Abstract
There is limited knowledge about the differences in carbon availability and metabolic quotients in temperate volcanic and tropical forest soils, and associated key influencing factors. Forest soils at various depths were sampled under a tropical rainforest and adjacent tea garden after clear-cutting, and under three temperate forests developed on a volcanic soil (e.g. Betula ermanii and Picea jezoensis, and Pinus koraiensis mainly mixed with Tilia amurensis, Fraxinus mandshurica and Quercus mongolica), to study soil microbial biomass carbon (MBC) concentration and metabolic quotients (qCO2, CO2-C/biomass-C). Soil MBC concentration and CO2 evolution were measured over 7-day and 21-day incubation periods, respectively, along with the main properties of the soils. On the basis of soil total C, both CO2 evolution and MBC concentrations appeared to decrease with increasing soil depth. There was a maximal qCO2 in the 0–2.5 cm soil under each forest stand. Neither incubation period affected the CO2 evolution rates, but incubation period did induce a significant difference in MBC concentration and qCO2 in tea soil and Picea jezoensis forest soil. The conversion of a tropical rainforest to a tea garden reduced the CO2 evolution and increased the qCO2 in soil. Comparing temperate and tropical forests, the results show that both Pinus koraiensis mixed with hardwoods and rainforest soil at less than 20 cm depth had a larger MBC concentration relative to soil total C and a lower qCO2 during both incubation periods, suggesting that microbial communities in both soils were more efficient in carbon use than communities in the other soils. Factor and regression analysis indicated that the 85% variation of the qCO2 in forest soils could be explained by soil properties such as the C:N ratio and the concentration of water soluble organic C and exchangeable Al (P < 0.001). The qCO2 values in forest soils, particularly in temperate volcanic forest soils, decreased with an increasing Al/C ratio in water-soluble organic matter. Soil properties, such as exchangeable Ca, Mg and Al and water-soluble organic C:N ratio, were associated with the variation of MBC. Thus, MBC concentrations and qCO2 of the soils are useful soil parameters for studying soil C availability and microbial utilization efficiency under temperate and tropical forests.
INTRODUCTION
Tree species can have significant impacts on soil fertility and microbial community structure (CitationDickinson and Pugh 1974; CitationSwift et al. 1979), which can in turn affect soil microbial biomass and microbial efficiency in carbon (C) use (e.g. CitationBauhus et al. 1998; CitationBlagodatskaya and Anderson 1998; CitationChen and Xu 2005; CitationPinzari et al. 1998). Microbial C, especially the microbial C : organic C ratio, can reveal the status of microorganisms in the C availability of soils (e.g. CitationInsam and Domsch 1988), and is considered to be a quantitative indicator for carbon dynamics in soils (CitationAnderson and Domsch 1985; CitationInsam and Haselwandter 1989; CitationXu et al. 2006). In temperate volcanic forest soils, it has been shown that metal–humus complexes (e.g. Al) are resistant to microbial decomposition and can offer physical protection against microbial breakdown (CitationShoji et al. 1993). The Al/C ratio of dissolved organic matter can be considered to be an important parameter for its stability against microbial decomposition (CitationBoudot et al. 1989; CitationSchwesig et al. 2003; CitationShoji et al. 1993). The quality and amount of litter and changes in soil properties can affect soil carbon availability and microbial utilization efficiency (e.g. CitationAnderson and Domsch 1993; CitationBlagodatskaya and Anderson 1998; CitationChen and Xu 2005; CitationHuygens et al. 2005; CitationSix et al. 2002). In tropical forests, high temperatures and rainfall increase soil C mineralization and nutrient leaching and, thus, increasing acidity can induce an accumulation of Al in soil profiles and a reduction of soil Ca and Mg. These variations are associated with the stability of metal–humus complexes and aggregate-C in the soil and their microbial decomposition (CitationAran et al. 2001; CitationHuygens et al. 2005; CitationSchwesig et al. 2003; CitationSix et al. 2002). There are usually lower concentrations of soil water-soluble organic C and total C under tropical forests than under temperate forests because of climatic conditions, which can affect soil C availability and microbial utilization. However, there is very limited knowledge about the differences in microbial biomass C and metabolic status in temperate volcanic and tropical forest soils.
