Abstract
To understand the influence of basal application of N fertilizer on nitrification potential and N2O and NO emissions, four soil samples were collected from an upland Andisol field just before (sample 1) and 4 (sample 2), 36 (sample 3) and 72 (sample 4) days after the basal application of N fertilizer during the Chinese cabbage growing season from 12 September to 30 November 2005. The potentials of N2O production and nitrification of the soils were determined using a 15N tracer technique and the soils were incubated for 25 days at 25°C and 60% water-filled pore space (WFPS). The results revealed that as much as 84–97% N2O and almost all NO were produced by nitrification. The 15N2O emission peak occurred approximately 350 h after the beginning of incubation for samples 1 and 2, but just 48 h later in samples 3 and 4. Total 15N2O emission during the 25-day incubation of samples 3 and 4 ranged from 190 to 198 µg N kg−1 soil, which was significantly higher than the 99–108 µg N kg−1 soil recorded in samples 1 and 2. Basal application of N fertilizer did not immediately increase the nitrification potential and the ratio of N2O to N added, but did dramatically increase the nitrification potential and the ratio of N2O to N added as (15NH4)2SO4 36–72 days after the basal N fertilizer was added. In contrast, NO emission was negatively correlated with nitrification potential and total N2O emission. As a result, a trade-off relationship between total NO and N2O emissions was identified. The results indicated that there was a time-lagged induction of the change of N turnover in the soil, which was possibly caused by slow population growth of the nitrifiers and/or a slow shift in the microbial community in the soil.
INTRODUCTION
Atmospheric N2O concentration has been increasing (CitationIntergovernmental Panel on Climate Change 2001). Increases in the atmospheric concentration of N2O contribute not only to global warming, constituting 6% of the anthropogenic greenhouse effect, but directly to the destruction of the stratospheric ozone through NO production (CitationIntergovernmental Panel on Climate Change 2001). Nitric oxide participates in the regulation of the oxidant balance and is oxidized into NO2 in the atmosphere, while redeposition of NO and NO2 as acid rain contributes to acidification and eutrophication of ecosystems (CitationCrutzen 1983; CitationNakahara et al. 2003; CitationVitousek et al. 1997). Agricultural soils are a major source of N2O, contributing approximately 6.3 Tg N2O-N per year or 35% of the global annual emission (CitationKroeze et al. 1999; CitationMosier et al. 1998), and are also considered to be an important source of NO, albeit not as important as fossil fuel combustion sources, accounting for approximately 5.5 Tg NO-N per year (CitationDavidson and Kingerlee 1997). Nitrous oxide is produced in soils as a byproduct or intermediate product of nitrification and denitrification processes (CitationBouwman 1990; CitationMinami 2005), as well as chemo-denitrification (Citationvan Cleemput 1998) and fungal transformations (CitationLaughlin and Stevens 2002). The magnitude of N2O and NO production and subsequent emission is determined by N supply, climatic factors and soil properties; the latter will determine the microbial reaction rate, soil aerobicity and gas diffusivity (CitationAkiyama et al. 2006). Nitric oxide emissions are predominantly associated with nitrification and are favored by soil characteristics that provide good aeration, coarse texture and low soil water contents, whereas N2O production and emission is stimulated by increased soil water content and compaction by denitrification (CitationCai et al. 1998; CitationGranli and Bockman 1994).
In Japan, up to 50% of agricultural fields for consecutive upland crop cultivation are covered with volcanic ash soils or Andisols (CitationClassification Committee of Cultivated Soils 1996). Volcanic ash soils tend to maintain their aerobic conditions because of their high porosity and, thus, as much as 87–92% of the N2O produced is derived from autotrophic nitrification (CitationInubushi et al. 1996; CitationKusa et al. 2006). A number of previous field studies have shown that N2O emissions from supplemental N fertilizer were higher than those from basal N fertilizer (CitationDobbie and Smith 2003; CitationJambert et al. 1997; CitationMeng et al. 2005; CitationWilliams et al. 1999; CitationYan et al. 2001). For example, CitationXiong et al. (2006) did not observe any emission peaks of N2O following the first application of N fertilizer, but did record peaks after the second to fifth applications of N fertilizer during five consecutive vegetable crops. CitationNishimura et al. (2005) also did not report any N2O peaks after basal N fertilization in upland rice field, but did record peaks approximately 10 days after the application of supplemental N fertilizer. In contrast, CitationAkiyama et al. (2000) found that the N2O emission peak following basal N application was higher than that after supplemental fertilizer. To understand the dynamic change in the nitrification potential induced by basal N fertilization and the responses in microbial N2O and NO emissions from agricultural soils to basal and supplemental application of N fertilizer, a laboratory incubation experiment was conducted using Andisol soil samples collected at different times after the basal application of N fertilizer during the Chinese cabbage growth period.
