Abstract
The role of arbuscular mycorrhizal fungus (AMF) on biotransformation of arsenic (As) in the rhizosphere of sunflower (Helianthus annuus L.) was studied in a rhizobag system with As-contaminated soil. The treatments consisted of a combination of two levels of AMF (Glomus aggregatum) inoculation (–AM and +AM) under sterile and non-sterile soil conditions (S and NS). Each treatment was designated as –AM/S, –AM/NS, +AM/S and +AM/NS. Sunflower seedlings were cultured in the rhizobag for 6 weeks. Rates of root AMF colonization in +AM treatments were approximately 40% and 0% in –AM/S treatment. The AMF inoculation reduced As toxicity symptoms and improved plant growth. Shoot As, but not the root As, concentrations were reduced by AMF inoculation. Shoot and root P concentrations increased in +AM treatments regardless of soil sterilization. Analyses of water soluble (WS) As in the soils at the end of the cultivation indicated that the amount of WS-arsenite (AsIII) was higher in the central compartment compared with the outer compartment, while WS-arsenate (AsV) was dominant in the outer compartment. Dimethylarsinic acid (DMAA) was detected only in +AM treatments and the concentration was higher in the +AM/NS treatment compared to the +AM/S. Trimethylarsine oxide (TMAO) was also detected in measurable amounts in the NS condition and AMF inoculation increased the amount of TMAO, especially in the central compartment. No methylated As species was detected in the –AM/S treatment. From these results, it is clear that the mycorrhizal roots colonized by Glomus aggregatum are primarily involved in DMAA formation. Aside from these mycorrhizal roots, the indigenous soil microorganisms in the mycorrhizosphere are responsible for promoting the transformation of DMAA into TMAO. These As biomethylation processes would favor the detoxification of As, especially at the interface of plant roots and rhizosphere soil.
INTRODUCTION
Arsenic (As) pollution is a serious environmental problem, especially in Bangladesh, Vietnam, Taiwan and the West Bengal District of India (CitationAbedin et al. 2002; CitationMeharg 2004). To understand the movement of As in the soil–water environment, several researchers have examined the vertical distribution of As in sediment columns taken by boring operations (CitationYamazaki et al. 2003a), As extractability (CitationYamazaki et al. 2003b), and background As contents in soils (CitationAli et al. 2003). In contrast, findings of As-accumulating ferns have created an opportunity for developing strategies that would remediate As-contaminated soils by using plant uptake ability (CitationMa et al. 2001; CitationVan et al. 2006). For the development of effective phytoremediation technology and for alleviating As toxicity in crop plants, an understanding of the dynamics of As in the plant–soil system is indispensable.
Soil microorganisms affect metal speciation and availability through their ability to modify its solubility (CitationBently and Chasteen 2002; CitationTurpeinen et al. 1999). Mobilization occurs through processes such as protonation, chelation and chemical transformation, while immobilization occurs through crystallization, sorption and assimilation into biomass. Such abilities of soil microorganisms to transform metals and metalloids in soils can be utilized as a means for removal, recovery or detoxification of inorganic and organic metal pollutants. Soil microorganisms also mediate As transformation processes, including methylation, demethylation, reduction of arsenate (AsV) and oxidation of arsenite (AsIII) (CitationGadd 2004). Such biotransformation influences the fate, mobility and bioavailability of As in soils, controlling its effects on soil organisms and its uptake by higher plants (CitationFitz and Wenzel 2002).
Mycorrhiza is an integral and functional part of plant roots that plays an important role in alleviating metal toxicity in plants. One of the dominant forms of mycorrhizas for symbiosis with plants is arbuscular mycorrhizal fungi (AMF), which belongs to the groups of endomycorrhiza (CitationSmith and Read 1997). The role of AMF in plant uptake of heavy metals has been extensively studied. Several studies have shown that AMF often protect plants against high concentrations of non-essential metals in their shoots by enhancing metal retention in the roots (CitationChristie et al. 2004; CitationJoner et al. 2000; CitationLeyval et al. 1997), in addition to the improvement of phosphorus (P) nutrition status (CitationLi et al. 1991) and uptake of some essential elements, resulting in greater plant biomass (CitationMarschner 1995). Because As has great similarity in chemical behavior to P, it is thought that AMF would also influence As dynamics in soils. The results of several studies on the role of mycorrhiza on As uptake by plants have been recently reported. The general consensus is that plants colonized by mycorrhizal fungi display enhanced resistance to As contamination in soils (CitationMeharg and Hartley-Whitaker 2002). Several studies suggest that mycorrhiza reduces the As concentration in shoots (CitationAsher and Reay 1979; CitationGonzalez-Chaves et al. 2002; CitationLiu et al. 2005; CitationMeharg et al. 1994; CitationSharples et al. 2000; CitationUltra et al. 2007) and acts as an As filter to maintain low As concentrations in the shoots, while improving P nutrition of the host plant.