On the basis of microbial C concentration, soil basal respiration (qCO2) is considered to be an alternative measurement of changes in microbial biomass in response to disturbance and environmental limitations (CitationAnderson and Domsch 1985; CitationInsam and Haselwandter 1989; CitationOdum 1985; CitationWardle and Ghani 1995). The qCO2 described by CitationOdum (1969) and by CitationAnderson and Domsch (1985) indicates the relative efficiency of soil microorganisms in carbon use measured during a short-term incubation (CitationAnderson and Domsch 1993; CitationWardle and Ghani 1995), and the intensity of carbon mineralization (CitationDilly and Munch 1998). CitationBardgett and Shine (1999) showed that the metabolic quotient was significantly affected by changes in litter diversity, being lower at higher levels of litter diversity. CitationAnderson and Domsch (1993) reported that qCO2 values decreased in a mixed forest ecosystem compared with a single plantation forest ecosystem. In earlier studies, CitationInsam and Haselwandter (1989) showed that the soil qCO2 in an ecosystem decreases during succession. However, to date, our knowledge is limited with regard to the differences in qCO2 under tropical and temperate forests at advanced succession stages. Indeed, a thorough discussion of the relationships between microbial C, qCO2 and soil properties in tropical and temperate forests is lacking. This is even true for the specific features of temperate volcanic and tropical forest soils, with a low microbial C : organic C ratio.
The objective of this work was to study the differences in soil microbial C and qCO2 in temperate volcanic and tropical forests, and the effects of soil properties on these biological variables. These results will improve our understanding of C availability and microbial utilization efficiency in temperate volcanic and tropical forest soils.
MATERIALS AND METHODS
Soil and litter sampling and chemical analysis
To examine the differences in soil carbon availability and metabolic quotient under different climatic conditions and in response to tree species, temperate and tropical forest soils at various depths were sampled near the Research Station of Changbai Mountain Forestry Ecology (128°28′E, 42°24′N) and the Research Station of Xishuangbanna Tropical Rainforest Ecosystem (101°16′E, 21°56′N), respectively. The area around the Changbai Mountain is a temperate, continental climate, with a long-term cold winter and warm summer. Annual mean temperature varies from 2.8°C at the bottom of the mountain to −7.3°C near the volcanic lake at the top, and annual mean precipitation varies from 750 to 1340 mm. Recent small-scale volcano eruptions occurred in 1597, 1668, 1702 and a very large-scale eruption occurred during 1000–1410 (CitationLiu et al. 1992; CitationZhao 1981). Three natural temperate forests in a natural vertical distribution along the northern slope were considered to be broadleaved Korean pine mixed forest (Pinus koraiensis mainly mixed with Tilia amurensis, Fraxinus mandshurica and Quercus mongolica, > 200 years old, altitude 740 m), spruce (Picea jezoensis, > 200 years old, altitude 1680 m) and birch (Betula ermanii, > 200 years old, altitude 1910 m). The mixed forest is now at advanced succession stages in this zone. Soil sampling was done after selecting three 4 m × 4 m plots in each forest stand. Samples of seven soil layers, 0–2.5, 2.5–5, 5–7.5, 7.5–10, 10–12.5, 12.5–15 and 15–20 cm, were collected separately within each plot in July 2005 using a thin stainless spade, when above-ground plant debris was removed. Thus, in total 63 temperate forest soil samples were taken simultaneously. In the tropical zone, the annual mean temperature is approximately 22°C, and annual mean precipitation is approximately 1400 mm, with an obvious dry and wet season over the year. Samples of the corresponding seven soil layers were collected in May 2005 under a seasonal tropical rainforest and adjacent tea garden, as previously described. A substantial proportion of the tree species in the seasonal rainforest (> 200 years old, altitude 720 m) is deciduous under the monsoon climate, although they do not shed leaves during the same seasons (CitationCao et al. 1996). The undisturbed rainforest has entered an advanced succession stage in this tropical zone. The tea garden was established approximately 60 years ago on the site after the existing rainforest was harvested. Fresh soils were kept separately in plastic bags and rapidly transported to the laboratory. Four 4 m × 4 m plots were selected under each stand, and one mixture of litter within each plot was collected. Fresh moist soils were sieved (2 mm, or 4 mm for 0–2.5 cm temperate soils) to remove small stones and roots, and stored in the dark at 4°C prior to incubation.
Temperate and tropical forest soils were classified as Andosols and Oxisols (Food and Agriculture Organization soil classification), respectively. Soil water-holding capacity was determined by saturating each soil in glass funnels with water and allowing drainage to field capacity under cover for 2–3 h at ambient temperature. Duplicate soils were dried at 105°C for 24 h to determine moisture content, and litter samples were dried at 70°C for 24 h to make powder for use. Total C and N concentrations in soil and litter samples were measured using a CN analyzer (MT-700 with an Auto Sampler MTA-600, Yanaco, Kyoto, Japan). Fresh soil pH (soil : water, 1:2.5) was measured using a portable pH meter. Soil water soluble organic C and total N concentrations were extracted with deionized water at a soil : water ratio of 1:5 (w/w) and measured using a TOC/TN-analyzer (Shimadzu TOC-VCPH/TN, Kyoto, Japan). Soil exchangeable metals such as Ca, Mg and Al were extracted by shaking 10-g fresh soil with a 50-mL 1 mol L−1 KCl solution for 60 min on an end-over-end shaker, and then filtered into 50-mL plastic bottles. Concentrations of these metals in the filtrates were measured using inductively coupled plasma-mass spectrometry. The main soil properties under temperate and tropical forests are presented in . Total C and N concentrations in litter samples are presented in .
