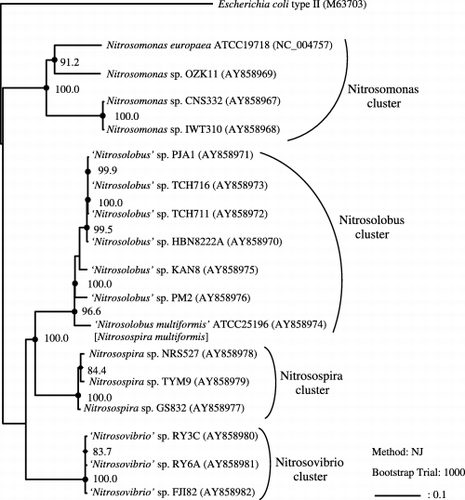
Homology and phylogenetic analysis of AOB based on pyk
It has been reported that in a comparison of 16S rDNA sequence similarities, the genera Nitrosospira, ‘Nitrosolobus’ and ‘Nitrosovibrio’ could not be distinguished from one another because of high homology values (CitationIda et al. 2004, Citation2005). The similarities of pyk were extremely high among strains within Nitrosospira (96.6–98.5%), ‘Nitrosolobus’ (93.7–99.9%) and ‘Nitrosovibrio’ (99.3–99.7%). Conversely, because of the low homology values between each of the three groups (76.8–82.3%), identification of each Nitrosospira, ‘Nitrosolobus’ and ‘Nitrosovibrio’ group was possible only according to the similarity values of pyk. It should be noted that the relationships between strains that are closely related based on pyk similarities completely corresponded to the formerly reported gap similarities (CitationIda et al. 2005). In addition, almost all these relationships corresponded to the pgk similarities. The phylogenetic tree based on the full length of pyk is illustrated in with PykA (Type II PYK) of E. coli as an outgroup. The other phylogenetic tree based on the Unweighted Pair Group Method with Arithmatic mean (UPGMA) method (CitationSokal and Michener 1958) showed the same results (data not shown). The pyk phylogenetic tree clearly enables a distinction between the “Nitrosomonas cluster”, “Nitrosospira cluster”, “Nitrosolobus cluster” and the “Nitrosovibrio cluster” previously defined in the gap and pgk phylogenetic trees (CitationIda et al. 2005). We conclude that the topology of the pyk phylogenetic tree resembles that of the pgk and gap phylogenetic trees. We also conclude that the variation that has accumulated in the pgk sequences is insufficient to erode the high similarity values between Nitrosospira and “Nitrosolobus”, as observed with the 16S rDNA sequences.
As the pyk phylogenetic tree showed a high resolution, the differences between each cluster, supported by high bootstrap values, could be resolved (). Furthermore, the topology of the pyk tree did not conflict with that of the 16S rDNA tree, in which the Nitrosomons cluster was clearly separated. Based on these results and the differences in the morphological characteristics of the genera Nitrosospira, ‘Nitrosolobus’ and ‘Nitrosovibrio’, we conclude that the evolutionary relationships of AOB could be clearly defined using pyk.
The phylogenetic relationships of Nitrosomonas spp. clustered in the pyk tree corresponded to those previously reported in the gap and pgk trees (CitationIda et al. 2005). In turn, strains CNS332 and IWT310 were closely related, while strains N. europaea and OZK11 were distant in both the gap and pgk trees. These relationships were reflected in the pyk tree. Although, within the genus Nitrosomonas, phenotypic differences closely coincided with the phylogenetic lineages in 16S rDNA (CitationKoops and Pommerening-Röser 2001), the construction of a larger database of Nitrosomonas species will be necessary to confirm whether the phylogenetic relationships of the gap, pgk and pyk trees correspond to those of the 16S rDNA tree.