As a rule, inorganic As species are more toxic than organic As species (CitationAdriano 2001). The AMF are known to change the chemical composition of root exudates and to affect the activities of soil enzymes, and to introduce physical modifications to the environment surrounding the roots (CitationSmith and Read 1997). These AMF-induced changes in the rhizosphere and the development of AMF mycelia, which can act as a nutrient source for other microorganisms, are likely to influence the structure and functioning of soil microbial communities in the mycorrhizosphere. Similarly, there could be specific modification in the environment between the plant roots and the mycorrhizosphere, such as changes in pH and redox potential, that could possibly promote the biotransformation process of As (CitationJeffies et al. 2002). Possible mechanisms to reduce As toxicity may include the enhancement of indigenous microflora that are capable of metabolizing As, the AMF association triggering the evolution of As co-metabolism between plant and AMF, and the direct involvement of AMF on As biomethylation (CitationBarea et al. 2005). As there are large numbers of bacteria (including actinomycetes) and fungi that are capable of As biomethylation (CitationFrankenberger and Arshad 2002), increased growth and activity of these indigenous microorganisms can be expected to promote the production of organic As species.
Only a few studies have directly investigated the fates of As in the rhizosphere with respect to the potential interactions in the soil–plant–microbe system. Recently, we examined the effects of AMF inoculation and P application on As dynamics in the rhizosphere of sunflower and detected the presence of dimethylarsinic acid (DMAA) in the soil inoculated with AMF (CitationUltra et al. 2007). Based on this result, we hypothesized that the chemical behavior and toxicity of As were altered in the mycorrhizosphere, which could be contributing to better survival of mycorrhizal plants in As-contaminated soil. However, it has not yet been clarified whether AMF and mycorrhizal roots are primarily involved in the transformation of inorganic As into the organic forms or whether the indigenous microorganisms are responsible for the process. Therefore, in this study, we attempted to elucidate the role of AMF on As biomethylation in the rhizosphere of sunflower through the evaluation of different As species and enzymatic activities in the rhizosphere soil.
MATERIALS AND METHODS
Soil sampling and rhizobag assembly
The As-contaminated soil used in these experiments was collected from the sub-surface layer (20–30 cm) of a cypress forest near a closed silver mine in Isotake, Ohda city, Shimane prefecture, Japan. The soil properties and the heavy metal contents were reported in a previous paper (CitationUltra et al. 2007). After air-drying, soil samples for cultivation and chemical analysis were passed through 5 mm and 2 mm mesh sieves, respectively. Soil for the sterile treatments was autoclaved at 121°C, 1 h, for three consecutive days prior to use. The comparison of some selected chemical properties before and after sterilization is given in . Methylated As species were not detected for the sterile and non-sterile soils prior to the experiment.
A rhizobag system composed of two compartments was used for the experiment. The system had one central compartment in which the plants were grown, and an outer compartment serving as the mycorrhizosphere (). The central compartment was separated using a 25 µm nylon mesh mounted on a cylindrical plastic
Table 1 Chemical properties of the soil collected from Shimane Prefecture, Japan, as affected by sterilization
Description of the treatments and plant culture
The treatments consisted of two levels of AMF (Glomus aggregatum) inoculation (–AM and +AM) under sterile and non-sterile soil conditions (S and NS), comprising four-treatment combinations. Each treatment was designated as –AM/S, –AM/NS, +AM/S and +AM/NS. Air-dried soil was used for non-sterile soil and autoclaved soil was used as sterile soil. Five grams of sterile (121°C, 30 min for three consecutive days) or non-sterile AMF inoculants (Kinkon-King, Hokko Chemical, Tokyo, Japan) were mixed with the soil in the central compartment of the –AM and +AM treatments, respectively. The only beneficial microorganism in the AMF inoculant used in these experiments was AMF (Glomus aggregatum) (Iwasaki K, 1998, pers. comm.). Sunflower (Helianthus annuus L. cv. “Sweet”) seeds were surface sterilized using a 70% ethanol solution for 5 min, then washed
three times with sterile distilled water and germinated on sterile petriplates lined with moistened filter paper. The pre-germinated seed was then planted in the central compartment. Pots were maintained at a moisture content of 40% of the field capacity under glasshouse conditions. Three replicates were used for each treatment.Plant sampling and chemical analysis
During the 6-week cultivation period, plants were periodically examined for toxicity symptoms (brown spots appearing on the leaves). At harvest, the toxicity score was evaluated as: (4) more than two-thirds of the leaf area turned brown; (3) two-thirds to one-third of the leaf area turned brown; (2) less than one-third of the leaf area turned brown; (1) only the edges of the leaf blade turned brown; (0) no symptoms. The scores for individual leaves were totaled for each plant and divided by the total number of leaves in each plant (referred to as the normalized toxicity score). After cultivation, the shoots were excised and the roots were carefully separated from the soil, and their fresh and dry weights were determined.