Soil respiration and microbial C during two incubation periods
All fresh soil samples taken from the forest floors were used to study soil respiration and microbial biomass carbon (MBC) concentration. Soil moisture contents were adjusted to 50–55% (w/w) (approximately 45% water-holding capacity) after 20-g samples of fresh soils (in triplicate) were added to 250-mL glass bottles and sealed with butyl rubber stoppers. This consistency of soil water content can reduce the uncertainty about the proportion of microbial biomass released by the fumigation–extraction (CitationDavidson et al. 1989; CitationSparling et al. 1990). Control bottles containing no soil were used as blanks to derive CO2 production from the soils. To compare the effects of incubation time on microbial C and qCO2 in soil, the oxic incubation was done at 25°C in the dark for 7 and 21 days, respectively. Three milliliters of headspace gas was sampled from each bottle 4, 7, 11, 14, 18 and 21 days after initiating the incubation, and immediately injected into a gas chromatograph for CO2 measurement; the rubber stoppers were removed from the bottles for 2 h to refresh the air. At the end of the 7-day and 21-day incubations, soil MBC concentrations were measured using the chloroform fumigation–extraction method (CitationVance et al. 1987). Soil salt-extractable organic C was extracted by shaking 5.0-g fresh soil with a 25-mL 0.5 mol L−1 K2SO4 solution for 60 min on an end-over-end shaker. The mixtures were centrifuged at 6400 g for 5 min and then filtered into 50-mL plastic bottles. The filtrate was analyzed for organic C using a TOC/TN-analyzer (Shimadzu TOC-VCPH/TN).
Carbon dioxide concentrations in the headspace were quantified using a modified gas chromatograph (Agilent 5890) equipped with a flame ionization detector (FID). Carbon dioxide was separated using one stainless steel column (2 m length and 2.2 mm internal diameter) that was packed with 50–80 mesh porapack Q, hydrogen was then used to reduce CO2 to CH4 in a Nickel catalytic converter at 375°C, and the CH4 was detected using the FID. The oven was operated at 55°C and the FID at 200°C, and N2 was used as a carrier gas at a flow rate of 20 cm3 min−1. The CO2 concentration was calculated using a linear calibration using a certified CO2 concentration that contains 0.400% CO2 in N2.
Calculation and statistical analysis
Soil respiration rates were obtained from the increase in cumulative CO2 concentration (minus the blank) against incubation time, and were expressed on the basis of initial soil total C. Because the ratio of soil microbial C to total C concentration reflects the linkage and interaction between the two parameters (CitationInsam and Domsch 1988), MBC levels were calculated as the proportion of initial soil total C using the equation: biomass C = 2.22 × E c, where E c is the difference in K2SO4-extractable organic C between fumigated and non-fumigated samples (CitationVance et al. 1987; CitationWu et al. 1990). The metabolic quotient (qCO2) was calculated as the ratio of microbially respired C (µg g−1 over the 7 and 21 days) to MBC and used as an indicator of microbial metabolism in soil. Means of three replicates and standard errors were calculated. Significant differences between means were analyzed using a t-test (STATISTICA software for Windows [release 4.5]), with a confidence interval of 95%. Principal component and regression analyses were carried out to explain some soil properties that affect microbial respiration, MBC and qCO2 in forest soils. Least significant differences (LSD) were calculated at the 5% level to assess the differences in soil properties with depth.