MATERIALS AND METHODS
Field experiment
The experiment was conducted at an experimental field in the National Institute for Agro-Environmental Sciences, Tsukuba, Japan (36°01′N, 140°07′E). The field includes six lysimeter plots, each with a 9 m2 (3 m × 3 m) cross-sectional area and a 1.2 m depth. The soil is a light-colored Andisol (Hapludand; CitationClassification Committee of Cultivated Soils 1996). The bulk density of the topsoil is 0.92 g cm−3. The soil pH in H2O is 5.90. Total organic C and N contents in the topsoil are 31.3 g C kg−1 soil and 2.60 g N kg−1 soil, respectively (CitationAkiyama et al. 2000). All plots were uniformly managed for sorghum cultivation for 2 years before experimentation began.
Prior to the sowing of Chinese cabbages on 12 September 2005, compound fertilizer, including urea (15 g N m−2), phosphate (15 g P2O5 m−2) and potassium (15 g K2O m−2), together with fresh cattle manure (1 kg m−2) containing approximately 7 g N kg−1 were used as basal fertilizer and spread uniformly on the soil and then incorporated into the top soil layer of 0–10 cm using a portable rotary tiller. No supplemental fertilizer was applied during the growing season. The cabbage was harvested on 30 November 2005.
Soil sampling
During the 80-day Chinese cabbage growing period, soil samples were collected for incubation four times on 12 September 2005, just before (hereinafter referred to as sample 1), 16 September, 4 days (sample 2), 18 October, 36 days (sample 3) and 23 November, 72 days (sample 4) after basal fertilization (). At each time, eight soil samples were taken from depths between 0 and 10 cm at different locations in each lysimeter plot and then all samples from the six lysimeter plots were mixed thoroughly to form a composite. The NH+ 4-N and NO− 3-N concentrations in field samples 1, 2, 3 and 4 were 2.13 and 7.57, 245.75 and 52.35, 0.57 and 4.76, and 0.44 and 1.03 mg N kg−1 soil, respectively. This indicates that most of the NO− 3 converted from the NH+ 4 applied was either absorbed by Chinese cabbage and microbes or leached out of the top soil 36 days after the basal application of N fertilizer as a result of the light texture of the soil.
Incubation process
The soil samples collected from the field were rapidly transported to the laboratory for sieving (2 mm). Within
24 h after sampling, each 15 g (on an oven-dried basis) fresh soil sample was weighed and distributed among 72 125-mL plastic bottles, and 50 g was added to nine 500-mL incubation bottles. All bottles were divided into three groups (i.e. 24 for each group). Pure water and (15NH4)2SO4 solution or K15NO3 (1.07 atom% 15N) solution were added to each bottle of group one and two, respectively, using a mini-pipette to adjust the soil to 60% water-filled pore space (WFPS) soil moisture and the concentration of NH+ 4 or NO− 3 to 260 mg N kg−1 (oven-dried weight). Pure water only was added to each bottle in group three to act as a control. All bottles were then covered with aluminum foil (punctured with needle holes to maintain an aerobic atmosphere in the bottles) and were incubated in the dark at 25°C. During the incubation, lost water was replaced every 3 days by mini-pipette. Three replicates of the 24 plastic bottles in each group were removed from the incubator at 12, 48, 108, 180, 276, 372, 492 and 612 h after the beginning of incubation to measure changes in soil NH+ 4 and NO− 3 concentrations by incubating with 10% KCl extraction solution for 30 min. The extracted solutions were filtered and stored in a deep freezer (–18°C) until analysis. The NH+ 4 and NO− 3 concentrations were measured using a colorimetric method and a TRAACS 2000 Continuous-Flow Analyzer (BRAN + LUEBBE, Hamburg, Germany). The 15N enrichment of NH+ 4 and NO− 3 were determined using a diffusion methodology (CitationBrookes et al. 1989) and analysis on a MAT 252 isotope ratio mass spectrometer (Thermo Electron, Bremen, Germany). Changes in soil (15NH4)2SO4-derived NH+ 4 concentration between successive sampling days were defined as the net nitrification rate between the sampling days.Three incubation bottles from each group were used to measure gaseous N2O and NO emissions at the above-mentioned times. For NO measurement, the bottles were allowed to aerate for approximately 30 min prior to being capped with a butyl rubber stopper. The first 5-mL sample was collected using a plastic syringe immediately after capping and analyzed immediately. Two more samples were collected and injected into a Chemiluminescence NOx analyzer (Model 42C, Thermo Environmental Instruments, Franklin, MA, USA) at 3.5 min interval to determine NO concentration. After the third sampling, the bottle was re-opened and left until the air established equilibrium inside the bottle and then re-capped and incubated. Just after and 12 h after closure, 1 mL headspace gas was sampled using an airtight syringe and used for N2O measurement. The N2O concentrations were analyzed using a gas chromatograph equipped with an electron capture detector at 340°C; the main separating column was packed with Porapak Q and the carrier gas was 5% methane in argon (GC-ECD 8A, Shimadzu, Kyoto, Japan). Samples for 15N-N2O measurement were stored in 50-mL gas-tight glass bottles and their isotopic enrichment was determined on a MAT 252 Mass Spectrometer with a PreCon system. The 15N-enriched gas fluxes were calculated from the atom% excess of samples, taking account of the atom% excess 15N of N applied.
