Abstract
Pedogenetic acidification processes in forest soils derived from sedimentary rocks under mesic and thermic soil temperature regimes (MSTR and TSTR; corresponding to mean annual soil temperatures of 8–15°C and 15–22°C, respectively) in the Kinki District were investigated based on titratable alkalinity and acidity characteristics and soil solution composition. According to statistical analyses of the soil properties, the titratable alkalinity required to acidify soils to pH 3.0 was considered to be derived from reactions occurring at the surface of amorphous Al oxides, while titratable acidity at a pH ranging from 5.5 to 8.3 results from dissociation of acidic functional groups of soil humus and/or deprotonation of oxide surfaces. These reactions were generally more prevalent in MSTR soils. Based on the soil solution composition and titratable alkalinity and acidity in the soil profiles, two processes were postulated for pedogenetic acidification, that is, eluvi-illuviation of inorganic Al followed by subsequent adsorption of dissolved organic carbon (DOC) onto the precipitates of Al hydroxides and comigration of Al and DOC in the form of organo-mineral complexes. Both processes were conspicuous in MSTR soils and significantly contributed to soil organic matter storage in the subsoil layers. Pedogenetic acidification in forest soils with MSTR was characterized by an accumulation of acidity in the form of amorphous compounds and/or organo-mineral complexes in the B horizon. It seems, to some extent, similar to podzol formation, at least in terms of Al translocation. Amorphous Al hydroxides protect against further acidification through protonation and/or partial monomerization and can, thus, be regarded as a temporary storage of acid neutralizing capacity of the soil, which would be otherwise leached out directly from the soil profile. In contrast, the acid-buffering reactions of TSTR soils seemed to occur, if at all, mostly at or near the soil surface and the contribution of the B-horizon soils was limited.
INTRODUCTION
A large part of Japan is situated in the humid temperate climatic zone with high precipitation, often exceeding 1,500 mm year−1. Because of the acid load derived both from ecosystem external (i.e. acid deposition) and internal processes, such as excess cation uptake by plants, N transformation and dissociation of organic or carbonic acid (CitationHelyer and Porter 1989; CitationJohnson et al. 1992; Citationvan Breemen et al. 1983, Citation1984), forest soils in Japan are usually acidic. In the pedogenesis of acidic forest soils, Al is considered to play an important role through, for example, dissolution from primary minerals, hydrolysis and formation of Al hydroxides, formation of organo-mineral complexes and translocation through the soil profile (e.g. CitationDriscoll 1989). Among them, the Al hydroxides can work as one of the major components of the acid neutralizing capacity (ANC) of soils through direct dissolution as well as indirectly through anion adsorption (CitationDavis and Hem 1989; CitationFunakawa et al. 1993b; CitationParfitt 1978). CitationFunakawa et al. (1993b) described the soil profiles from non-volcanic origin in the cool temperate zone forests of northern Kyoto as being a sequence of different stages of soil acidification with lower horizons representing earlier stages. The first stage is characterized by the accumulation of amorphous Al compounds as a result of Al illuviation (weak podzolization), and is typically observed in the B horizons; successive monomerization of these amorphous Al hydroxides because of an increasing acid load forms the second stage; and a clear decrease in amorphous Al content, weakened structure and consequent eluviation of Fe under reducing conditions comprises the third stage.
Sesquioxides also play an important role in soil taxonomy. CitationHirai et al. (1991) attempted to classify Japanese forest soils into four groups based mainly on the characteristics of sesquioxides in the B horizon, that is, soils strongly affected by volcanic ash and characterized by the formation of allophane, soils with a relatively large amount of amorphous oxides in the cool temperate climatic zone (corresponding to a mesic soil temperature regime [MSTR] with a mean annual soil temperature of 8–15°C), soils characterized by lower contents of amorphous compounds in the warm temperate climatic zone (corresponding to a thermic soil temperature regime [TSTR] with a mean annual soil temperature of 15–22°C), and soils with very small amounts of amorphous oxides in the subtropical climatic zone (corresponding to a hyperthermic soil temperature regime). According to the Unified Soil Classification System of Japan, 2nd Approximation (CitationFourth Committee for Soil Classification and Nomenclature 2002), the four soils as classified by CitationHirai et al. (1991) are approximately equivalent to Brown Kuroboku soils, Haplic Brown Forest soils, Haplic or Yellow Brown Forest soils and Argic or Cambic Red-yellow soils, respectively, based mainly on the organic C contents in the B-horizon soils because there is a clear correlation between the amounts of total C and the amorphous compounds (acid ammonium oxalate-extractable Al plus Fe). The process of pedogenetic acidification described by CitationFunakawa et al. (1993b), which emphasized the formation and subsequent dissolution of amorphous Al compounds during podzolization, was observed in soils in the second group of Hirai's classification or in the Haplic Brown Forest soils of the Unified Soil Classification System of Japan, that is, forest soils typically derived from sedimentary rocks in the cool temperate climatic zone. As the sesquioxidic properties of the B-horizon soils are variable according to the soil temperature regimes, it is worthwhile to compare corresponding pedogenetic processes among soils under different bioclimatic conditions. In the present study, we compared the pedogenetic acidification processes in forest soils under both cool and warm temperate climatic conditions (focusing on soil solution composition dynamics and soil acidity/alkalinity of soils) to assess the validity of the proposed soil classification systems from a pedogenetic point of view, as well as to further understand the long-term processes of soil organic matter (SOM) accumulation and soil acidification.
