Abstract
An anaerobic incubation experiment was conducted to investigate methane (CH4) production potential in soil samples collected from a paddy field after exposure to free-air CO2 enrichment (FACE). The FACE experiment with two CO2 levels, ambient and ambient + 200 p.p.m.v CO2 during the rice growing season, was conducted at Shizukuishi, Iwate Prefecture, Japan. The soil was a wet Andosol. Soil samples were taken from the surface (0–1 cm) and the sub-surface (1–10 cm) soil layers 2 months after rice harvest. Sub-samples of the fresh soils were put into glass bottles and submerged under N2 gas headspace during the incubation. The results showed that, prior to incubation, the contents of total C and dissolved organic C (DOC) were significantly greater in FACE soil than ambient soil. During the incubation, CH4 production potential was approximately 2–4-fold higher in FACE soil than ambient soil and approximately 500–1,000-fold greater in surface soil than sub-surface soil. In general, the FACE soil contained more DOC than ambient soil, particularly in the surface soil layer. These findings suggest that FACE treatment exerted long-term positive effects on CH4 production and increased organic C content in this paddy soil, particularly in the surface soil layer.
Key words:
INTRODUCTION
The atmospheric CO2 concentration has been increasing at a rate of 0.5% annually and the major increase has occurred since the 1850s (CitationLal and Kimble 1995). Fossil fuel combustion and land-use changes are two principal human activities responsible for the increase. Increased atmospheric CO2 can stimulate rice biomass production (above and belowground) and grain yield (CitationKim et al. 2003; CitationSakai et al. 2001; CitationZiska et al. 1997). Stimulated root growth can release more carbonaceous substances to soil in the form of rhizodeposition, such as root exudates, secretion, sloughed-off cells and decaying roots (CitationLi and Yagi 2004; CitationLynch and Whipps 1990).
Dissolved organic C (DOC) is a mobile and labile form of soil organic C. In general, root exudates and microbially degraded products are regarded as the most important DOC sources. Elevated CO2 can affect DOC dynamics in soils by increasing the amount of soluble organic C compounds from roots, and by stimulating or depressing decomposition of soil organic matter depending on N supply levels. However, most studies on elevated CO2 effects have been carried out in upland soils (CitationCheng and Johnson 1998; CitationGinkel et al. 2000; CitationSchortemeyer et al. 1996) and only a few studies have been conducted in paddy soils (CitationCheng et al. 2001; CitationHoque et al. 2001; CitationInubushi et al. 2003). The direct effect of elevated CO2 on DOC dynamics would be expected to affect CH4 production and emission from paddy soils indirectly, but such an effect has not been extensively identified. Furthermore, most investigations on CH4 production and emission have been conducted during the rice growing season (CitationHoque et al. 2001; CitationInubushi et al. 2003), but limited information is available on CH4 production potential in rice soils collected after rice harvest or during the off-crop period.
The objective of this research was to investigate CH4 production potential in surface and sub-surface soil samples collected 2 months after rice harvest from a paddy field exposed to free-air CO2 enrichment in Japan.
MATERIALS AND METHODS
The paddy soil used in this study was collected from a field experiment that was conducted under free-air CO2 enrichment (FACE) conditions at Shizukuishi, Iwate Prefecture, Japan (latitude 39°40′, longitude 141°E, elevation 200 m a.s.l.). The soil was a wet Andosol, a typical paddy soil in Iwate Prefecture. The FACE experiment with two CO2 levels, ambient and ambient + 200 p.p.m.v CO2, was designed using a randomized complete block with four replicates. The FACE plots were named A, B, C and D, and the ambient plots were named W, X, Y and Z. The FACE facility was fully described by CitationOkada et al. (2001).