RESULTS
Soil and litter properties under tropical and temperate forests
Some of the properties of temperate and tropical forest soils at different depths are presented in . The concentrations of total C and N and water soluble organic C in temperate forest soils were much larger than those in tropical forest soils (P ≤ 0.05), and were reduced at greater rates with increasing soil depth, compared to the reduction in the concentrations in tropical forest soils. Comparing selected temperate forest stands, the results showed that the broadleaved Korean pine mixed forest soil at less than 20 cm depth had a lower soil C:N ratio and lower water soluble organic C concentration (P ≤ 0.05) than the birch and spruce forest soils. Soil exchangeable Ca and Mg concentrations under temperate forests, especially under the broadleaved Korean pine mixed forest, were much larger than those in tropical forest soils (P ≤ 0.05), and there was a sharp reduction in these concentrations with increasing soil depth (P ≤ 0.05). This is contrary to the change in exchangeable Al concentration in the 0–20 cm soil. The accumulation of soil exchangeable Al became larger with increasing soil depth, and its maximal concentration occurred in sub-surface soils under spruce and birch (P ≤ 0.05). The exchangeable Al concentration in tropical forest soil was much larger than that in temperate forest soil (P ≤ 0.05), which was contrary to the variation in soil pH. There was a larger total N concentration in litter under birch forest and tea garden than under the other forests, and a similar total C concentration occurred in all the litters, with the exception of the litter under the broadleaved Korean pine mixed forest (P ≤ 0.05) (). There was a lower C:N ratio in the litter under birch forest and tea garden than under the other forests (P ≤ 0.05) ().
Microbial respiration, microbial carbon and metabolic quotients in forest soils
Soil microbial respiration and microbial C concentration were calculated according to initial soil total C. shows the variations of microbial respiration, microbial C and metabolic quotients in temperate and tropical forest soils during the 7-day and 21-day incubations. On the basis of soil total C, CO2 evolution rates and MBC concentrations appeared to decrease with increasing soil depth (P ≤ 0.05). There was a maximal qCO2 in the 0–2.5 cm soil under each forest stand. Both incubation periods had no impact on microbial respiration, but did affect microbial C and qCO2 measurements in tea soil and spruce forest soil. The rainforest conversion to tea garden increased soil microbial respiration and qCO2, resulting in an increase in microbial C utilization inefficiency (P ≤ 0.05). Comparing selected temperate forests, the results showed that the broadleaved Korean pine mixed forest soil at less than 20 cm depth was characterized by lower microbial respiration relative to soil total C and lower qCO2, and with a larger MBC concentration relative to soil total C during both incubations (P ≤ 0.05). This indicated that microbial communities in the soil were more efficient in carbon use than communities in the other soils. With the exception of the 0–2.5 and 2.5–5.0-cm soil layers, there was no difference in qCO2 values in the broadleaved Korean pine mixed forest and rainforest soils at depths.
Relationship between microbial carbon and metabolic quotients and soil properties
Variations in soil microbial C concentrations were associated with soil properties, such as exchangeable Ca, Mg and Al, and the concentration ratios of water soluble organic C to total N (). The metabolic quotients appeared to increase with increasing C:N ratio and water soluble organic C concentration in the soil (P < 0.0001) (), but decreased with increasing soil exchangeable Al concentration (P = 0.002) (). The qCO2 values in forest soils, particularly in temperate volcanic forest soils, decreased with an increasing Al/C ratio of water soluble organic matter (P < 0.01) ().
DISCUSSION
Two eigenvectors from a principal component analysis reveal that both microbial biomass C and metabolic quotients were mainly associated with soil properties such as C:N ratio, exchangeable Ca, Mg and Al, and water soluble organic C; neither the 7-day nor the 21-day incubation affected the correlation (). Hence, the 7-day incubation can reflect the change in microbial biomass C and metabolic quotients of the soils (). Considering that there are small variations in the soil properties after a 7-day incubation (data not shown), soil properties such as C:N ratio, exchangeable Ca, Mg and Al, and soluble organic C can, thus, affect soil microbial biomass C and metabolic quotients under temperate and tropical forests.
The broadleaved Koran pine mixed forest soil had a larger microbial C relative to soil total C and a lower
Table 1 Main properties of the temperate and tropical soils at various depths
Table 2 Total C and N concentrations of litter under different forest stands
Figure 1 Effects of tree species and soil depths on (a) microbial respiration, (b) microbial biomass C and (c) metabolic quotient in temperate and tropical soils during the 7-day (▪) and 21-day incubations (□). Error bars are the standard error of three replicates.
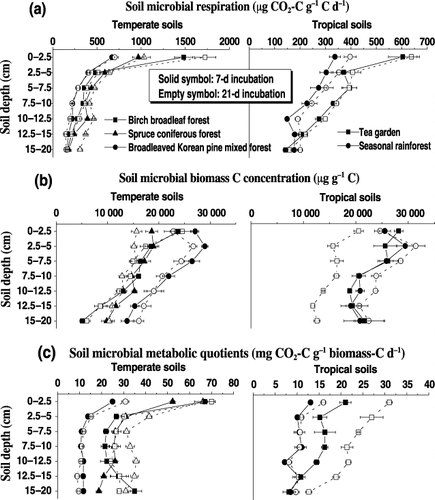
Figure 2 Relationship between soil microbial biomass C concentrations in the 7-day incubation to the concentrations of exchangeable (a) Ca, (b) Mg and (d) Al, and to (c) the ratios of water soluble organic C to total N concentration in temperate and tropical soils at various depths. Error bars are the standard error of three replicates. The linear or non-linear regression indicates the microbial C, y, against (the concentrations of soil exchangeable Ca, Mg and Al, or the ratios of soil water soluble organic C to total N concentration), x.