Statistical analyses
Statistical analyses were conducted using SPSS. Statistically significant differences in total N2O emission among soils were analyzed using anova and least significant difference (LSD) calculations at a 5% significance level (P = 0.05). Linear regression analysis was used to identify significant correlations between N2O and NO emissions.
RESULTS
Nitrous oxide emission
shows the net nitrification rate and cumulative N2O emission from 15N-labeled fertilizer added to an
Table 1 Net nitrification rates and supplemental fertilizer derived N2O emission from the soils during the first 180 h and 25-day incubation after adding (15NH4)2SO4 or K15NO3 fertilizer and incubating at 25°C and 60% water-filled pore space
Figure 2 (a) Total N2O emission from the soils during the 25-day incubation with and without supplemental N addition. CK represents the treatment without supplemental N addition. (b) Supplemental (15NH4)2SO4-derived N2O emission from the soils during the 25-day incubation. Error bars denote the standard deviation (n = 3).
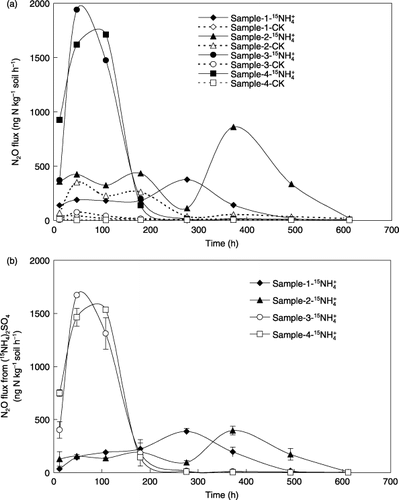
Table 2 Total N2O and NO emissions from the soils with and without supplemental N addition during the 25-day incubation
listed the total N2O and NO emissions determined using a GC-ECD and a chemiluminescence NOx analyzer, respectively, from the incubated soils with and without supplemental N addition. Total N2O emission during the 25-day incubation at 60% WFPS and 25°C was significantly higher in samples 2, 3 and 4 than in sample 1. To exclude the likely influence of basal N fertilizer and mineralized N from soil organic matter on N2O emission, the results for total N2O emission (, ) were compared with those for 15N2O emission (, ,). The pattern of cumulative 15N2O emissions during incubation is illustrated in . Cumulative 15N2O emissions during the 25-day incubation at 60% WFPS and 25°C in samples 3 and 4 were 190 and 198 µg N kg−1 soil, respectively, which were significantly higher than the 99.3 µg N kg−1 soil recorded in sample 1 and the 108 µg N kg−1 soil recorded in sample 2 (). In addition, 25–31% of the cumulative 15N2O emission was released in the first 180 h of incubation in samples 1 and 2, whereas as much as 94–96% was released for samples 3 and 4. In total, the emission factor of N2O, the ratio of emitted 15N2O-N to applied 15NH+ 4-N, was 0.072–0.075% in samples 3 and 4, which was nearly twice the amount in samples 1 and 2 (0.038–0.041%).
Nitric oxide emission and the ratio of NO to N2O
Similar to N2O, NO emission was found only in NH+ 4-amended soil and was under the detection limit in NO− 3-amended soil (). For samples 1 and 2, large NO emissions occurred throughout the experimental period until the end of incubation. In contrast, almost all NO was produced and emitted within the first 180 h of incubation in samples 3 and 4. Regression analysis showed that NO emission during the incubation period was significantly correlated with N2O emission in all soils except sample 2 ().
Total NO emissions in samples 1 and 2 were 8540 and 8525 µg N kg−1 soil, respectively, significantly higher than the emissions recorded in samples 3 and 4 (). However, NO emission from control sample 2, which was amended without (15NH4)2SO4, amounted to 6167 µg N kg−1 soil, resulting in the net NO emission from 15NH+ 4 added to sample 2 to be only 2358 µg N kg−1 soil. The large emission of NO from sample 2 may be attributed to the presence of a large amount of urea that was applied as basal fertilizer.
The ratios of NO to N2O emitted in samples 1 and 2 were 81 and 38, respectively, and were significantly larger than those emitted in samples 3 and 4 (). While,
Figure 3 Change in cumulative (15NH4)2SO4-derived N2O emission from the soils during the 25-day incubation after adding supplemental (15NH4)2SO4. Error bars denote the standard deviation (n = 3).