Rice seedlings (Oryza sativa L cv. Akitakomachi) were raised in growth chambers for 3 weeks at Tohoku National Agricultural Experiment Station, Morioka, Japan. The seedlings for ambient CO2 plots were grown in a chamber supplied with ambient CO2 concentration, and those for FACE plots grown in a separate chamber with elevated CO2 (ambient + 200 p.p.m.v). The seedlings were then transplanted by hand into the field at a density of three plants per hill and 19 hills m−2 on 20 May 2004. Nitrogen fertilizers were applied as basal dressing at a rate of 80 kg N ha−1, of which 60 kg was supplied as controlled release urea and 20 kg as ammonium sulfate. Phosphorus and K fertilizers were applied as basal dressings at a rate of 130 kg P ha−1 and 120 kg K ha−1, respectively. The above fertilizer rates (N–P–K) are close to the standard fertilizer rates (90–130–125 kg ha−1 N–P–K) in the region of Japan (CitationHoque et al. 2001). Throughout the growing season (i.e. from transplanting until harvest), CO2 was continuously supplied and released above the rice plant canopy, and diluted by wind turbulence in the FACE plots. Rice was
Table 1 Changes in root dry biomass (g m−2) in plots exposed to free-air CO2 enrichment (FACE) and ambient CO2
Soil samples (surface 0–1 cm and sub-surface 1–10 cm) were separately collected on 2 December 2004 from FACE (plots B, C and D) and ambient (plots W, Y and Z) fields after rice harvest. Plots A and X had been ploughed before our sampling. After removal of all visible plant debris, roots and small stones, the soil was kept at 5°C in a refrigerator prior to incubation. Selected properties of the soil samples used in this experiment are listed in . Total C and N contents were determined using a CN analyzer (MT-700 with an Auto Sampler MTA-600; Yanaco, Kyoto, Japan). Soil pH value (1:1, H2O) was measured using a pH meter (HORIBA/F-13, HORIBA Advanced Techno, Co., Ltd., Kyoto, Japan.).
After homogenization, 10 g sub-samples of the fresh soils (equivalent to 5 g oven-dried soil, in triplicate) were placed into glass bottles (50 mL) and submerged under a 2 cm water layer during the entire incubation. The air in the bottles was removed by evacuation and replaced with N2 gas after the evacuated bottles were sealed with butyl rubber stoppers. The soils were incubated
Table 2 Selected properties of the soil samples used in this experiment
At the end of the incubation, the bottles were vigorously shaken by hand to obtain soil slurries. The slurries were transferred to centrifuge tubes, and centrifuged at 2,150 g for 15 min. The supernatants were filtered through a 0.40 µm Millipore filter and stored at −20°C. The organic C content in the solutions regarded as DOC was analyzed using a total carbon analyzer (TOC-5000, Shimadzu, Japan).
Statistical analysis was carried out using SPSS software (SPSS Inc., Chicago, Illinois, USA). All data were expressed as mean ± standard error and subjected to anova, Tukey's and t-tests (P < 0.05).
RESULTS
Root dry biomass and soil chemical properties
Root dry biomass was 10–12 g m−2 more under FACE conditions than ambient conditions at each sampling date (). Compared with the ambient treatment, FACE treatment significantly increased total C and total N contents and DOC content in both the surface soil and sub-surface soil. Higher contents of total C and total N as well as DOC were obtained in the surface soil than the sub-surface soil. In contrast, pH values were markedly decreased in the soil samples with FACE compared with ambient CO2 ().
CH4 production potential in surface and sub-surface soils
In general, CH4 production potential increased from the start to the end of the incubation (). No significant difference in CH4 production potential was found between the FACE and ambient treatments at the early stage of the incubation, that is, within 2 days for surface soil and 10 days for sub-surface soil. However, towards the end of the incubation, CH4 production potential became significantly higher in FACE soil (collected from FACE plots) than ambient soil (taken from ambient plots), regardless of soil depth. Furthermore, at the end of incubation a marked difference in CH4 production potential was observed between surface soil and sub-surface soil, and CH4 production potential was approximately 500–1,000-fold greater in surface soil than sub-surface soil.
Figure 1 Change in CH4 production from (a) surface (0–1 cm) and (b) sub-surface (1–10 cm) soil samples collected from a rice paddy field exposed to elevated and ambient CO2 concentrations. Vertical bars indicate the standard error of the mean. Values are the mean of three replicates. Asterisks indicate significant differences between free-air CO2 enrichment (FACE) and ambient treatments using an independent sample t-test (P ≤ 0.05).
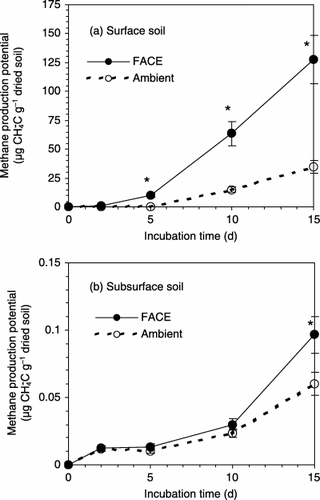
Dissolved organic C content in surface and sub-surface soils
The DOC content was measured at the end of the incubation (). A significant difference in DOC content was observed in surface soil samples between the FACE and ambient treatments, but not in sub-surface soil samples. The amount of DOC obviously increased with FACE treatment in surface soil samples compared with the ambient treatment. For the FACE treatment, higher DOC content was detected in surface soil than sub-surface soil samples.