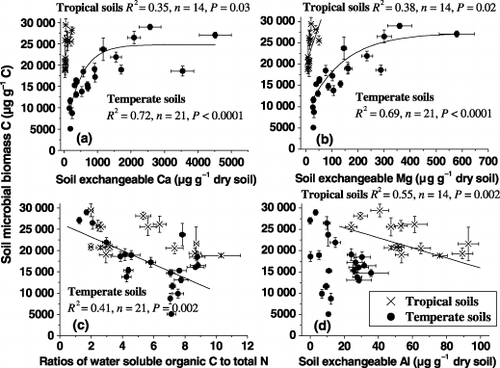
Figure 3 Relationships between microbial metabolic quotients in the 7-day incubation to (a) the total C:N ratios, (b) the concentrations of water soluble organic C and (c) exchangeable Al, and to (d) the ratios of exchangeable Al to water soluble organic C concentration in temperate and tropical soils at various depths. Error bars are the standard error of three replicates. The linear or non-linear regression indicates the metabolic quotients, y, against (the total C:N ratios, the concentrations of water soluble organic C and exchangeable Al, or the ratios of exchangeable Al to water soluble organic C concentration in the soil), x.
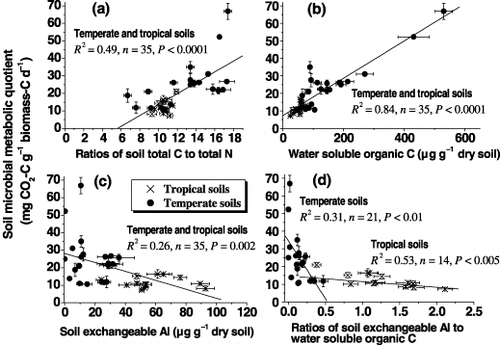
Table 3 Two eigenvectors from a principal component analysis of the standardized data of different soil chemical and biological properties under temperate and tropical forests
where y is the microbial metabolic quotient (qCO2, mg CO2-C g−1 biomass-C day−1) and a, b and c represent C:N ratio, the concentration of water soluble organic C and exchangeable Al (µg g−1 dry soil), respectively, in the soil.
where y is the microbial metabolic quotient (qCO2, mg CO2-C g−1 biomass-C day−1) and a, b and c represent C:N ratio, water soluble organic C : total N ratio and exchangeable Al concentration (µg g−1 dry soil), respectively, in the soil.
The regression models were both significant and explained 85% and 65% of the variability of qCO2 in the soils, respectively. It is clear that soil C:N ratio had a larger contribution to the qCO2 than soil water soluble organic C and exchangeable Al (EquationEqs 1,Equation2). It is noteworthy to mention that under experimental conditions, qCO2 values of forest soils appeared to increase with increasing soil C:N ratio () and with increasing water soluble organic C (). This is to say that great microbial inefficiency in carbon use can occur in forest soils associated with large soil C:N ratios and with large soluble organic C concentrations (EquationEqs 1,Equation2). This was in good agreement with our previous results using Japanese temperate volcanic forest soils (CitationXu et al. 2006). A lower concentration of water soluble organic C and lower C:N ratio through the soil profile under the broadleaved Koran pine mixed forest than under birch and spruce () can, thus, explain a lower qCO2 value in the soil ().
There was a significantly positive relationship between qCO2 and soil pH (r = 0.47, P < 0.01), and between qCO2 and exchangeable Ca concentration (r = 0.38, P ≤ 0.05), in the soils at various depths. This relationship did not change when temperate and tropical forest soils were separated (data not shown). This mainly resulted from a large qCO2, soil pH and exchangeable Ca concentration in the 0–2.5-cm soil under each forest stand (, ). However, in the soil profiles under temperate and tropical forests, qCO2 was negatively correlated with exchangeable Al concentration (r = −0.51, P = 0.002). Hence, high specific microbial respiration may be indicative of acid stress rather than nutrient stress in the soils. This will, however, be difficult to distinguish under field conditions because acid soils usually have a poorer nutrient status than soils with higher pH.