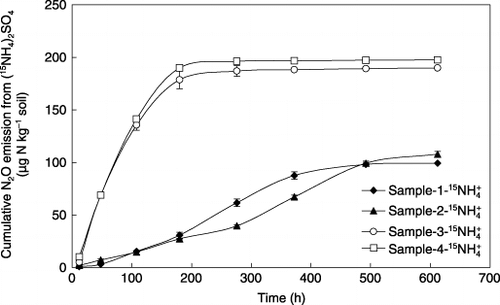
Figure 4 Nitric oxide emission from the soils during the 25-day incubation after adding supplemental (15NH4)2SO4. Error bars denote the standard deviation (n = 3).
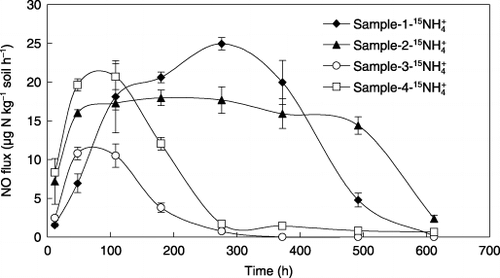
Variation in NH+ 4 and NO− 3 concentration
After (15NH4)2SO4 was added to soils, rapid decreases in 15NH4-N and increases in 15NO3-N concentrations occurred in samples 3 and 4 () and as much as 81–96% of the 15NH+ 4 added was nitrified to 15NO− 3 within the first 180 h of incubation (). In contrast, the magnitude of NH+ 4 added decreased more slowly, but was completely consumed, during the 25-day incubation in samples 1 and 2, resulting in only 33–35% of the 15NH+ 4 added being consumed in the first 180 h.
The changes in 15NO3-N concentration during the 25-day incubation could be fitted to two types of models. Samples 1 and 2 followed a zero-order reaction model, whereas samples 3 and 4 followed a first-order reaction model (). However, changes in 15NO3-N concentration in samples 3 and 4 also followed a zero-order model within the first 180 h of incubation, during this period nearly all of the 15NH4-N was oxidized
Table 3 Reaction models and best-fit parameters obtained from the rates of nitrification after adding supplemental (15NH4)2SO4 to Andisols and incubating at 25°C and 60% water-filled pore space
DISCUSSION
Effect of basal N fertilizer on nitrification potential and N2O emission
Many studies examining the environmental factors affecting N2O and NO emissions from cultivated soils have been undertaken. Soil temperature, moisture regime and fertilizer management are considered to be the major factors influencing emissions of nitrogen oxides (CitationDavidson 1993; CitationGranli and Bockman 1994). Studies have shown that a WFPS of 60% is the optimum moisture for nitrification (CitationGranli and Bockman 1994). In the present study, we designed similar environmental conditions (60% WFPS moisture and 25°C) in the laboratory to compare soils sampled at different times after basal application of N fertilizer, to evaluate how the application of basal N fertilizer influences the nitrification potential, and N2O and NO emissions from supplemental N fertilizer in an Andisol. In general, NO and N2O are produced in soils by nitrification and denitrification synchronically; however, the emission ratio of NO/N2O was relatively constant during nitrification (CitationDavidson 1993). CitationInubushi et al. (1996) reported that 87–90% of N2O produced was derived from autotrophic nitrification during the incubation of an Andisol at 60 and 80% water-holding capacity with NH+ 4 added for 32 days at 30°C. Our results showed that N2O emission in NO− 3-amended soil was equal to 3–16% of that in NH+ 4-amended soil, even though fresh cattle manure was applied and no NO was released during the 25-day incubation at 60% WFPS and 25°C, indicating that N2O and NO were predominantly produced by nitrification ().
Total N2O emission during the 25-day incubation in samples 1 and 2 was similar, but only half of that in samples 3 and 4. For samples 3 and 4, as much as 94–96% of the cumulative 15N2O emission from (15NH4)2SO4 was released within 180 h of incubation; of course 81–96% of NH+ 4 added was oxidized. In contrast, only 25–31% of the total N2O emission occurred in the first 180 h in samples 1 and 2 when 33–35% of the NH+ 4 added disappeared. This indicates that nitrifiers in samples 3 and 4 not only responded more rapidly to the NH+ 4 added, but also converted NH+ 4 into N2O more efficiently. There was a significant temporal variation in nitrification rate constants, which sharply increased from 0.378–0.456 in samples 1 and 2 to 1.144–1.276 in samples 3 and 4 (), indicating that the application of basal N-amended fertilizer significantly changed the soil nitrification potential (CitationFlowers and O’Callaghan 1983; CitationWheatley et al. 2001).