MATERIALS AND METHODS
Study sites
A total of 12 sites were selected from the eastern slope of Mt Odaigahara (1,695 m in altitude) in southern Mie Prefecture, Japan (). Elevation ranged from 110 to 1,600 m and all the soils were located on upper convex slopes. Three profiles (MD1, MD2 and FD) were situated in the warm temperate zone with TSTR, in which evergreen species such as Quercus glauca and Castanopsis cuspidata dominated, and three (TT, OT and OM) were situated in the cool temperate zone with MSTR. Among the latter, TT and OT were covered by deciduous broad-leaved forest dominated by Fagus crenata and Quercus crispula, whereas OM was situated under a forest of Picea jezoensis. The remaining six profiles (OF, ST1, ST2, MT, KD1 and KD2) were considered to be at the border of both zones and the vegetation covers were composed of mixtures of deciduous and evergreen species. The annual precipitation in the area is between 4,700 mm (Owase city) and approximately 6,000 mm (Mt Odaigahara). The average air temperature is 15.6°C in Owase city (15 m in altitude). Mesozoic sedimentary rocks, such as sandstone, mudstone and chert, cover a large part of the area. The influence of volcanic ejecta could be small. A brief description of each soil profile is given in . Most of the soils are classified as Dystrudepts using the US Department of Agriculture soil classification system (CitationSoil Survey Staff 2006), or as Haplic Brown Forest soils or Non-Allophanic Kuroboku soils using the Unified Soil Classification System of Japan, 2nd Approximation (CitationFourth Committee for Soil Classification and Nomenclature 2002).
Physicochemical and mineralogical properties of the soils
Soil samples were collected from each pedogenetic horizon of the soil profiles and then air-dried and passed through a 2-mm mesh sieve for the following chemical and mineralogical analyses. Soil pH in water or 1 mol L−1 KCl solution was measured with a glass electrode using a soil : solution ratio of 1:5. Cation exchange capacity (CEC) and the content of exchangeable bases were measured after extracting with 1 mol L−1 NH4OAc at pH 7.0 and then with a 10% NaCl solution (CitationThomas 1982). The NH+ 4 extracted with 10% NaCl solution was distilled after the addition of concentrated NaOH solution and collected into 2% H3BO4 solution, followed by determination of NH4 with HCl titration (0.01 mol L−1). The contents of exchangeable bases (Na, K, Mg and Ca) in NH4OAc solution were determined by atomic absorption spectrophotometry (AAS) (AA-840-01; Shimadzu, Kyoto, Japan). Exchangeable Al and H were extracted with 1 mol L−1 KCl. The exchange acidity (Al + H) was determined by titration with 0.01 mol L−1 NaOH at pH 8.3 using phenolphthalein as an indicator and then, after the addition of 4% NaF solution to liberate OH− from Al(OH)3 precipitates, the exchangeable Al was determined by back titration to the same pH (8.3) with 0.01 mol L−1 HCl. The content of exchangeable H was determined as the difference. Total C and N contents were measured with a NC analyzer (Sumigraph NC-800; Sumika Chemical Analytical Service, Tokyo, Japan). Free oxides (Fe, Al and Si) were extracted using three different methods: (1) extraction in the dark with acid (pH 3) 0.2 mol L−1 ammonium oxalate (CitationMcKeague and Day 1966) – Feo, Alo, Sio; (2) extraction with a citrate-bicarbonate mixed solution buffered at pH 7.3 with the addition of sodium dithionite (DCB) at 80°C (CitationMehra and Jackson 1960) – Fed, Ald, Sid; (3) extraction with 0.1 mol L−1 pyrophosphate at pH 10 (CitationSchuppli et al. 1983) – Fep, Alp, Sip. The contents of Fe, Al and Si in each extract were determined by multi-channel inductively coupled argon plasma atomic emission spectroscopy (ICP-AES) (SPS-1500; Seiko Instruments, Tokyo, Japan) after filtration of the extracts by 0.45 (for [1] and [2]) or 0.025 µm (for [3]) Millipore filters (Tokyo, Japan). Carbon content in the 0.1 mol L−1 pyrophosphate extracts was determined using the wet combustion method (CitationTinsley 1947). Particle size distribution was determined using the pipette method. Clay minerals were identified by X-ray diffraction (XRD) analysis using Cu–Kα radiation (X-ray diffractometer, RAD-2RS; Rigaku, Tokyo, Japan).