DISCUSSION
Free-air CO2 enrichment obviously stimulated root biomass production (), which is similar to our
Figure 2 Dissolved organic C (DOC) in surface (0–1 cm) and sub-surface (1–10 cm) soil samples collected from a rice paddy field exposed to elevated and ambient CO2 concentrations. Columns with the same letter were not significantly different using Tukey's tests (P ≤ 0.05). Vertical bars indicate the standard error of the mean. Values are the mean of three replicates.
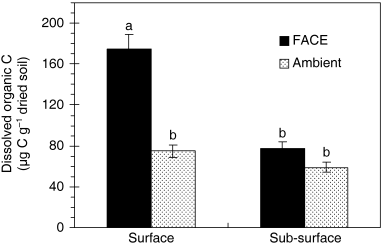
During the rice growing period, methane production was enhanced by CO2 enrichment because of the stimulated root biomass in the form of root exudates or root autolysis products, which were readily decomposed by fermentative bacteria to CO2, H2 and acetate, and then utilized by methanogens and further changed into CH4 (CitationInubushi et al. 2003; CitationLi et al. 2004; CitationMinoda et al. 1996). In the above experiments, the soil samples for measuring CH4 production were taken during the rice growing season. However, the soil samples tested in the present study were collected 2 months after rice harvest. The labile organic C, such as low molecular weight organic substances (e.g. organic acids and amino acids), contained in root exudates had possibly been degraded during the off-crop period. After rice harvest, plant-derived C remained in the soil at least in three forms: root residues, stubble and rhizodeposits (CitationKimura et al. 2004). An incubation experiment with 13C-tracer showed that, after 60 days of incubation, rhizodeposits C in water-soluble organic C decreased more rapidly than those in microbial biomass C and soil organic C, and further indicated that plant-derived C (root and stubble) accounted for 18–28% of total water-soluble organic C, 17–32% of total microbial biomass C and 2.3–4.1% of total soil organic C, respectively (CitationLu et al. 2003). In addition, during the rice growing season, microbial biomass C was enhanced in paddy soil, particularly in the surface soil layer of FACE treatment (CitationInubushi et al. 2001). Thus, the higher CH4 production potential in the FACE surface soil () might be caused by the decomposition of microbial biomass C and water-soluble organic C derived from photosynthesized C allocated to soil during the rice growing season (CitationHoque et al. 2001; CitationInubushi et al. 2001). Another possibility is that high molecular weight organic substances derived from the rice plant were decomposed to low molecular weight substances contributing to CH4 production. Our results suggest that FACE treatment greatly affected C storage and turnover in the paddy soil, and exerted long-term effects on CH4 production, particularly in the surface soil layer.
In conclusion, as the tested soil samples were collected 2 months after rice harvest, this study provided an insight into the long-term effects of FACE on CH4 production potential, as well as C sequestration in the paddy soil. The FACE treatment exerted long-term positive effects on CH4 production and increased the organic C content in the paddy soil, particularly in the surface soil layer. Further research is needed to investigate the contribution of surface soil to CH4 production in the flooded paddy field under CO2 enrichment.
ACKNOWLEDGMENTS
This study was financially supported by the Japan Society for the Promotion of Science in the form of a postdoctoral fellowship to Dr Y. Lou (P04429), the International Foundation for Science (C/3593) and the Rice-FACE project under the CREST (Core Research for Evolutional Science and Technology) program of Japan Science and Technology Corporation. We would like to express our special thanks to Dr Padre Agnes from the International Rice Research Institute, Philippines, for checking this manuscript.
REFERENCES
- Lal , R , Kimble , J , Levine , E and Stewart , BA . 1995 . Advances in Soil Science: Soils and Global Change , 1 – 2 . Boca Raton, Florida, USA : CRC Press .