Starved ammonia oxidizers exhibit a lag phase of up to 150 h, which would be of considerable significance and probable disadvantage (CitationBatchelor et al. 1997). As competition will be intense for the limited supply of N, organisms need to respond rapidly to gain a competitive advantage, while also investing the minimum energy. Ammonia oxidizers are poor competitors for NH+ 4 compared to heterotrophic bacteria (Citationvan Niel et al. 1993). This is because ammonia oxidizers use CO2 as a sole source of C and the energy for the CO2 fixation originates from nitrification (CitationAleem et al. 1965). NH3 and NO− 2 are not very effective energy sources and as much as 30 g NH3 is needed for the build-up of 1 g dry mass of Nitrosomonas sp. (CitationSchlegel 1992). Therefore, the active growth of ammonia oxidizers was very slow (CitationNishio and Fujimoto 1990; CitationSchmidt 1982; CitationWheatley et al. 2001). The population of ammonia oxidizers did not change within 13 days, but increased from 8.9 × 106 to 38 × 106 cells g−1 soil after 39 days of NH+ 4 applied to a tomato field (CitationOkano et al. 2004). The calculated generation time for ammonia oxidizers in the field is as long as 372.7 h (CitationOkano et al. 2004). Furthermore, CitationMendum et al. (1999) and CitationAvrahami et al. (2003) found that there was not a community shift in ammonia oxidizers during 4–6 weeks of incubation, but there was after 16 weeks of incubation, although the ammonia oxidizer population did not exhibit net growth. The application of N fertilizer could gradually stimulate the growth of Nitrosospira clusters 1 and 3 and Nitrosomonas cluster 7, which have high nitrification potentials with high ratios of N2O to N added and become dominant in lieu of Nitrosospira clusters 2, 4 and 9 (CitationAvrahami et al. 2003; CitationBruns et al. 1999; CitationKowalchuk et al. 2000; CitationWebster et al. 2002).
CitationWheatley et al. (2001) found that there was an apparent maximum level of nitrification potential in a UK clay loam, which may be related to the biomass of nitrifiers, and N addition did not stimulate nitrifier growth in soils that had already attained the maximum nitrification potential. CitationOkano et al. (2004) observed a similar phenomenon in which the population growth of ammonia oxidizers had ceased by the time most of the added NH+ 4 had been depleted in the case of 1.5 mmol L−1 (NH4)2SO4 treatment. In contrast, growth reached a plateau at approximately 6.0 × 107 cells g−1 soil, although there was still a high NH+ 4 concentration remaining in the case of 7.5 mmol L−1 (NH4)2SO4 treatment. Apparently, the stimulation effect of N fertilizer on population growth and nitrification activity of nitrifiers mainly depends on whether the soil attains the maximum nitrification potential. In the present study, it is unlikely that the tested soil reached the maximum nitrification potential before or just after basal application of N fertilizer, probably because of the size of the indigenous nitrifier population. Our study indicated that basal application of N fertilizer was unable to stimulate the population growth of nitrifiers immediately, which resulted in a relatively long response time for nitrification and decreased the ratio of N2O to N added as basal fertilizer. However, the basal application of N fertilizer did reduce the response time and dramatically increase the ratio of N2O to N added as supplemental fertilizer at 36 days or more after basal fertilization. This is likely to be because of the increased population of nitrifiers and/or a shift in the community of ammonia oxidizers from a low to high ratio of N2O to N added.
Effect of basal fertilizer on NO emission
In the present study, NO emission was related significantly to N2O emission during the incubation period in all soils except sample 2, indicating that N2O and NO were simultaneously produced (). However, total NO emission during the 25-day incubation was negatively correlated with total N2O emission (, R = −0.94, n = 3). In other words, increased nitrification potential in samples 3 and 4 enhanced N2O emission, but reduced NO emission. This finding is strongly supported by the results of previous studies. CitationCheng et al. (2004) reported that the ratio of emitted N2O relative to net nitrification rate in acid soils was lower than that in alkaline soils, but the ratio of emitted NO to net nitrification rate was significantly higher in acid soils. CitationHou et al. (2000) found that the same tested soil amended with urea released more N2O but less NO at 70% than at 40% WFPS. Our finding further confirmed that there was a trade-off relationship between total NO and N2O emissions and the ratio of NO/N2O decreased with increased nitrification potential in the tested Andisol (CitationWolf and Russow 2000).
CitationYamulki et al. (1997) reported that NO, but not N2O, was emitted from sterile acid soils, indicating that NO could also be produced by chemodenitrification. Gross NO production rate by chemodenitrification was not correlated with the amounts of NH+ 4 and NO− 3 or with the rate of the overall nitrification, but was correlated with the concentration of HNO2, which originated from autotrophic microbial oxidation of NH+ 4 to NO− 2 (CitationVenterea and Rolston 2000). The NO was produced by chemodenitrification through the aqueous decomposition of HNO2 and reactions of HNO2 with phenolic and other functional constituents of soil organic matter or with reduced metal cations (Citationvan Cleemput and Baert 1976; CitationNelson 1982; CitationStevenson 1994). CitationMorrill and Dawson (1967) found that Nitrobacter populations could have experienced slightly greater lag effects than ammonia oxidizers, resulting in NO− 2 accumulation, which in turn provided the opportunity for abiotic HNO2 and NO production prior to utilization by oxidative or reductive microbes. In the present study, although no NO− 2 was measured in soil, we could not exclude the contribution of chemodenitrification to NO production.