Table 1 Brief description of the soils studied
The titration of the soils was conducted as follows: 4.0 g of the soil samples was suspended in 40 mL of 0.10 mol L−1 NaCl solution as the supporting electrolyte. After pre-equilibration for 2 min, the suspension was titrated, while stirring, with 0.10 mol L−1 HCl or NaOH using a potentiometric automatic titrator (HSS/HTS-10A; Toa Denpa Kogyo, Kyoto, Japan), keeping the titration rate at 0.07 mL min−1. Titratable acidity was determined as OH− consumption at pH 8.3, and titratable alkalinity as H+ consumption at pH 3.0 (CitationFunakawa et al. 1993b; CitationKinniburgh 1986).
Soil solution composition
Soil solution was collected from two selected layers of soils at different elevations, that is, MD1, MD2, KD1, KD2, TT and OT profiles, using the porous cup method with a hand-operated vacuum pump during the period from May to November 1995 (CitationFunakawa et al. 1992). Collected soil solution was preserved at 4°C until analysis.
The soil solutions were filtered using a 0.45-µm Millipore filter. The pH of each soil solution was determined using a glass electrode. The concentrations of Na, K, Mg and Ca were determined using AAS, and those of Al, Fe and Si using ICP-AES. The concentrations of Cl, NO3 and SO4 were determined by high performance liquid chromatography (HPLC) on an octa-decyl-silyl column (ODS-CLC; Shimadzu) using 0.8 mmol L−1 trimesic acid and 1.0 mmol L−1 tetra-n-butylammonium hydroxide solution in water at pH 4.6 as a mobile phase. The concentration of dissolved organic carbon (DOC) was determined using a total organic carbon analyzer (TOC–5000; Shimadzu).
Dissolved Al was speciated into three fractions based on the rate of reaction with 8-hydroxyquinoline (oxine) and reactivity to a strongly acidic cation-exchange resin as follows (CitationBloom and Erich 1989). The total amount of Al dissolved was determined using ICP-AES. The total amount of monomeric Al was determined by a rapid reaction with oxine (CitationBarnes 1975; modified by CitationLaZerte 1984). In this procedure, to keep the contact time of the oxine with any Al polymers to a minimum, the sample solution was treated by sequential addition of buffer solution (approximately 2 mol L−1 NH4OAc at pH 8.3), oxine reagent (5%[w/v] of 8-hydroxyquinoline solution) and methyl isobutyl ketone (MIBK) within 15 s. Then the MIBK extract was collected and the concentration of the Al–oxine complex was determined spectrophotometrically at 390 nm. The difference between the total dissolved Al and the total amount of monomeric species was assigned to strongly complexed Al, in which polymeric and/or colloidal Al would also be included. Monomeric Al was further speciated following the procedure of CitationHodges (1987). The amount of Al that was not adsorbed by a partially neutralized (to pH 4.2) acidic cation-exchange resin (Duolite IR-120B) in a column was determined using the oxine method and assigned to organic monomeric species. The difference between the total amount of monomeric Al and the amount of organic monomeric Al, the fraction not retained by the cation exchange resin, was assigned to inorganic monomeric Al species.
RESULTS
General physicochemical and mineralogical properties of the soils studied
The general physico-chemical and mineralogical characteristics of the selected soils, from which soil solution composition was measured, are presented in . The results are summarized as follows:
1. | The pH (H2O) of the soils, especially in the surface horizons, was very low, normally less than 5. The decrease in soil pH from the C horizon toward the surface reflects pedogenetic soil acidification processes. Among exchangeable cations, Al usually dominated and the base saturation of the soils was below 5% in most cases, suggesting that acid neutralization through cation exchange between H+ supplied and exchangeable bases of the soils was limited under the present situation. | ||||
2. | Most of the soils showed a rather fine texture, the clay contents usually exceeding 40%. The dominant clay mineral species were mostly 2:1–2:1:1 intergrades and vermiculite occasionally appeared in the highly acidified (pH [H2O] below 4.2) upper horizons. The Sio content of nearly zero suggests that amorphous Al–Si compounds were virtually absent, unlike Al hydroxides and/or organo-mineral complexes. | ||||
3. | B horizon soils in a MSTR contained higher amounts of amorphous compounds (Alo + 1/2Feo) and organic matter (Cp) than those in a TSTR, consistent with earlier results reported by CitationHirai et al. (1991). |
Titratable alkalinity and acidity of the soils
Acid and alkali titration curves of the selected soils are presented in . In general, the titratable alkalinity required to acidify the soils to pH 3.0 was higher in the B or C horizons than in the surface horizons for soils in the MSTR (TT and OT), whereas it was at a maximum at or near the surface for soils in the TSTR (MD1 and MD2). The titratable acidity required to neutralize soils to pH 8.3 increased from the C horizon toward the surface, presumably because of the increasing influence of organic matter dissociation. As this process may mask other important relationships between titration data and physicochemical properties, we refer only to soils with a total carbon content of less than 100 g kg−1 in the following discussion on the titration data.