A 0.5 g portion of the fresh roots from each plant was subjected to trypan blue staining for microscopic examination of mycorrhizal infection (CitationBrundrett 1994). The root length colonized by AMF was quantified using the gridline intersection method (CitationGiovennetti and Mosse 1980). The remaining roots and all the shoots were oven-dried at 90°C for 2 days and ground for chemical analysis. The tissues were digested using a sulfuric acid and hydrogen peroxide mixture in a Kjeldahl flask at 300°C and analyzed for As using hydride generation-inductively coupled plasma-atomic emission spectrometry (HG-ICP-AES; ICPS-1000IV equipped with HVG-1, Shimadzu, Kyoto, Japan). The detection limit for As was 1.0 µg L−1 in the digested sample solution. The P content was determined for the same digest using ICP-AES. To verify the accuracy of As and P determination, standard reference materials of pepperbush (CRM No.1) and sargasso (CRM No. 9) provided by the National Institute for Environmental Studies, Tsukuba, Japan, were analyzed. The recovery rates of As and P with respect to their certified values were 90–95%.
Soil sampling and chemical analysis
After the sunflower roots were sampled from the central compartment, the soils in the central and outer compartments were passed through a 2 mm sieve. Portions of 20 g were stored at 4°C for the analysis of acid phosphatase activity and dehydrogenase activity; the remaining soil was freeze-dried and stored at −30°C for chemical analysis.
Soil samples were shaken with distilled water (1:100) for 2 h at room temperature to obtain a water-soluble (WS) fraction. The WS fraction was analyzed for different forms of As using cold-trap vapor-reduction atomic absorption spectrometry (AA-6800 equipped with ASA-2sp, Shimadzu), and the concentrations of AsIII, AsV, monomethylarsonic acid (MMAA), dimethylarsinic acid (DMAA) and trimethylarsine oxide (TMAO) were determined (CitationMasscheleyn et al. 1991; CitationUltra et al. 2007). Calibration curves for each As species were prepared using standard solutions (Tri Chemical Laboratories, Yamanashi, Japan). The detection limit for each As species was 0.1 µg L−1 in the sample solution. Water-soluble P content was determined using ICP-AES in the same soil extract.
Acid phosphatase activity in the soil was estimated based on p-nitrophenol release after incubation with p-nitrophenyl phosphate (CitationAlef et al. 1995). In brief, 1 g of the soil sample was treated with 0.25 mL of toluene, 4 mL of modified universal buffer (MUB; pH 6) and 1 mL of 15 mmol L−1 p-nitrophenyl phosphate, and incubated for 1 h at 37°C. The MUB was prepared from a stock solution (fivefold strength) containing 12.1 g of Tris, 11.6 g of maleic acid, 14 g of citric acid and 6.3 g of boric acid dissolved in 500 mL NaOH (1 mol L−1); diluted with sterile distilled water. To terminate the reaction, 1 mL of 0.5 mol L−1 CaCl2 and 4 mL of 0.5 mol L−1 NaOH were added to the mixture prior to filtration. The absorbance at 400 nm resulting from p-nitrophenol was measured using a spectrophotometer (UV-1200, Shimadzu) and the acid phosphatase activity was expressed in mg (p-nitrophenol) g−1 h−1. The dehydrogenase activity was estimated based on the incubation of soil with an electron acceptor 2(p-iodophenyl)-3-(p-nitrophenyl)-5-phenyl tetrazolium chloride (INT) at 40°C for 2 h (CitationVon Mersi and Schinner 1991). The reaction product, iodonitrotetrazolium formazan (INF) was extracted using N,N-dimethylformamide and an ethanol mixture (1:1, v/v) and quantified using the spectrophotometer at 464 nm against a blank. Dehydrogenase activity was expressed in µg INF g−1 h−1.
Statistical analysis
For the data on toxicity symptoms, plant growth, and As and P concentrations, Tukey's tests were used to determine any significant differences (P ≤ 0.05) between the treatments. Main effects and interaction effects of treatments and compartments on As distribution and the proportion of different forms of As in the total WS-As were subjected to a two-way anova.