Microbial metabolic quotient is considered to be an index for the evaluation of the efficiency of soil microbial communities for substrate utilization (CitationInsam 1990). The more efficiently the microorganisms function, the greater the fraction of substrate C that is incorporated into biomass and the lower the C per unit biomass that is lost through respiration. This can result in a low metabolic quotient. Hence, the relatively lower qCO2 under the mixed temperate forest and the rainforest compared with the simple plantation (Picea, Betula) forest (P ≤ 0.05) () can reflect an increase in the efficiency of substance utilization by the soil microbial community. The large microbial C utilization in the mixed temperate forest soil was in accordance with the results reported by CitationAnderson and Domsch (1993), who observed that qCO2 decreased in a mixed forest ecosystem compared with a simple plantation forest ecosystem. Litter under tropical and temperate forests contained different total C and N concentrations, and different C:N ratios (). The quality and decomposition of litter can affect metal ions, soluble organic C and C:N ratios in surface soils (), thus affecting the qCO2.
There is general consensus that the interaction of soil organic matter with metal ions such as Al3+ and Fe3+ is the main reason for the stability of soil organic matter in soils against microbial biodegradation (CitationLundström et al. 2000; CitationNierop et al. 2002; CitationZysset and Berggren 2001). Microbial C utilization in the soils can be influenced not only by Al concentration, but also by its combination with soluble organic C. shows the relationship between qCO2 and the concentration ratios of soil exchangeable Al to water soluble organic C. The qCO2 values in forest soils, particularly in temperate volcanic forest soils, decreased with an increasing Al/C ratio of water soluble organic matter. The Al/C ratio of dissolved organic matter can be considered to be an important parameter for its stability against microbial decomposition (CitationBoudot et al. 1989; CitationSchwesig et al. 2003). In long-term incubation studies, CitationSchwesig et al. (2003) showed that for natural dissolved organic matter, Al/C ratios > 0.1 increased the half-life of the stable dissolved organic matter fraction up to fourfold. Hence, Al concentration in combination with dissolved organic C can affect soil microbial C utilization and the stability of dissolved organic matter under temperate and tropical forests.
The parameter soil microbial C : organic C ratios might provide additional information on the differences in carbon availability because this parameter is less affected by storage conditions. The ratios respond readily to disturbance effects and can provide an effective warning on the deterioration of soil quality (CitationBauhus et al. 1998; CitationInsam and Domsch 1988; CitationPinzari et al. 1998; CitationPriha and Smolander 1997; CitationValsecchi et al. 1995). Both the 7-day and 21-day incubations could induce a significant difference in MBC concentration and qCO2 in tea soil and spruce forest soil (). Hence, the same incubation conditions are needed to examine differences in soil carbon availability and microbial utilization status under different climatic conditions and forests. Microbial biomass C concentrations under temperate and tropical forests appeared to increase with increasing exchangeable Ca and Mg concentration in soil, and at high concentrations, the increase in soil C availability slowed under temperate forests (). This indicates that the addition of Ca and Mg (e.g. gypsum) can improve microbial C availability in soils, particularly in tropical forest soils. The comparatively large microbial C relative to soil total C under the broadleaved Koran pine mixed forest indicated good microbial growth in the soil (), which was, in part, attributed to larger concentrations of exchangeable Ca and Mg under the mixed forest than under the birch and spruce forests (P ≤ 0.05) ().
Stepwise multiple regression analysis indicated that variations in microbial biomass C concentrations in temperate and tropical forest soils can be explained by soil properties such as the water soluble organic C : total N ratio, and the concentration of exchangeable Mg and Al. The multiple linear regressions were expressed as follows:
where y is microbial biomass C (µg g−1 C) and a, b and c represent the water soluble organic C : total N ratio and the concentration of exchangeable Mg and Al (µg g−1 dry soil), respectively, in the soil.
The regression model was significant and explained 49% of the variability in microbial biomass C in the soils. It is clear that the soil water soluble organic C : total N ratio had a larger contribution to the microbial C concentration than the concentration of soil exchangeable Mg and Al (EquationEqn 3). As shown in , there was a significantly negative relationship between microbial biomass C concentration and exchangeable Al concentration in tropical forest soils (r = −0.62, P = 0.002). However, no obvious relationship between either variable was observed when temperate and tropical soils were combined (). This is different from the relationship indicated by EquationEq. 3. Although there was a negative relationship between exchangeable Al concentration and soil respiration (r = −0.38, P ≤ 0.05), the Al toxic effects on soil microorganisms varied with the experimental conditions and with soil properties such as soluble carbon and pH. Many studies have shown that soil microbial communities and growth and root biomass can affect soil respiration and carbon availability (e.g. CitationLi et al. 2000). Further understanding of the interactions between soil microbial activities, microbial biomass and soil properties is necessary to improve the prediction of C cycling processes in soil. CitationNeill and Gignoux (2006) have showed that microbial growth should be considered when modeling carbon processes in soil. Considering that the Al effects on soil carbon availability and microbial biomass are complicated, it is vital that we further examine the effects of Al ions on carbon availability and microbial utilization in temperate volcanic and tropical forest soils.