Table 2 General physicochemical properties of the selected soils
shows correlation coefficients between the titration data and the selected chemical properties of the soil (n = 43). The titratable alkalinity required to acidify soils to pH 3.0 is highly correlated with some extractable Al or Fe compounds (Alo [r = 0.83**], Ald [r = 0.82**], Alp [r = 0.79**] and Feo [r = 0.74**]) and also with pH (NaF) (r = 0.73**). These correlations indicate the importance of amorphous Al, including organo-mineral complexes and probably Fe compounds as an active acid buffering component in soils with low base saturation through protonation of oxides and/or partial monomerization of Al as also reported for acidic forest soils by CitationKamoshita et al. (1979) and CitationFunakawa et al. (1993b). is a scattergram between Alo and titratable alkalinity to pH 3.0. The value of the slope, 0.22, indicates that only a small portion of the Alo fraction can contribute to the acid neutralization reaction. Thus, the titratable alkalinity derived from amorphous Al oxide was higher in soils under MSTR than in soils under TSTR.
Table 3 Correlation coefficients among the physicochemical properties and titration data of the soils (n = 43)
Figure 2 Relationships between (a) titratable alkalinity and Alo (extraction in the dark with acid [pH 3] 0.2 mol L−1 ammonium oxalate), (b) OH− consumption and exchangeable Al and (c) OH− consumption and pyrophosphate extractable C (Cp).
![Figure 2 Relationships between (a) titratable alkalinity and Alo (extraction in the dark with acid [pH 3] 0.2 mol L−1 ammonium oxalate), (b) OH− consumption and exchangeable Al and (c) OH− consumption and pyrophosphate extractable C (Cp).](/cms/asset/f9c6547d-0c53-4e93-9787-8433811fbff4/tssp_a_10382604_o_f0002g.gif)
Some titration curves display a conspicuous pH buffer zone in the pH range 4.5 to 5.5 (). Based on the fact that OH− consumption in this zone is highly correlated with exchangeable Al content (; r = 0.96**), the buffering effect can be attributed to the hydrolytic reactions of exchangeable Al. shows this correlation to have a slope of 0.48, indicating that the amount of OH− consumed in the pH range 4.5 to 5.5 is equivalent to half the exchangeable Al content. One reason for this discrepancy may derive from the incomplete hydrolysis of the Al3+ ion under relatively high rates of OH− addition. No clear relationship was observed between the extent of this buffering and the soil temperature regime of the samples.
The OH− consumption in the pH range 5.5 to 8.3 is correlated with total carbon content, Cp and Alp + 1/2Fep (; r = 0.90**, 0.90** and 0.80**, respectively), indicating that the acidity in this pH range originates from organo-mineral complexes via the dissociation of the acidic functional groups of soil humus and/or the deprotonation of oxide surfaces, as follows:
where M– represents metal ions (such as Fe or Al) at the surface of soil particles and R–COOH represents the acidic functional groups of organic matter. describes the relationship between Cp content and OH− consumption in the pH range 5.5 to 8.3. As the carboxylic functional group content of fulvic acid in cool temperate zone soils is reported to be 0.61–0.85 cmol g−1 organic matter (CitationStevenson 1982), the slope obtained in , 0.78 cmol g−1 C (equivalent to 0.46 cmol g−1 organic matter), seemed to be reasonable if most of the carboxylic groups of the Cp fraction react with added OH− as in the first and second reactions shown above. In early research relating to titration, the buffer region between pH 5.5 and 8.3 was often attributed to hydroxy-Al interlayer components (CitationSchwertmann and Jackson 1963, Citation1964). The third reaction above may also involve edges of layer silicates. Titratable acidity in this pH range was generally higher in MSTR rather than TSTR B horizon soils.
Soil solution composition
summarizes the soil solution composition for each soil profile. The concentrations of Na, Cl and SO4 in the soil solution from both surface and subsurface horizons were similar, suggesting that these elements are little affected by ion exchange reactions or absorption by plant roots. The notable exception is the case of SO4 in TT, in which the concentration of SO4 at a depth of 40 cm is almost half that at a depth of 15 cm. The concentrations of K, Mg and Ca usually decreased with depth in each profile, presumably because of plant uptake. In TT, active nitrification seems to promote leaching of Ca from the upper horizon.
Table 4 Soil solution composition
Figure 3 Relationships observed among the soil solution compositions. (a) Anion deficit and dissolved organic carbon (DOC), (b) inorganic monomeric Al (Alinorg) and pH and (c) organic monomeric Al (Alorg) and DOC.