RESULTS
Plant growth, As toxicity and AM infection in roots
Arsenic toxicity symptoms began to develop in the sunflower plants 3 weeks after transplanting in the –AM/S treatment and approximately 4 weeks after transplanting in the other treatments. Initial symptoms were a browning at the edges of the leaf blades and red-brown necrotic spots on older leaves, followed by the senescence of the leaves. At harvest, the average normalized toxicity scores in the +AM treatments were lower compared with the –AM, and the symptoms were most clearly alleviated in the +AM/S treatment (). Shoot dry weight tended to increase with AMF inoculation and the highest value was obtained in the +AM/S treatment. Root dry weight in the S condition was relatively higher compared with the NS condition, regardless of AMF treatment. A significant increase in root dry weight as a result of AMF inoculation was only observed in the NS condition, while the value of the +AM/NS treatment was also higher than that of –AM/NS. The root length colonized in the +AM treatments was approximately 42% and 38% under S and NS conditions, respectively, while plants in the –AM/NS treatments showed less than 10% and no roots were colonized in the –AM/S treatment. The colonization observed in the –AM/NS treatment probably resulted from mycorrhizal fungi initially existing in the soil.
Concentration and contents of As and P in plants
The concentration of As in the shoots was significantly reduced by AMF inoculation under both S and NS conditions (). Shoot As content did not differ
Table 2 Normalized As toxicity scores, AM root length colonized, dry matter yield, and the As and P concentrations in shoots and roots of sunflower grown in As contaminated soil†
Table 3 Contents of As and P, ratios of shoot content to root content, and ratios of P content to As content in the shoots and roots of sunflower plants grown on As-contaminated soil†
Concentration of water soluble P in soil
The concentration of water soluble P (WS-P) in the soils after sunflower cultivation was lower in the central compartment than in the outer compartment, especially in the +AM treatments (). Regardless of the AMF inoculation, the concentrations under S conditions were slightly higher than NS conditions.
Concentration and forms of WS-As in soils
In general, the dominant As species in the WS fraction was AsV in the outer compartment and AsIII in the central compartment (). The lowest concentration of
Figure 2 Amounts of water soluble P (WS-P) in the soil collected from the rhizosphere and bulk compartments of the rhizobag system. Data are mean ± standard deviation for three replications. Bars on the top right indicate the least significant differences (LSD) for comparison between treatments (left), between compartments (middle) and between any pair of data (right) at P < 0.05. +AM, plus arbuscular mycorrhizal fungus inoculation; –AM, without arbuscular mycorrhizal fungus inoculation.
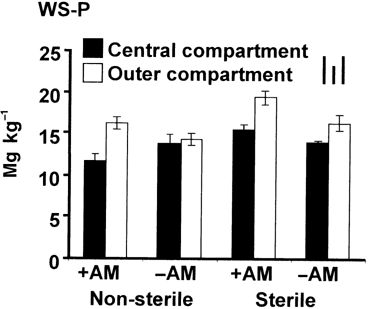
Figure 3 Amounts of (A) water soluble (WS)-AsIII and (B) WS-AsV in the soil collected from different compartments of the rhizobag. Bars on the top right indicate the least significant differences (LSD) for comparison between treatments (left), between compartments (middle) and between any pair of data (right) at P < 0.05. +AM, plus arbuscular mycorrhizal fungus inoculation; –AM, without arbuscular mycorrhizal fungus inoculation.
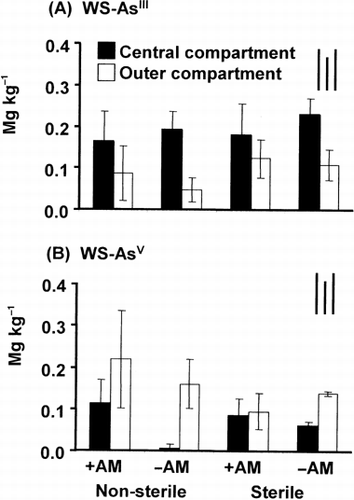
Under S conditions, WS-DMAA was detected only in the central compartment of the +AM treatment. The concentration of WS-DMAA and WS-TMAO in the +AM/NS treatment was significantly higher in the central compartment than in the outer compartment (). While WS-DMAA was only detected in the +AM treatments and the values in the +AM/NS treatment were higher than those in the +AM/S treatment, WS-TMAO was only detected under NS conditions with or without AM inoculation and the amounts in the central compartment were relatively higher than those in the outer compartment. WS-MMAA was not detected in any soil samples (data not shown).
Acid phosphatase and dehydrogenase activity in soils
Acid phosphatase activity in the soil samples from the central compartment was significantly higher than that
Figure 4 Amounts of (A) water soluble dimethylarsinic acid (WS-DMAA) and (B) water soluble trimethylarsine oxide (WS-TMAO) in the soil collected from different compartments of the rhizobag. Bars on the top right indicate the least significant differences (LSD) for comparison between treatments (left), between compartments (middle) and between any pair of data (right) at P < 0.05. +AM, plus arbuscular mycorrhizal fungus inoculation; –AM, without arbuscular mycorrhizal fungus inoculation.