In conclusion, the phylogenetic tree based on the gene sequences of PYK, a key enzyme in the glycolytic pathway, enabled successful distinction of the three genera Nitrosospira, ‘Nitrosolobus’ and ‘Nitrosovibrio’, whose identification could not be clearly resolved into different clusters using 16S rDNA sequence comparison. Moreover, because the topology of the pyk phylogenetic tree resembled that of the pgk and gap trees and the pyk tree enabled classification of the closely related AOB ‘Nitrosolobus’, ‘Nitrosovibrio’ and Nitrosospira more accurately than the pgk and gap trees, we conclude that the pyk tree reflects the evolutionary relationships of β-proteobacterial AOB more clearly than the gap or pgk tree. Based on these results, we are convinced that analysis of PYK gene sequences could play an important role in the in-depth classification and identification of β-proteobacterial AOB at a lower rank than the genus level.
Analysis of high-resolution housekeeping and protein-coding gene sequences that evolve steadily, such as pyk, is a technique with major future potential for identifying markers that should enable the precise classification of bacteria. However, to ensure reliability, accumulation of pyk sequence data of AOB will continue to be necessary.
ACKNOWLEDGMENTS
This study was supported by the Grants-in-Aid for the Open Research Center Projects, the 21st century: Centers of Excellence (COE) program and Scientific Research (C) from the Ministry of Education, Culture, Sports, Science and Technology of Japan.
REFERENCES
- Prosser , JI . 1989 . Autotrophic nitrification in bacteria . AdvMicrobPhysiol , 30 : 125 – 181 .
- Head , IM , Hiorns , WD , Embley , TM , McCarthy , AJ and Saunders , JR . 1993 . The phylogeny of autotrophic ammonia-oxidizing bacteria as determined by analysis of 16S ribosomal RNA gene sequences . JGenMicrobiol , 139 : 1147 – 1153 .
- Head , IM , Hiorns , WD , Embley , TM , McCarthy , AJ and Saunders , JR . 1995 . Nitrosospira multiformiscomb. nov. and Nitrosospira tenuiscomb. nov. In validation of the publication of new names and new combinations previously effectively published outside the IJSB, List no. 54 . IntJSystBacteriol , 45 : 619 – 620 .
- Ida , T , Satoh , M , Yabe , R , Takahashi , R and Tokuyama , T . 2004 . Identification of genus Nitrosovibrio, ammonia-oxidizing bacteria, by comparison of the N-terminal amino acid sequences of phosphoglycerate kinase . JBiosciBioeng , 98 : 380 – 383 .
- Ida , T , Kugimiya , M , Kogure , M , Takahashi , R and Tokuyama , T . 2005 . Phylogenetic relationships among ammonia-oxidizing bacteria as revealed by gene sequences of glyceraldehyde 3-phosphate dehydrogenase and phosphoglycerate kinase . JBiosciBioeng , 99 : 569 – 576 .
- Teske , A , Alm , E , Regan , JM , Toze , S , Rittman , BE and Stahl , DA . 1994 . Evolutionary relationships among ammonia- and nitrite-oxidizing bacteria . JBacteriol , 176 : 6623 – 6630 .
- Purkhold , U , Pommerening-Röser , A , Juretschko , S , Schmid , MC , Koops , HP and Wagner , M . 2000 . Phylogeny of all recognized species of ammonia oxidizers based on comparative 16S rRNA and amoAsequence analysis: implication for molecular diversity surveys . ApplEnvironMicrobiol , 66 : 5368 – 5382 .
- Rotthauwe , JH , Witzel , KP and Liesack , W . 1997 . The ammonia monooxygenase structural gene amoAas a functional marker: molecular fine-scale analysis of natural ammonia-oxidizing populations . ApplEnvironMicrobiol , 63 : 4704 – 4712 .
- Aakra , Å , Utåker , JB , Pommerening-Röser , A , Koops , HP and Nes , IF . 2001 . Detailed phylogeny of ammonia-oxidizing bacteria determined by rDNA sequences and DNA homology values . IntJSystBacteriol , 51 : 2021 – 2030 .