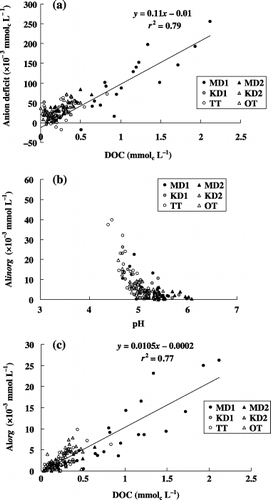
The concentration of DOC was generally lower than 1 mmol L−1, except for MD1, in which it sometimes reached 2 mmol L−1. shows a positive correlation between the concentration of DOC and the anion deficit of the soil solution, suggesting that the organic compounds behaved as anionic components in the soil solution. Judging from the slope of 0.11, the average content of dissociated acidic functional groups is equivalent to 110 mmolc mol−1 C. This value is slightly higher than the carboxylic group contents of the soil solution reported by CitationNambu and Yonebayashi (1999) for forest soils in Kyoto Prefecture, that is, 4.9–5.9 mmolc g−1 C (59–71 mmolc mol−1 C).
In KD2, TT and OT, the concentration of Al decreased in the subsoils with a concomitant increase in pH and decrease in DOC (), suggesting precipitation of Al through hydrolysis and/or coprecipitation of Al–organic matter complexes in the subsoils. As the decrease in Al concentration in the subsoils is accompanied by a decrease in both the sum of cations and the anion deficit in KD2 and OT, the precipitation of Al might be directly associated with the removal of organic anions from the solution phase. Although there were significant concentrations of Al in the soil solution, Fe was rarely present, indicating that translocation of Fe in the soil profiles did not occur.
In general, the concentration of total Al in the soil solution in this study was lower and the proportions of organic monomeric and/or strongly complexed Al were higher than for soils in northern Kyoto (CitationFunakawa et al. 1993a). The organic monomeric and strongly complexed Al fraction are still relatively small, however (less than 50% of total dissolved Al except for MD1, in which a higher concentration of DOC also exists), and the inorganic monomeric Al (Alinorg) is the major fraction. The concentration of Alinorg drastically decreases as the pH approaches 5 (). In contrast, the concentration of organic monomeric Al (Alorg) has a positive correlation with DOC concentration with a slope of 0.0105 mol mol−1 C (), suggesting that DOC supplied from the upper soil layers and/or litter layers is primarily responsible for mobilizing Al in the form of organo-mineral complexes.
DISCUSSION
Eluvi-illuviation of inorganic Al and subsequent adsorption of DOC
summarizes the soil solution composition and characteristics of the soil solid phase in each soil profile with the reaction of inorganic Al in the left figure and that of organic Al and DOC in the right figure. Three patterns of acid neutralization, that is, surface neutralization, subsurface neutralization and limited neutralization, were observed judging from titratable alkalinity and the concentration of Alinorg and the pH of the soil solution. In the MD1 and MD2 profile surface layers, the solution pH was higher than 5, Alinorg below 5 × 10−3 mmol L−1 and titratable alkalinity higher than 10 cmol kg−1. Most of the acidity supplied by the soil surface, if any, is probably neutralized in these layers (surface neutralization). In the surface horizons of the other profiles (KD1, KD2, TT and OT), the titratable alkalinity of the surface soils is lower than 10 cmol kg−1, solution pH is 5 or below, and larger concentrations of Alinorg (> 5 × 10−3 mmol L−1) are dissolved. In the KD2 and OT profiles the concentrations of Alinorg in the soil solution decreased at or below the AB or Bw1 horizon, along with a pH rise to approximately 5.2, and an increase in titratable alkalinity to over 10 cmol kg−1 in subsoil layers (subsurface neutralization). In contrast, the titratable alkalinity of the KD1 and TT soils never exceeded 10 cmol kg−1, solution pH remains at or below 5, and Alinorg concentrations are high even in the B horizons (limited neutralization). These results show that a large part of the soil solution acidity can be removed and, as a result of the rise in pH, Alinorg in the solution precipitated through hydrolysis in the soil layers has high titratable alkalinity, that is, > 10 cmol kg−1 in this study. Although such an acidity-neutralizing layer was found at or near the soil surface under TSTR (MD1 and MD2), it was occasionally formed in the subsoil under MSTR. presents northern Kyoto MSTR forest soil data in the same format as (data from CitationFunakawa et al. 1993a,Citationb). Two profiles (S3 and N4b) have an acid-neutralizing layer in the B horizons (subsurface neutralization) and another one (H2) probably shows a similar trend in the upper B horizon. The remaining H1b profile (Podzolic soils) has already largely lost the alkalinity derived from amorphous compounds of Al because of intensive acidification and, therefore, the solution pH never increases to above 5, even in the lowermost horizon. Thus, soil solution acidity is often neutralized in the subsoil under MSTR, resulting in accumulation of solution phase Al through hydrolysis and precipitation. Dissolved organic matter in the soil solution, still possessing a certain concentration of dissociated acidic functional groups, can be adsorbed on these amorphous compounds of Al. Thus, as organo-mineral complexes accumulated in B horizons are considered to also comprise the active fraction of titratable acidity, the process described here also contributes to the increase in titratable acidity in the B horizon soils.