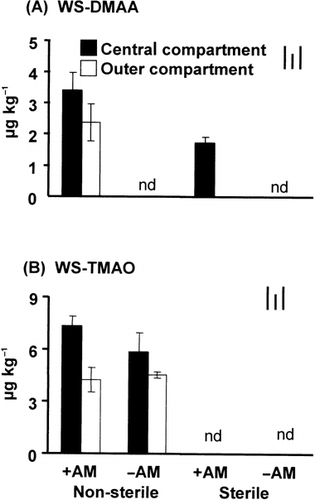
DISCUSSION
Sterilization of soils prior to experimental use resulted in the elimination of native mycorrhiza, while inoculated G. aggregatum successfully colonized sunflower roots even at high levels of As in the soils (). Root
Figure 5 (A) Acid phosphatase and (B) dehydrogenase activity in the soil collected from different compartments of the rhizobag planted with sunflower. Bars on the top right indicate the least significant differences (LSD) for comparison between treatments (left), between compartments (middle) and between any pair of data (right) at P < 0.05. +AM, plus arbuscular mycorrhizal fungus inoculation; –AM, without arbuscular mycorrhizal fungus inoculation; INF, iodonitrotetrazolium formazan.
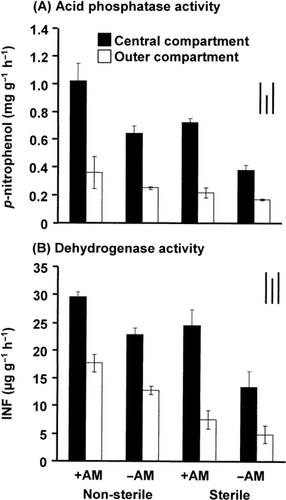
The AMF inoculation promoted plant growth and alleviated As toxicity symptoms in the sunflower plants, especially in the sterile soil. Higher growth in the sterile soil compared with the non-sterile soil could be attributed, in part, to an increase in nutrient availability in soils after sterilization (), especially P and NH4-N (CitationSakamoto et al. 1992; CitationYamamoto et al. 2006). However, regardless of soil sterilization, AMF inoculation increased plant P uptake, but lowered the As concentration in shoots as well as the shoot As uptake per root dry mass. In contrast, the As concentration in roots was relatively higher in the +AM treatments than in the –AM treatment, and a significant increase in root As content was observed as a result of AMF inoculation under NS conditions (,). As evident from the high P/As ratio in AMF inoculated plants (), AMF inoculation reduced the translocation of As to the shoots and promoted P uptake, and increased the tolerance against As toxicity through an As dilution effect by growth enhancement (CitationMeharg et al. 1994; CitationUltra et al. 2007).
These observations are consistent with our previous results and similar to the reports by CitationLeung et al. (2005) and CitationLiu et al. (2005), which showed that AMF restricted the transfer of As from the soil to the above-ground plant organs, resulting in reduced As concentration in shoots, although the overall As uptake was increased because of an AMF-induced increase in biomass. Several mechanisms have been proposed to explain how AMF affects As transport and translocation in tolerant and resistant plants. CitationGonzalez-Chaves et al. (2002) reported a reduction of AsV influx in AMF-colonized Holcus lanatus by suppression of a high-affinity phosphate/AsV transport system. In contrast, CitationSharples et al. (2000) showed that ericoid mycorrhizal fungus Hymenocyphus ericae decreased the net As uptake in plants by inducing AsIII efflux from roots. It is also plausible that AMF will be able to sequester As by binding on hypae and other fungal tissues in the roots, thus restricting the transport and translocation of As.
The amount of WS-P in the soil after sunflower cultivation indicates the residual effects of AMF inoculation, plant uptake, soil sterilization and their interaction. As we have already discussed the As–P dynamics in soil as affected by AMF (CitationUltra et al. 2007), the following discussion focuses on the role of AMF in As transformation.
Distribution of WS-As between the compartments was different for AsV and AsIII (). The increase in WS-AsIII in the central compartment could be attributed to several factors/processes, such as higher mobility of AsIII in the soil and transformation of As mediated by microorganisms in the rhizosphere (CitationCervantes et al. 1994; CitationFitz and Wenzel 2002). AsIII, which is more mobile than AsV (CitationHering and Dixit 2005; CitationMarschner 1995), could be carried by mass flow and accumulate with time in the rhizosphere, especially when transport exceeds uptake (CitationVetterlein and Jahn 2004).
In the case of AsV, a depletion zone could be formed near the root surfaces after plant uptake as with the case of P (CitationMarschner 1995). Therefore, a lower amount of WS-AsV in the central compared with the outer compartment could be explained in part by plant uptake. However, it should be noted that a significant difference between the central and the outside compartment occurred in the –AM and not in the +AM treatment. This observation suggests an AMF-induced modification of microbial activities and community structures in the mycorrhizosphere (CitationMarschner and Baumann 2003), which might have affected the speciation and dynamics of As.