- Kim , HY , Lieffering , M , Kobayashi , K , Okada , M and Miura , S . 2003 . Seasonal changes in the effects of elevated CO2on rice at three levels of nitrogen supply: a free air CO2enrichment (FACE) experiment . Global Change Biol , 9 : 826 – 837 .
- Sakai , H , Yagi , K , Kobayashi , K and Kawashima , S . 2001 . Rice carbon balance under elevated CO2 . New Phytol , 150 : 241 – 249 .
- Ziska , LH , Namuco , OS , Moya , TB and Quilang , J . 1997 . Growth and yield response of field-grown tropical rice to increasing carbon dioxide and air temperature . AgronJ , 89 : 45 – 53 .
- Li , Z and Yagi , K . 2004 . Rice root-derived carbon input and its effect on decomposition of old soil carbon pool under elevated CO2 . Soil BiolBiochem , 36 : 1967 – 1973 .
- Lynch , JM and Whipps , JM . 1990 . Substrate flow in the rhizosphere . Plant Soil , 129 : 1 – 10 .
- Cheng , W and Johnson , DW . 1998 . Elevated CO2 rhizosphere processes, and soil organic matter decomposition . Plant Soil , 202 : 167 – 174 .
- Ginkel , JH , Gorissen , A and Polci , D . 2000 . Elevated atmospheric CO2concentration: effects of increased C input in a Lolium perennesoil on microorganisms and decomposition . Soil BiolBiochem , 32 : 449 – 456 .
- Schortemeyer , M , Hartwig , UA and Hendrey , GR . 1996 . Microbial community changes in the rhizosphere of white clover and perennial ryegrass exposed to free air CO2enrichment (FACE) . Soil BiolBiochem , 28 : 1717 – 1724 .
- Cheng , W , Inubushi , K , Yagi , K , Sakai , H and Kobayashi , K . 2001 . Effect of elevated CO2on biological nitrogen fixation, nitrogen mineralization and carbon decomposition in submerged rice soil . BiolFertSoils , 34 : 7 – 13 .
- Hoque , MM , Inubushi , K Miura , S . 2001 . Biological dinitrogen fixation and soil microbial biomass carbon as influenced by free-air carbon dioxide enrichment (FACE) at three levels of nitrogen fertilization in a paddy field . BiolFertSoils , 34 : 453 – 459 .
- Inubushi , K , Cheng , W Aonuma , S . 2003 . Effects of free-air CO2enrichment (FACE) on CH4emission from a rice paddy field . Global Change Biol , 9 : 1458 – 1464 .
- Okada , M , Lieffering , M , Nakamura , H , Yoshimoto , M , Kim , HY and Kobayashi , K . 2001 . Free-air CO2enrichment (FACE) using pure CO2injection: system description . New Phytol , 150 : 251 – 260 .
- Inubushi , K , Cheng , W and Chander , K . 1999 . Carbon dynamics in submerged soil microcosms as influenced by elevated CO2and temperature . Soil SciPlant Nutr , 45 : 863 – 872 .
- Inubushi , K , Hoque , MM Miura , S . 2001 . Effect of free-air CO2enrichment (FACE) on microbial biomass in paddy field soil . Soil SciPlant Nutr , 47 : 737 – 745 .
- Inubushi , K , Wada , H and Takai , Y . 1982 . Easily decomposable organic matter in paddy soils (II) Chlorophyll-type compounds in Apg horizons . JpnJSoil SciPlant Nutr , 53 : 277 – 282 . (in Japanese)
- Li , Z , Yagi , K , Sakai , H and Kobayashi , K . 2004 . Influence of elevated CO2and nitrogen nutrition on rice plant growth, soil microbial biomass, dissolved organic carbon and dissolved CH4 . Plant Soil , 258 : 81 – 90 .
- Minoda , T , Kimura , M and Wada , E . 1996 . Photosynthates as dominant source of CH4and CO2in soil water and CH4emitted to the atmosphere from paddy fields . JGeophysRes , 101 : 21091 – 21097 .
- Kimura , M , Murase , J and Lu , Y . 2004 . Carbon cycling in rice field ecosystems in the context of input, decomposition and translocation of organic materials and the fates of their end products (CO2and CH4) . Soil BiolBiochem , 36 : 1399 – 1416 .
- Lu , Y , Watanabe , A and Kimura , M . 2003 . Carbon dynamics of rhizodeposits, root- and shoot-residues in a rice soil . Soil BiolBiochem , 35 : 1223 – 1230 .