Figure 4 Chemical properties and titration data of the soil profiles superimposed with the soil solution composition. Error bars are standard error. Values with the same letter are not significantly different (P < 0.05). Alo, Feo, extraction in the dark with acid (pH 3) 0.2 mol L−1 ammonium oxalate; Alp, Fep, extraction with 0.1 mol L−1 pyrophosphate at pH 10; DOC, dissolved organic carbon.
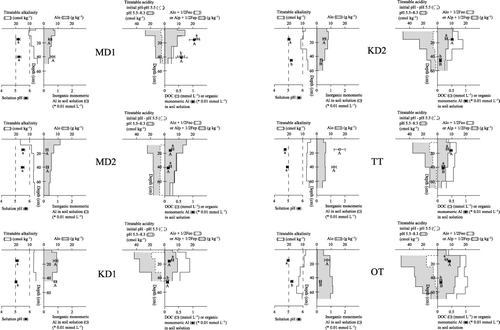
Comigration of Al and DOC in the soil profiles
The concentration of Alorg in the soil solution substantially decreases with depth in KD2, TT and OT (, ). As DOC also decreases to some extent with depth, precipitation of complexes of Al and dissolved organic matter complexes may have occurred in the subhorizon, as discussed by CitationDeConinck (1980) for the formation of spodic horizons. As fairly large amounts of amorphous compounds (Alo + 1/2Feo) already exist in the subhorizons, adsorption of Al–dissolved organic matter complexes onto amorphous compounds may also be possible. As shows that all of the forest soils studied in northern Kyoto also show such a trend, this process may be involved in soil formation under MSTR. It seems, to some extent, similar to podzol formation, at least in terms of Al translocation, and enhances the accumulation of titratable acidity in subsoils.
Dynamics of titratable alkalinity and acidity in the pedogenetic processes
summarizes the amounts of titratable acidity, titratable alkalinity and total C in the soil profiles on a kmol ha−1 basis using all the data from the present study (12 profiles) and data from previous studies (CitationFunakawa et al. 1993b[8 profiles] and CitationFunakawa et al. 2003[4 profiles from northern Kyoto: ON1 and ON2 in TSTR and KT1 (podzolic soil) and KT2 in MSTR]). As discussed previously, pedogenetic acidification in forest soils with MSTR is characterized by an accumulation of acidity in the form of amorphous compounds and/or organo-mineral complexes in the B horizons, originally supplied from the overlying horizon. shows that the titratable acidity in subsoil layers (15–45 cm) amounts to 292–816 kmol ha−1 and is higher among soils in MSTR than in TSTR because of a higher contribution of organo-mineral complexes (represented by Cp). As the in situ annual acid load on acid soils (i.e. low and intermediate rates of soil acidification) are reported to mostly range from 1 to 7 kmol ha−1 year−1 (Citationvan Breemen et al. 1984), the amounts of titratable acidity observed in the present study are equivalent to hundreds of times the annual acid load. The cumulative acid load that accompanies DOC adsorption and/or precipitation enhances the accumulation of total C in the subsoil layers (). As the DOC flux that can accumulate between the 15 cm and 40 or 45 cm layers of soils is calculated to be 0.10 (MD2) to 0.66 (MD1) Mg ha−1 year−1 (average: 0.28) based on the soil solution composition in the present study under the assumption of annual amount of percolating water to be 1,000 mm, the amounts of organic C accumulated in the subsoil layers (i.e. 25–112 Mg ha−1; ) also corresponding to hundreds of times the annual DOC flux. Thus, the pedogenetic processes, including acid transfer and C migration to the subsoil layers are considered to be prolonged at least for hundreds of years.
According to , the amounts of titratable alkalinity in the 15–45 cm layers of soils tend to be higher compared with those in the surface 15 cm layers of soils in MSTR. As amorphous Al hydroxides (Alo) compose major parts of the titratable alkalinity, they play an important role in retarding further acidification through protonation and/or partial monomerization. In this context, amorphous Al hydroxides, which would otherwise be leached out directly from the soil profile, can be regarded as making a temporary contribution to the acid neutralizing capacity of the soil. This process delays the outflow of acids (H+ or Al3+) from soils. Both stepwise processes of precipitation of inorganic Al and a subsequent adsorption of DOC, and comigration and precipitation of Al–soluble organic matter complexes are involved in the accumulation processes of organo-mineral complexes in the B horizons. In contrast, for soils under TSTR, the acid-buffering reactions, if any, occur mostly at or near the soil surface. One possible explanation for such an apparent deep penetration of soil acidification in MSTR is that under MSTR the amount of percolating water is higher than under TSTR because the precipitation is higher and evapotranspiration is lower at high elevations in the region, and that such a difference in the degree of leaching possibly brings more intensive soil acidification under MSTR. The fact that the titratable alkalinity required to acidify soils to pH 3.0 and the titratable acidity required to neutralize soils to pH 8.3 in B horizon soils is apparently lower in TSTR soils than MSTR soils () reflects the lower accumulation of amorphous and/or organo-mineral complexes in the B horizons of the former. The classification scheme proposed by CitationHirai et al. (1991), which emphasizes the properties of free oxides in B horizon soils, is pedogenetically supported by the dynamics of acidity/alkalinity and SOM observed in the present study.