In our previous experiment, we detected DMAA in the mycorrhizosphere of sunflower plants. Because methylated As species have been reported to be less toxic than inorganic As (CitationBentley and Chasteen 2002; CitationMukhopadhyay et al. 2002; CitationSchmidt et al. 2004), we hypothesized that the chemical behavior and toxicity of As are altered in the mycorrhizosphere, which contributes to better survival of mycorrhizal plants in As-contaminated soil (CitationUltra et al. in press). However, it has not yet been clarified whether mycorrhizal roots alone can methylate As or whether AMF can indirectly induce the biomethylation process by indigenous microorganisms in the rhizosphere. In this experiment, either DMAA or TMAO was detected in the +AM/S or +AM/NS treatments, and these organic As species were not detected in the –AM/S treatment. These facts clearly indicated that mycorrhizal roots were primarily involved in As biomethylation in the rhizosphere of sunflowers.
It is noteworthy that in the sterile soils, DMAA was detected only in the central compartment of +AM treatments, which would serve as evidence that mycorrhizal roots were capable of DMAA formation in the rhizosphere. However, our experimental system could not determine whether or not AMF in association with sunflower roots is primarily responsible for As biomethylation. According to a report by CitationRaab et al. (2005), sunflower plants can methylate As in the form of MMAA and DMAA. Therefore, we cannot deny the possibility that the DMAA detected in our experiment is formed by sunflowers or by some possible solubilization from other fractions in the soil by AMF or some other reason. However, taking into consideration the fact that DMAA was not detected in the central compartment of the –AM/S treatment, it is more likely that AM and mycorrhizal roots could synergistically enhance As biomethylation.
Furthermore, a higher concentration of WS-DMAA was detected in the central compartment compared to the outer compartment, especially in the +AM/NS treatment (). This suggests that the presence of AMF and mycorrhizal roots enhanced the capability of the indigenous microorganisms for biomethylation by means of stimulating their activity, especially in the rhizosphere (CitationTurpeinen et al. 1999). The number of microorganisms in the rhizosphere is typically one order of magnitude larger than in the non-rhizosphere soil because of the continuous input of root-derived organic substrate, resulting in a more diverse, active and synergistic community (CitationJeffries et al. 2002; CitationMarschner 1995). Concurrent evidence of higher microbial activity in the rhizosphere of +AM treatments is shown by high phosphatase and dehydrogenase enzyme activities ().
Trimethylarsine oxide was detected in measurable amounts only under NS conditions. This result would indicate that its formation is primarily mediated by indigenous microorganisms and less by AMF. However, in the central compartment of the +AM treatment, the concentration of WS-TMAO was higher than in the outer compartment of the +AM treatment and in the central compartment of the –AM treatment. These results imply that the presence of mycorrhizal roots has the ability to enhance TMAO formation. It has been generally accepted that DMAA is a precursor of TMAO (CitationThomas et al. 2004) and a substrate for fungal conversion to arsenobetaine and arsenolipids found in marine animals (CitationCervantes et al. 1994). Therefore, it would also be true in this experiment that TMAO was transformed from DMAA, which was converted from AsV. These As transformation processes could be possible mechanisms for As detoxification by AMF and sunflower roots. Thus, there is a possibility that AMF and mycorrhizal roots contribute to the formation of TMAO, indirectly through the supply of DMAA as a precursor of TMAO.
In conclusion, consistent with our previous report, AMF inoculation of sunflower grown on As-contaminated soil alleviated As toxicity by improving P nutrition and reducing As concentration in the shoots. It was clarified that mycorrhizal roots are primarily involved in DMAA formation, which would affect the fate of As in the rhizosphere soil. Aside from the mycorrhizal roots, indigenous soil microorganisms in the mycorrhizosphere may possibly be capable of promoting the transformation of DMAA into TMAO.
ACKNOWLEDGMENTS
This research was supported by a Sasakawa Scientific Research Grant (No. 17-207) from The Japan Science Society and by a Grant-in-Aid for Scientific Research (B, No. 15380223) from the Ministry of Education, Culture, Sports, Science and Technology (MEXT) of Japan. The authors gratefully acknowledge Dr Shingo Matsumoto, Shimane University, for his assistance in sampling the As-contaminated soils.
Notes
Present address: University of Eastern Philippines, Catarman, Northern Samar 6400, Philippines.
REFERENCES
- Abedin , MJ , Cotter-Howells , J and Meharg , AA . 2002 . Arsenic uptake and accumulation in rice (Oryza sativaL.) irrigated with contaminated water . Plant Soil , 240 : 311 – 319 .
- Meharg , AA . 2004 . Arsenic in rice – understanding a new disaster for south-east Asia . Trends Plant Sci , 9 : 415 – 417 .
- Yamazaki , C , Ishiga , H , Ahmed , F , Itoh , K , Suyama , K and Yamamoto , H . 2003a . Vertical distribution of arsenic in Ganges Delta sediments in Deuli Village, Bangladesh . Soil SciPlant Nutr , 49 : 567 – 574 .