In contrast, extracellular NO is an oxidative intermediate in autotrophic oxidation of NH+ 4 to NO− 2, although this has not been confirmed (CitationHooper 1982). In addition, NO is not necessarily a precursor for the formation of N2O under nitrification (CitationWolf and Russow 2000). Therefore, it is possible that NO just “leaks” from the oxidization process of NH+ 4. There is evidence that Nitrosomonas and Nitrobacter populations preferentially grow on the oppositely charged surfaces in soils, each tending to proliferate on the surfaces to which its primary substrates are absorbed (CitationUnderhill and Porsser 1987). The growth rate of nitrite oxidizers was faster than that of ammonia oxidizers after N addition (CitationDe Boer et al. 1989), which in turn accelerated the oxidation of NO− 2 and was also conducive to the oxidation of NH+ 4. Microbial NO− 2 utilization occurring simultaneously with abiotic HNO2 reduction could have competed for available NO− 2 and thereby attenuated abiotic NO production (CitationVenterea and Rolston 2000). Furthermore, an increased oxidation rate of NH+ 4 to NO− 2 and, in particular, of NO− 2 to NO− 3 induced by N addition possibly reduced “leaking” of NO from the atuotrophic oxidation of NH+ 4. The third possibility is that the dominant ammonia oxidizers induced by N addition in samples 3 and 4 differed greatly from those in samples 1 and 2, and may have a high nitrification potential with a high ratio of N2O produced to N added and a low ratio of NO produced to N added. Further study is needed to evaluate the contribution of chemodenitrification to NO production and to determine whether there is a community shift in ammonia oxidizers among these four soils.
CONCLUSION
An incubation experiment of a cultivated Andisol collected at different times before and after basal fertilization indicated that there was a time lagged induction in N2O emissions, which was possibly caused by slow population growth of nitrifiers and/or a slow shift in the microbial community in the soil. The change in N turnover in the soil dramatically increased the ratio of N2O to N added as supplemental fertilizer at 36 days or more after basal fertilization. In addition, slow oxidization of NH+ 4 to NO− 2 and/or NO− 2 to NO− 3 in the soil during the lag-time resulted in relatively large NO emissions. In contrast, accelerated oxidation by increased nitrification potential increased N2O emission, but reduced NO emission after the lag time, which in turn showed a trade-off relationship between total NO and N2O emissions.
ACKNOWLEDGMENTS
This study was funded by the Japan Society for the Promotion of Science (JSPS) and partly by the Global Environment Research Fund S2_3a. We also thank the National Basic Research Program of China (2005CB121101) and the National Natural Science Foundation of China (40621001) for their financial support.
REFERENCES
- Akiyama , H , Tsuruta , H and Watanabe , T . 2000 . N2O and NO emissions from soils after the application of different chemical fertilizers . Chemosphere-Global Change Sci , 2 : 313 – 320 .
- Akiyama , H , Yan , XY and Yagi , K . 2006 . Estimations of emission factors for fertilizer-induced direct N2O emissions from agricultural soils in Japan: Summary of available data . Soil Sci. Plant Nutr. , 52 : 774 – 787 .
- Aleem , MIH , Hoch , GE and Varner , JE . 1965 . Water as the source of oxidizing and reducing power in bacteria chemosynthesis . Proc. Natl Acad. Sci. USA , 54 : 869 – 873 .
- Avrahami , S , Liesack , W and Conrad , R . 2003 . Effects of temperature and fertilizer on activity and community structure of soil ammonia oxidizers . Environ. Microbiol , 5 : 691 – 705 .
- Batchelor , SE , Cooper , M Chrabra , SR . 1997 . Cell density-regulated recovery of starved biofilm populations of ammonia-oxidising bacteria . Appl. Environ. Microbiol , 63 : 2281 – 2286 .
- Bouwman , AF . 1990 . “ Exchange of greenhouse gases between terrestrial ecosystems and the atmosphere ” . In Soils and the Greenhouse Effect , Edited by: Bouwman , AF . 61 – 127 . Chichester : John Wiley & Sons .
- Brookes , PD , Stark , JM and McInteer , BB . 1989 . Diffusion method to prepare soil extracts for automated nitrogen-15 analysis . Soil Sci. Soc. Am. J , 53 : 1707 – 1711 .
- Bruns , MA , Stephen , JR , Kowalchuk , GA , Prosser , JI and Paul , EA . 1999 . Comparative diversity of ammonia oxidizer 16S rRNA gene sequences in native, tilled and successional soils . Appl. Environ. Microbiol , 65 : 2994 – 3000 .
- Cai , GX , Fan , XH , Yang , Z and Zhu , ZL . 1998 . Gaseous loss of nitrogen from fertilizers applied to wheat on a calcareous soil in North China Plain . Pedosphere , 8 : 45 – 52 .