Figure 5 Chemical properties and titration data of the soil profiles in northern Kyoto superimposed with the soil solution composition. Data are cited from Funakawa et al. (1993a). Error bars are standard error. Values with the same letter are not significantly different (P < 0.05). Alo, Feo, extraction in the dark with acid (pH 3) 0.2 mol L−1 ammonium oxalate; Alp, Fep, extraction with 0.1 mol L−1 pyrophosphate at pH 10; DOC, dissolved organic carbon.
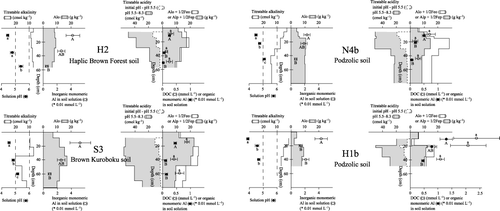
Conclusions
Based on the soil solution composition and titratable alkalinity and acidity in the soil profiles, two processes were postulated for pedogenetic acidification, that is, eluvi-illuviation of inorganic Al followed by subsequent adsorption of DOC and comigration of Al and DOC in the form of organo-mineral complexes. Both processes were conspicuous in MSTR soils and significantly contributed to SOM storage in the subsoil layers. Pedogenetic acidification in forest soils with MSTR was characterized by an accumulation of acidity in the form of amorphous compounds and/or organo-mineral complexes in the B horizon. It seems, to some extent, similar to podzol formation, at least in terms of Al translocation. Amorphous Al hydroxides protect against further acidification through protonation and/or partial monomerization and can, thus, be regarded as a temporary storage of acid neutralizing capacity of the soil, which would otherwise be leached out directly from the soil profile. In contrast, the acid-buffering reactions of TSTR soils seemed to occur, if at all, mostly at or near the soil surface and the contribution of the B-horizon soils was limited. Thus, the pedogenetic processes in MSTR and TSTR were different in terms of the dynamics of acidity and Al and, hence, the proposed soil classification system (the Unified Soil Classification System of Japan, 2nd Approximation) can be useful when we consider the environmental functions and behavior of forest soils in relation to SOM storage and long-term acidification.
Notes
Present addresses: Industrial Technology Center, Kyoto Municipal Industrial Research Institute, Kyoto 600-8813, Japan.
Faculty of Bioresources and Environmental Sciences, Ishikawa Prefectural University, Ishikawa 921-8836, Japan.
REFERENCES
- Barnes , RA . 1975 . The determination of specific forms of aluminum in natural water . Chem. Geol , 15 : 177 – 191 .
- Bloom , PR and Erich , MS . 1989 . “ The quantification of aqueous aluminum ” . In The Environmental Chemistry of Aluminum , Edited by: Sposito , G. 1 – 27 . Florida : CRC Press .
- Davis , JA and Hem , JD . 1989 . “ The surface chemistry of aluminum oxides and hydroxides ” . In The Environmental Chemistry of Aluminum , Edited by: Sposito , G. 185 – 219 . Florida : CRC Press .
- DeConinck , F . 1980 . Major mechanisms in formation of spodic horizon . Geoderma , 24 : 108 – 128 .
- Driscoll , CT . 1989 . “ The chemistry of aluminum in surface waters ” . In The Environmental Chemistry of Aluminum , Edited by: Sposito , G. 241 – 277 . Florida : CRC Press .
- Fourth Committee for Soil Classification and Nomenclature . 2002 . Unified Soil Classification System of Japan (2nd Approximation) (Final Report (The Extract)) . Pedologist , 46 : 36 – 45 . (in Japanese)
- Funakawa , S , Ashida , M and Yonebayashi , K . 2003 . Charge characteristics of forest soils derived from sedimentary rocks in Kinki District, Japan, in relation to pedogenetic acidification process . Soil Sci. Plant Nutr , 49 : 387 – 396 .
- Funakawa , S , Hirai , H and Kyuma , K . 1992 . Soil-forming processes under natural forest north of Kyoto in relation to soil solution composition . Soil Sci. Plant Nutr , 38 : 101 – 112 .
- Funakawa , S , Hirai , H and Kyuma , K . 1993a . Speciation of Al in soil solution from forest soils in northern Kyoto with special reference to their pedogenetic process . Soil Sci. Plant Nutr , 39 : 281 – 290 .