- Yamazaki , C , Itoh , K , Higashi , N , Ishiga , H , Suyama , K and Yamamoto , H . 2003b . Arsenic extractability with phosphate and citrate from peat collected in Bangladesh . Soil SciPlant Nutr , 49 : 859 – 865 .
- Ali , MM , Ishiga , H and Wakatsuki , T . 2003 . Influence of soil type and properties on distribution and changes in arsenic contents of different paddy soils in Bangladesh . Soil SciPlant Nutr , 49 : 111 – 123 .
- Ma , LQ , Komar , KM , Tu , C , Zhang , W , Cai , Y and Kennelley , ED . 2001 . A fern that hyperaccumulates arsenic . Nature , 409 : 579 – 579 .
- Van , TK , Kang , Y Fukui , T . 2006 . Arsenic and heavy metals accumulation by Athyrium yokoscensefrom contaminated soils . Soil SciPlant Nutr , 52 : 701 – 710 .
- Bentley , R and Chasteen , TG . 2002 . Microbial methylation of metalloids: Arsenic, antimony and bismuth . MicrobiolMolBiolRev , 66 : 250 – 271 .
- Turpeinen , R , Pantsar-Kallio , M , Haggblom , M and Kairesalo , T . 1999 . Influence of microbes on the mobilization, toxicity and biomethylation of arsenic in soil . SciTotal Environ , 236 : 173 – 180 .
- Gadd , GM . 2004 . Microbial influence on metal mobility and application for bioremediation . Geoderma , 122 : 109 – 199 .
- Fitz , WJ and Wenzel , WW . 2002 . Arsenic transformation in the soil–rhizosphere–plant system: fundamentals and potential application to phytoremediation . JBiotech , 99 : 259 – 278 .
- Smith , SE and Read , DJ . 1997 . “ Section 1: Vesicular-arbuscular Mycorrhizas ” . In Mycorrhizal Symbiosis , 2nd edn , 126 – 159 . London : Academic Press. .
- Christie , P , Li , X and Chen , B . 2004 . Arbuscular mycorrhiza can depress translocation of zinc to shoots of host plants in soils moderately polluted with zinc . Plant Soil , 261 : 209 – 217 .
- Joner , EJ , Briones , R and Leyval , C . 2000 . Metal-binding capacity of arbuscular mycorrhizal mycelium . Plant Soil , 226 : 227 – 234 .
- Leyval , C , Turnau , K and Haselwandter , K . 1997 . Effect of heavy metal pollution on mycorrhizal colonization and function: physiological, ecological and applied aspects . Mycorrhiza , 7 : 139 – 153 .
- Li , XL , George , E and Marschner , H . 1991 . Extension of the phosphorus depletion zone in VA-mycorrhizal white clover in a calcareous soil . Plant Soil , 136 : 41 – 48 .
- Marschner , H . 1995 . Mineral Nutrition for Higher Plants , 2nd edn , San Diego : Academic Press .
- Meharg , AA and Hartley-Whitaker , J . 2002 . Arsenic uptake and metabolism in arsenic resistant and nonresistant plant species . New Phytol , 154 : 29 – 43 .
- Asher , CJ and Reay , PF . 1979 . Arsenic uptake by barley Hordeum vulgarecultivar zephyr seedlings . AustJPlant Physiol , 6 : 459 – 466 .
- Gonzalez-Chavez , C , Harris , PJ , Dodd , J and Meharg , AA . 2002 . Arbuscular mycorrhizal fungi confer enhanced arsenate resistance on Holcus lanatus . New Phytol , 155 : 163 – 171 .
- Liu , Y , Zhu , YG , Chen , BD , Christie , P and Li , LX . 2005 . Yield and uptake of arbuscular mycorrhizal tomato colonized by Glomus mosseaeBEG167 in As spiked soil under glasshouse conditions . EnvironInt , 31 : 867 – 873 .
- Meharg , AA , Naylor , J and Macnair , MR . 1994 . Phosphorus nutrition of arsenate-tolerant and nontolerant phenotypes of velvet grass . JEnvironQual , 23 : 234 – 238 .
- Sharples , JM , Meharg , AA , Chambers , SM and Cairney , JWG . 2000 . Mechanism of arsenate resistance in the ericoid mycorrhizal fungus Hymenoscyphus ericae . Plant Physiol , 124 : 1327 – 1334 .
- Ultra , VU , Tanaka , S , Sakurai , K and Iwasaki , K . 2007 . Effects of arbuscular mycorrhiza and phosphorus application on arsenic toxicity in sunflower (Helianthus annuusL.) and on the transformation of arsenic in the rhizosphere . Plant Soil , 290 : 29 – 41 .
- Adriano , DC . 2001 . Trace Elements in the Terrestrial Environment , New York : Springer .