CONCLUSIONS
Comparing selected temperate and tropical forests, our results showed that broadleaved Korean pine mixed forest and rainforest soils at less than 20 cm depth had a larger MBC concentration relative to soil total C during short-term incubations, and a lower qCO2, suggesting that microbial communities in both forest soils were more efficient in carbon use than the communities in the other soils. The conversion of a tropical rainforest to a tea garden reduced CO2 evolution and increased the qCO2 in the soil. The results indicated that the variations of qCO2 and microbial C in temperate and tropical forest soils were mainly attributable to soil properties such as C:N ratio, soluble organic C, exchangeable Ca, Mg and Al ions.
ACKNOWLEDGMENTS
This work was funded by the National Natural Sciences Foundation of China (Grant No. 20477044) and by the Hundred Talents Project from the Chinese Academy of Sciences. The authors thank Dr Sha L.Q. from the Xishuangbanna Tropical Botanical Garden and Professor Han S.J. from the Research Station of Changbai Mountain Forest Ecology for their assistance and support with soil sampling.
REFERENCES
- Dickinson , CH and Pugh , GJF . 1974 . Biology of Plant Litter Decomposition , Vol. 1–2 , London : Academic Press .
- Swift , MJ , Heal , OW and Anderson , JM . 1979 . Decomposition in Terrestrial Ecosystems , Oxford : Blackwell .
- Bauhus , J , Pare , D and Cote , L . 1998 . Effects of tree species, stand age and soil type on soil microbial biomass and its activity in a southern boreal forest . Soil BiolBiochem , 30 : 1077 – 1089 .
- Blagodatskaya , EV and Anderson , TH . 1998 . Interactive effects of pH and substrate quality on the fungal-to-bacterial ratio and qCO2of microbial communities in forest soils . Soil BiolBiochem , 30 : 1269 – 1274 .
- Chen , CR and Xu , ZH . 2005 . Soil carbon and nitrogen pools and microbial properties in a 6-year-old slash pine plantation of subtropical Australia: impacts of harvest residue management . ForEcolManage , 206 : 237 – 247 .
- Pinzari , F , Trinchera , A , Benedetti , A and Sequi , P . 1998 . Defining soil quality in Mediterranean forest systems: microbial biomass activity . Fresenius EnvironBull , 7 : 447 – 457 .
- Insam , H and Domsch , KH . 1988 . Relationship between soil organic carbon and microbial biomass on chronosequences of reclamation sites . MicrobiolEcol , 15 : 177 – 188 .
- Anderson , TH and Domsch , KH . 1985 . Determination of eco-physiological maintenance requirements of soil microorganisms in a dormant state . BiolFertilSoils , 1 : 81 – 89 .
- Insam , H and Haselwandter , K . 1989 . Metabolic quotient of the soil microflora in relation to plant succession . Oecologia , 79 : 171 – 178 .
- Xu , XK , Inubushi , K and Sakamoto , K . 2006 . Effect of vegetations and temperature on microbial biomass carbon and metabolic quotients of temperate volcanic forest soils . Geoderma , 136 : 310 – 319 .
- Shoji , S , Nanzyo , M and Dahlgren , RA . 1993 . Volcanic Ash Soils: Genesis, Properties, and Utilization , Amsterdam : Elsevier .
- Boudot , JP , Bel HadjBrahim , A , Steiman , R and Seigle-Murandi , F . 1989 . Biodegradation of synthetic organo-metallic complexes of iron and aluminium with selected metal to carbon ratios . Soil BiolBiochem , 21 : 961 – 966 .
- Schwesig , D , Kalbitz , K and Matzner , E . 2003 . Effects of aluminium on the mineralization of dissolved organic carbon derived from forest floors . EurJSoil Sci , 54 : 311 – 322 .
- Anderson , TH and Domsch , KH . 1993 . The metabolic quotient for CO2(qCO2) as a specific activity parameter to assess the effects of environmental conditions, such as pH, on the microbial biomass of forest soils . Soil BiolBiochem , 25 : 393 – 395 .
- Huygens , D , Boeckx , P , Van Cleemput , O , Oyarzun , CE and Godoy , R . 2005 . Aggregate and soil organic carbon dynamics in south Chilean Andisols . Biogeosciences , 2 : 159 – 174 .
- Six , J , Conant , RT , Paul , EA and Paustian , K . 2002 . Stabilization mechanisms of soil organic matter: implications for C-saturation of soils . Plant Soil , 241 : 155 – 176 .