- Cheng , WG , Tsuruta , H , Chen , GX and Yagi , K . 2004 . N2O and NO production in various Chinese agriculture soils by nitrification . Soil Biol. Biochem , 36 : 953 – 963 .
- Classification Committee of Cultivated Soils . 1996 . “ Andosols ” . In Classification of Cultivated Soils in Japan: Third Approximation. , 9 – 10 . Tsukuba, , Japan : National Institute for Agro-Environmental Sciences .
- Crutzen , PJ . 1983 . “ Atmospheric interactions – homogeneous gas reactions of C, N and S containing compounds ” . In The Major Biogeochemical Cycles and their Interactions , Edited by: Bolin , B and Cook , RB . 67 – 112 . New York : Wiley .
- Davidson , EA . 1993 . “ Soil water content and the ratio of nitrous oxide and nitric oxide emitted from soil ” . In Biogeochemistry of Global Change: Radioactively Active Trace Gases , Edited by: Oremland , RS . 369 – 386 . New York : Chapman & Hall .
- Davidson , EA and Kingerlee , W . 1997 . A global inventory of nitric oxide emissions from soils . Nutr. Cycl. Agroecosys , 48 : 37 – 50 .
- De Boer , W , Duyts , H and Laanbroek , HJ . 1989 . Urea stimulated autotrophic nitrification in suspensions of fertilized acid heart soil . Soil Biol. Biochem , 21 : 349 – 354 .
- Dobbie , KE and Smith , KA . 2003 . Impact of different forms of N fertilizer on N2O emissions from intensive grassland . Nutr. Cycl. Agroecosys , 67 : 37 – 46 .
- Flowers , TH and O’Callaghan , JR . 1983 . Nitrification in soils incubated with pig slurry or ammonium sulphate . Soil Biol. Biochem , 15 : 337 – 342 .
- Granli , T and Bøckman , OC . 1994 . Nitrous oxide from agriculture . Norwegian J. Agr. Sci , 12 ( Suppl. ) : 1 – 128 .
- Hooper , A . 1982 . “ Ammonia oxidation and energy transduction in the nitrifying bacteria ” . In Microbial Chemoautotrophy , Edited by: Strohl , WR and Tuvinen , OH . 133 – 167 . Columbus, OH : Ohio State University Press .
- Hou , A , Akiyama , H , Nakajima , Y , Sudo , S and Tsuruta , H . 2000 . Effects of urea form and soil moisture on N2O and NO emissions from Japanese Andosols . Chemosphere-Global Change Sci , 2 : 321 – 327 .
- Inubushi , K , Naganuma , H and Kitahara , S . 1996 . Contribution of denitrification and autotrophic and heterotrophic nitrification to nitrous oxide production in andosols . Biol. Fertil. Soils , 23 : 292 – 298 .
- Intergovernmental Panel on Climate Change . 2001 . Climate change 2001: The Scientific Basis , Cambridge : Cambridge University Press .
- Jambert , C , Serca , D and Delmas , R . 1997 . Quantification of N-losses as NH3, NO and N2O and N2from fertilized maize fields in southwestern France . Nutr. Cycl. Agroecosys , 48 : 91 – 104 .
- Kowalchuk , GA , Stienstra , AW , Heilig , GH , Stenphen , JR and Woldendrop , JW . 2000 . Changes in the community structure of ammonia-oxidizing bacteria during secondary succession of calcareous grasslands . Environ. Microbiol , 2 : 99 – 110 .
- Kroeze , CA , Mosier , AR and Bouwman , L . 1999 . Closing the global N2O budget: A retrospective analysis 1500–1994 . Global Biogeochem. Cycl , 13 : 1 – 8 .
- Kusa , K , Hu , R , Sawamoto , T and Hatano , R . 2006 . Three years of nitrous oxide and nitric oxide emissions from silandic andosols cultivated with maize in Hokkaido, Japan . Soil Sci. Plant Nutr , 52 : 103 – 113 .
- Laughlin , RJ and Stevens , RJ . 2002 . Evidence for fungal dominance of denitrification and codenitrification in a grass soil . Soil Sci. Soc. Am. J , 66 : 1540 – 1548 .
- Mendum , TA , Sockett , RE and Hirsch , PR . 1999 . Use of molecular and isotopic techniques to monitor the response of autotrophic ammonia-oxidizing populations of the β subdivision of the class Protecobacteria in arable soils to nitrogen fertilizer . Appl. Environ. Microbiol , 65 : 4155 – 4162 .
- Meng , L , Ding , WX and Cai , ZC . 2005 . Long-term application of organic manure and nitrogen fertilizer on N2O emission, soil quality and crop productivity in a sandy loam soil . Soil Biol. Biochem , 37 : 2037 – 2045 .
- Minami , K . 2005 . N cycle, N flow trends in Japan, and strategies for reducing N2O emission and NO3 pollution . Pedosphere , 15 : 164 – 172 .