- Jeffries , P , Gianinazzi , S , Perotto , S , Turnau , K and Barea , JM . 2002 . The contribution of arbuscular mycorrhizal fungi in sustainable maintenance of plant health and soil fertility . BiolFertile Soils , 37 : 1 – 16 .
- Barea , JM , Pozo , MJ , Azcon , R and Azcon-Aguilar , C . 2005 . Microbial co-operation in the rhizosphere . JExpBot , 56 : 1761 – 1778 .
- Frankenberger , WT and Arshad , M . 2002 . “ Volatilization of arsenic ” . In Environmental Chemistry of Arsenic , Edited by: Frankenberger , WT . 363 – 380 . New York : Marcel Dekker .
- Brundrett , M , Melville , L and Peterson , L . 1994 . Practical Methods in Mycorrhiza Research , Guelph, Ontario, , Canada : Mycologue Publications .
- Giovannetti , M and Mosse , B . 1980 . An evaluation of techniques for measuring vesicular arbuscular infection in roots . New Phytol , 84 : 489 – 500 .
- Masscheleyn , PH , Delaune , RD and Patric , WH . 1991 . A hydride generation atomic absorption technique for arsenic speciation . JEnvironQual , 20 : 96 – 100 .
- Alef , K , Nannipieri , P and Trazar-Cepeda , C . 1995 . “ Phosphatase activity ” . In Methods in Applied Soil Microbiology and Biochemistry , Edited by: Alef , K and Nannipieri , P . 335 – 344 . London : Academic Press .
- Von Mersi , W and Schinner , F . 1991 . An improved and accurate method for dehydrogenase activity of soils with iodonitrotetrazolium chloride . Biol. Fertil. Soils , 11 : 216 – 220 .
- Sakamoto , K , Yoshida , T and Satoh , M . 1992 . Comparison of carbon and nitrogen mineralization between fumigation and heating treatments . Soil SciPlant Nutr , 38 : 133 – 140 .
- Yamamoto , T , Tanaka , S , Iwasaki , K and Sakurai , K . Effects of methyl bromide and chloropicrin fumigation and steam sterilization on soil nitrogen dynamics and microbial community . Proc. of the 18th World Congress in Soil Science . July 9–15 , Philadelphia, USA.
- Leung , HM , Ye , ZH and Wong , MH . 2005 . Interactions of mycorrhizal fungi with Pteris vittata(As hyperaccumulator) in As-contaminated soils . EnvironPollut , 139 : 1 – 8 .
- Cervantes , C , Ji , G , Ramires , JL and Silver , S . 1994 . Resistance to arsenic compounds in microorganisms . FEMS MicrobiolRev , 15 : 355 – 367 .
- Hering , JG and Dixit , S . 2005 . “ Contrasting sorption behavior of arsenic (III) and arsenic (V) in suspensions of iron and aluminum oxyhydroxides ” . In Advances in Arsenic Research: Integration of Experimental and Observational Studies and Implications for Mitigation , Edited by: O'day , PA , Vlassopoulos , D Meng , X . 8 – 24 . Washington, DC : ACS . ACS Symposium Series 915
- Vetterlein , D and Jahn , R . 2004 . Gradients in soil solution composition between bulk soil and rhizosphere – In situ measurement with changing soil content . Plant Soil , 258 : 307 – 317 .
- Marschner , P and Baumann , K . 2003 . Changes in bacterial community structure induced by mycorrhizal colonisation in split-root maize . Plant Soil , 251 : 279 – 289 .
- Mukhopadhyay , R , Rosen , BP , Phung , LT and Siver , S . 2002 . Microbial arsenic: from geocycle to gene and enzymes . FEMS MicrobiolRev , 26 : 311 – 325 .
- Schmidt , AC , Mattusch , J , Reisser , W and Wennrich , R . 2004 . Uptake and accumulation behavior of angiosperms irrigated with solution of different arsenic species . Chemosphere , 56 : 305 – 313 .
- Raab , A , Schat , H , Maharg , AA and Feldman , J . 2005 . Uptake, translocation and transformation of arsenate and arsenite in sunflower (Helianthus annuss): formation of arsenic–phytochelatin complex during exposure to high arsenic concentrations . New Phytologist , 168 : 551 – 558 .
- Thomas , DJ , Waters , SB and Styblo , M . 2004 . Elucidating the pathway for arsenic methylation . Toxic Applied Pharma. , 194 : 319 – 326 .
- Inskeep , WP , McDermott , TR and Fendorf , S . 2002 . “ Arsenic(V)/(III) cycling in soils and natural waters: chemical and microbiological process ” . In Environmental Chemistry of Arsenic , Edited by: Frankenberger , WT . 183 – 216 . New York : Marcel Dekker .
- Present address: University of Eastern Philippines, Catarman, Northern Samar 6400, Philippines.