- Aran , D , Gury , M and Jeanroy , E . 2001 . Organo-metallic complexes in an Andosol: a comparative study with a Cambisol and Podzol . Geoderma , 99 : 65 – 79 .
- Odum , EP . 1985 . Trends expected in stressed ecosystems . Bioscience , 35 : 419 – 422 .
- Wardle , DA and Ghani , A . 1995 . A critique of the microbial metabolic quotient (qCO2) as a bioindicator of disturbance and ecosystem development . Soil BiolBiochem , 27 : 1601 – 1610 .
- Odum , EP . 1969 . The strategy of ecosystem development . Science , 164 : 261 – 270 .
- Dilly , O and Munch , JC . 1998 . Ratios between estimates of microbial biomass content and microbial activity in soils . BiolFertilSoils , 27 : 374 – 379 .
- Bardgett , RD and Shine , A . 1999 . Linkages between plant litter diversity, soil microbial biomass and ecosystem function in temperate grasslands . Soil BiolBiochem , 31 : 317 – 321 .
- Liu , Q , Wang , Z and Wang , S . 1992 . Recent volcano eruptions and vegetation history of alpine and sub-alpine of Changbai Mountain . ForEcosystRes , 6 : 57 – 62 . (in Chinese)
- Zhao , DC . 1981 . Preliminary study of the effects of volcano on vegetation development and succession . ForEcosystRes , 2 : 81 – 87 . (in Chinese)
- Cao , M , Zhang , JH , Feng , ZL , Deng , JW and Deng , XB . 1996 . Tree species composition of a seasonal rain forest in Xishuangbanna, Southwest China . TropEcol , 37 : 183 – 192 .
- Davidson , EA , Eckert , RW , Hart , SC and Firestone , MK . 1989 . Direct extraction of microbial biomass nitrogen from forest and grassland soils of California . Soil BiolBiochem , 21 : 773 – 778 .
- Sparling , GP , Feltham , CW , Reynolds , J , West , AW and Singleton , P . 1990 . Estimation of soil microbial C by a fumigation extraction method: Use on soils of high organic matter content, and a reassessment of the kECfactor . Soil BiolBiochem , 22 : 301 – 307 .
- Vance , ED , Brookes , PC and Jenkinson , DS . 1987 . An extraction method for measuring soil microbial biomass C . Soil BiolBiochem , 19 : 703 – 707 .
- Vance , ED and Chapin , FS . 2001 . Substrate limitations to microbial activity in taiga forest floors . Soil BiolBiochem , 33 : 173 – 188 .
- Hu , YL , Wang , SL and Zeng , DH . 2006 . Effects of single Chinese fir and mixed leaf litters on soil chemical, microbial properties and soil enzyme activities . Plant Soil , 282 : 379 – 386 .
- Insam , H . 1990 . Are the soil microbial biomass and basal respiration governed by the climatic regime? . Soil BiolBiochem , 22 : 525 – 532 .
- Lundström , US , van Breemen , N and Bain , DC . 2000 . The podzolization process. A review . Geoderma , 94 : 91 – 107 .
- Nierop , KGJ , Jansen , B and Verstrten , JA . 2002 . Dissolved organic matter, aluminium and iron interactions: precipitation induced by metal/carbon ratio, pH and competition . SciTotal Environ , 300 : 201 – 211 .
- Zysset , M and Berggren , D . 2001 . Retention and release of dissolved organic matter in Podzol B horizons . EurJSoil Sci , 52 : 409 – 421 .
- Priha , O and Smolander , A . 1997 . Microbial biomass and activity in soil and litter under Pinus sylvestris, Picea abies and Betula pendula at originally similar field afforestation sites . BiolFertilSoils , 24 : 45 – 51 .
- Valsecchi , G , Gigliotti , C and Farini , A . 1995 . Microbial biomass, activity, and organic matter accumulation in soils contaminated with heavy metals . BiolFertilSoils , 20 : 253 – 259 .
- Li , YQ , Xu , M , Zou , XM and Xia , Y . 2005 . Soil CO2efflux and fungal and bacterial biomass in a plantation and a secondary forest in wet tropics in Puerto Rico . Plant Soil , 268 : 151 – 160 .
- Neill , C and Gignoux , J . 2006 . Soil organic matter decomposition driven by microbial growth: A simple model for a complex network of interactions . Soil BiolBiochem , 38 : 803 – 811 .
- Wu , J , Joergensen , KG , Pommerening , B , Chaussod , R and Brookes , PC . 1990 . Measurement of soil microbial biomass C by fumigation-extraction – an automated procedure . Soil Biol. Biochem. , 22 : 1167 – 1169 .