- Palys , T , Nakamura , LK and Cohan , FM . 1997 . Discovery and classification of ecological diversity in the bacterial world: the role of DNA sequence data . IntJSystBacteriol , 47 : 1145 – 1156 .
- Palys , T , Berger , E , Mitrica , I , Nakamura , LK and Cohan , FM . 2000 . Protein-coding genes as molecular markers for ecologically distinct populations: the case of two Bacillusspecies . IntJSystBacteriol , 50 : 1021 – 1028 .
- Domig , KJ , Mayer , HK and Kneifel , W . 2003 . Methods used for the isolation, enumeration, characterization and identification of Enterococcusspp. 2. Pheno and genotypic criteria . IntJFood Microbiol , 88 : 165 – 188 .
- Vandamme , P , Pot , B , Gillis , M , de Vos , P , Kersters , K and Swings , J . 1996 . Polyphasic taxonomy, a consensus approach to bacterial systematics . Microbiol Rev , 60 : 407 – 438 .
- Stackebrandt , E , Frederiksen , W Garrity , GM . 2002 . Report of the ad hoc committee for the re-evaluation of the species definition in bacteriology . IntJSystEvolMicrobiol , 52 : 1043 – 1047 .
- Cooper , JE and Feil , EJ . 2004 . Multilocus sequence typing-what is resolved? . Trends Microbiol , 12 : 373 – 377 .
- Muñoz , ME and Ponce , E . 2003 . Pyruvate kinase: current status of regulatory and functional properties . CompBiochemPhysiolB BiochemMolBiol , 135 : 197 – 218 .
- Potter , S and Fothergill-Gilmore , LA . 1991 . The pyruvate kinase of Thermoplasma acidophilum: purification, kinetic characterization and use as a phylogenetic marker . BiochemSocTrans , 20 : 11S
- Satoh , K , Yanagida , T Isobe , K . 2003 . Effect of root exudates on growth of newly isolated nitrifying bacteria from barley rhizoplane . Soil SciPlant Nutr , 49 : 757 – 762 .
- Takahashi , R , Ohishi , M , Ohshima , M , Saitoh , M , Omata , K and Tokuyama , T . 2001 . Characteristics of an ammonia-oxidizing bacterium with a plasmid isolated from alkaline soils and its phylogenetic relationship . JBiosciBioeng , 92 : 232 – 236 .
- Tomiyama , H , Ohshima , M Ishii , S . 2001 . Characteristics of newly isolated nitrifying bacteria from rhizoplane of paddy rice . Microbes Environ , 16 : 101 – 108 .
- Chain , P , Lamerdin , J Larimer , F . 2003 . Complete genome sequence of the ammonia-oxidizing bacterium and obligate chemolithoautotroph Nitrosomonas europaea . JBacteriol , 185 : 2759 – 2773 .
- Barton , JG and Sternbert , MJ . 1987 . A strategy for the rapid multiple alignment of protein sequence: confidence levels from tertiary structure comparisons . JMolBiol , 198 : 327 – 337 .
- Tyler , EC , Horton , MR and Krause , PR . 1991 . A review of algorithms for molecular sequence comparison . CompBiomedRes , 24 : 72 – 96 .
- Saitou , N and Nei , M . 1987 . A neighbor-joining method: a new method for reconstructing phylogenetic trees . MolBiolEvol , 4 : 406 – 425 .
- Altschul , SF , Madden , TL Schaffer , AA . 1997 . Gapped BLAST and PSIBLAST: a new generation of protein database search programs . Nucleic Acids Res , 25 : 3389 – 3402 .
- Sokal , RR and Michener , CD . 1958 . A statistical method for evaluating systematic relationships . UniKansas SciBull , 28 : 1409 – 1438 .
- Koops , HP and Pommerening-Röser , A . 2001 . Distribution and ecophysiology of the nitrifying bacteria emphasizing cultured species . FEMS Microbiol Ecol , 37 : 1 – 9 .