- Morrill , L and Dawson , J . 1967 . Patterns observed for the oxidation of ammonium to nitrate by soil organisms . Soil Sci. Soc. Am. J , 31 : 757 – 760 .
- Mosier , A , Kroeze , C , Nevison , C , Oenema , O , Seitzinger , S and Van Cleemput , O . 1998 . Closing the global N2O budget: Nitrous oxide emissions through the agricultural nitrogen cycle . Nutr. Cycl. Agroecosys , 52 : 225 – 248 .
- Nakahara , O , Yamagami , T , Koide , T , Sakai , K and Hatano , R . 2003 . Spatial variation in nitrogen deposition over five adjacent catchments in a larch forest . Soil Sci. Plant Nutr , 49 : 741 – 746 .
- Nelson , D . 1982 . “ Gaseous losses of nitrogen other than through denitrification ” . In Nitrogen in Agricultural Soils , Edited by: Stevenson , F . 327 – 364 . Madison : American Society of Agronomy .
- Nishimura , S , Sawamoto , T , Akiyama , H , Sudo , S , Cheng , W and Yagi , K . 2005 . Continuous, automated nitrous oxide measurements from paddy soils converted to uplands . Soil Sci. Soc. Am. J , 69 : 1977 – 1986 .
- Nishio , T and Fujimoto , T . 1990 . Kinetics of nitrification of various amounts of ammonium added to soils . Soil Biol. Biochem , 22 : 51 – 55 .
- Okano , Y , Hristova , KR Leutenegger , CM . 2004 . Application of real-time PCR to study effects of ammonium on population size of ammonia-oxidizing bacteria in soil . Appl. Environ. Microbiol , 70 : 1008 – 1016 .
- Schlegel , HG . 1992 . Allgemeine Mikrobiologe , Stuttgart : Thieme Verlag .
- Schmidt , EL . 1982 . Nitrification in soil . Agron , 22 : 253 – 288 .
- Stevenson , FJ . 1994 . Humus Chemistry: Genesis, Composition, Reaction , New York : John Wiley .
- Underhill , SE and Porsser , JI . 1987 . Surface attachment of nitrifying bacteria and their inhibition by potassium ethyl xanthate . Microbial Ecol , 14 : 129 – 139 .
- van Cleemput , O . 1998 . Subsoils: chemo- and biological denitrification, N2O and N2emissions . Nutr. Cycl. Agroecosys , 52 : 187 – 194 .
- van Cleemput , O and Baert , L . 1976 . Theoretical consideration on nitrite self-decomposition reactions in soils . Soil Sci. Soc. Am. J , 40 : 322 – 324 .
- van Niel , EW , Arts , PAM , Wesselink , BJ , Robertson , LA and Kuene , JG . 1993 . Competition between heterotrophic and autotrophic nitrifiers for ammonia in chemostat cultures . FEMS Microbiol. Ecol , 102 : 109 – 118 .
- Venterea , RT and Rolston , DE . 2000 . Mechanisms and kinetics of nitric and nitrous oxide production during nitrification in agricultural soil . Global Change Biol , 6 : 303 – 316 .
- Vitousek , PM , Aber , JD Howarth , RW . 1997 . Human alteration of the global nitrogen cycle: Sources and consequences . Ecol. Appl , 7 : 737 – 750 .
- Webster , G , Embley , TM and Prosser , JI . 2002 . Grassland management regimens reduce small-scale heterogeneity and species diversity of β-proteobacterial ammonia oxidizer populations . Appl. Environ. Microbiol , 68 : 20 – 30 .
- Wheatley , RE , Ritz , K , Crabb , D and Caul , S . 2001 . Temporal variations in potential nitrification dynamics in soil related to differences in rates and types of carbon and nitrogen inputs . Soil Biol. Biochem , 33 : 2135 – 2144 .
- Williams , DL , Ineson , P and Coward , PA . 1999 . Temporal variation in nitrous oxides fluxes from urine-affected grassland . Soil Biol. Biochem , 31 : 779 – 788 .
- Wolf , I and Russow , R . 2000 . Different pathways of formation of N2O, N2and NO in black earth soil . Soil Biol. Biochem , 32 : 229 – 239 .
- Xiong , ZQ , Xie , YX , Xing , GX , Zhu , ZL and Butenhoff , C . 2006 . Measurements of nitrous oxide emissions from vegetable production in China . Atmos. Environ , 40 : 2225 – 2234 .
- Yamulki , S , Harrison , RM , Goulding , KWT and Webster , CP . 1997 . N2O, NO and NO2fluxes from a grassland: effect of soil pH . Soil Biol. Biochem , 29 : 1199 – 1208 .
- Yan , XY , Hosen , Y and Yagi , K . 2001 . Nitrous oxide and nitric oxide emissions from maize field plots as affected by N fertilizer type and application method . Biol. Fertil. Soils , 34 : 297 – 303 .