- Funakawa , S , Nambu , K , Hirai , H and Kyuma , K . 1993b . Pedogenetic acidification of forest soils in northern Kyoto . Soil Sci. Plant Nutr , 39 : 677 – 690 .
- Helyer , KR and Porter , WM . 1989 . “ Soil acidification, its measurement and processes involved ” . In Soil Acidity and Plant Growth , Edited by: Robson , AD . 61 – 101 . Sydney : Academic Press .
- Hirai , H , Yoshikawa , K , Funakawa , S and Kyuma , K . 1991 . Characteristics of brown forest soils developed under different bio-climatic conditions in the Kinki district with special reference to their pedogenetic processes . Soil Sci. Plant Nutr , 37 : 639 – 649 .
- Hodges , SC . 1987 . Aluminum speciation: a comparison of five methods . Soil Sci. Soc. Am. J , 51 : 57 – 64 .
- Johnson , DW , Lindberg , SE and Pitelka , LF . 1992 . “ Introduction ” . In Atmospheric Deposition and Forest Nutrient Cycling , Edited by: Johnson , DW and Lindberg , SE . 1 – 7 . New York : Springer-Verlag .
- Kamoshita , Y , Kojima , M , Hasegawa , Y and Satoh , Y . 1979 . “Ko-Sanenki-Hi” An experimental numeral to learn soil buffer against acid and base . J. Soil Sci. Manure Jpn , 50 : 249 – 254 . (in Japanese)
- Kinniburgh , DG . 1986 . Towards more detailed methods for quantifying the acid susceptibility of rocks and soils . J Geol. Soc , 143 : 679 – 690 .
- LaZerte , BD . 1984 . Forms of aqueous aluminum in acidified catchments of central Ontario: a methodological analysis . Can. J. Fish Aquat. Sci , 41 : 766 – 776 .
- McKeague , JA and Day , JH . 1966 . Dithionite- and oxalate-extractable Fe and Al as aids in differentiating various classes of soils . Can. J. Soil Sci , 46 : 13 – 22 .
- Mehra , OP and Jackson , ML . 1960 . Iron oxide removal from soils and clays by a dithionite-citrate system buffered with sodium bicarbonate . Clays Clay Miner , 7 : 317 – 327 .
- Nambu , K and Yonebayashi , K . 1999 . Acidic properties of dissolved organic matter leached from organic layers in temperate forest . Soil Sci Plant Nutr , 45 : 65 – 77 .
- Parfitt , RL . 1978 . “ Anion adsorption by soils and soil materials ” . In Advances in Agronomy , Edited by: Brady , NC . Vol. 30 , 1 – 50 . New York : Academic Press .
- Schuppli , PA , Ross , GJ and McKeague , JA . 1983 . The effective removal of suspended materials from pyrophosphate extracts of soils from tropical and temperate regions . Soil Sci. Soc. Am. J , 47 : 1026 – 1032 .
- Schwertmann , U and Jackson , ML . 1963 . “ Hydrogen-aluminum clays: a third buffer range appearing in potentiometric titration ” . In Science Vol. 139 , 1052 – 1054 . Washington, DC
- Schwertmann , U and Jackson , ML . 1964 . Influence of hydroxy aluminum ions on pH titration curves of hydronium–aluminium clays . Soil Sci. Soc. Am. Proc , 28 : 179 – 183 .
- Soil Survey Staff . 2006 . Keys to Soil Taxonomy , 10th edn , Washington : US Government Printing Office .
- Stevenson , FJ . 1982 . “ Reactive functional groups of humic substances ” . In Humus Chemistry; Genesis, Composition, Reactions , Edited by: Stevenson , FJ . 221 – 243 . New York : John Wiley & Sons .
- Thomas , GW . 1982 . “ Exchangeable cations ” . In Methods of Soil Analysis, Part 2, Chemical and Mineralogical Properties , Edited by: Page , AL , Miller , RH and Keeney , DR . 159 – 165 . Madison : American Society of Agronomy and Soil Science Society of America .
- Tinsley , J . The determination of organic carbon in soils by dichromate mixture . Transactions of the 4th International Congress of Soil Science . 1986 , Hakuyusha, Tokyo. Edited by: Standard Soil Analyses and Methods Committee Japan . Vol. 1 , pp. 161 – 164 . cited from Standard Soil Analyses and Methods, [in Japanese])
- van Breemen , N , Driscoll , CT and Mulder , J . 1984 . Acidic deposition and internal proton sources in acidification of soils and waters . Nature , 307 : 599 – 604 .
- van Breemen , N , Mulder , J and Driscoll , CT . 1983 . Acidification and alkalization of soils . Plant Soil , 75 : 283 – 308 .
- Present addresses: Industrial Technology Center, Kyoto Municipal Industrial Research Institute, Kyoto 600-8813, Japan.
- Faculty of Bioresources and Environmental Sciences, Ishikawa Prefectural University, Ishikawa 921-8836, Japan.