Abstract
It is widely recognized that the efficiency of substrate C use in acid and/or disturbed soils by soil microorganisms is relatively low based on the observation that metabolic quotients (qCO2) are usually high in these soils. In the present study, threshold concentrations of glucose, at which the ratio of respiration to assimilation by soil microorganisms began to increase, were comparatively analyzed using two soils differing in pH and disturbance, a Japanese arable soil disturbed by cultivation and a strongly acidic Japanese forest soil. Varying concentrations of glucose C, generally less than those in the microbial biomass C, were added to the two soils. The ratio of respired to utilized glucose C remained at approximately 20% when lower concentrations of glucose were added and respiration rates did not increase (zero-order types), whereas the ratio increased when the concentrations of added glucose exceeded a certain level and respiration rates increased (growth-associated types). The substrate-induced respiration rate a few hours after the addition of glucose increased only for the growth-associated types, although chloroform-labile C increased in both types as the concentrations of added glucose increased. The results clearly confirmed the presence of a threshold concentration of glucose above which the ratio of respiration to assimilation increased. The threshold concentrations in Japanese arable and forest soils were lower than the concentration previously reported in a moderately acidic Kazakh forest soil. The lower threshold concentrations observed in the Japanese arable and forest soils are considered to result from different microbial growth characteristics after the addition of glucose linked with a shorter lag period before the exponential increase of the respiration rate and a lower ratio of substrate induced respiration rate to biomass C, respectively. The results suggest that the efficiency of substrate C use in acid and disturbed soils is relatively low in situations where higher concentrations of substrates are occasionally supplied with temporal C ‘flushes’, such as may occur in the rhizosphere or in the vicinity of plant residues.
Introduction
Microorganisms are central to the carbon (C) cycle in soils because a large fraction of the C flow in soils passes through the microbial biomass. This organic C provides not only a source of energy, resulting in the formation of CO2 (respiration), but also a source of C for the production of new cells (assimilation) (CitationScow 1997). Therefore, the ratio of respiration to assimilation indicates the efficiency of soil microbes in their use of substrate C: the higher this ratio, the less efficient their use of substrate C.
The ratio of the soil respiration rate to microbial biomass C (the metabolic quotient, qCO2) can be considered to be an indicator of the relative efficiency of substrate C use by soil microbes (CitationWardle and Ghani 1995). Several studies have shown increases in qCO2 after disturbance (e.g. CitationFritze et al. 1994; CitationXu et al. 2007). In addition, several studies have shown that qCO2 is negatively correlated with soil pH (e.g. CitationAnderson and Domsch 1993). This suggests that soil microbes in acid soils use C less efficiently because they divert energy from growth to cell maintenance functions. However, qCO2 is an indirect index and may not be a good indicator of soil microbial efficiency in C use because it does not incorporate the utilization of substrate C by the microbial biomass (CitationKillham 1985). Therefore, several authors have directly measured the ratio of respired C to glucose C (as a model substrate) utilized by microbes in soils subject to stress, such as by heavy metal contamination and in acid soils. CitationChander and Brookes (1991) and CitationBardgett and Saggar (1994) observed that the ratio of respired 14C to utilized glucose 14C in soils subject to stress was higher than that in non-stressed soils. However, CitationWitter and Dahlin (1995) and CitationDahlin and Witter (1998) observed that this ratio in soils subject to stress was not significantly different from that in non-stressed soils. These results were obtained after the addition of a single concentration of glucose with a higher C content than biomass C. Several studies have shown that the ratio of respired C to utilized glucose C increases with increasing concentrations of added glucose (CitationBremer and Kuikman 1994; CitationBremer and van Kessel 1990; CitationMarstorp and Witter 1999; CitationNguyen and Henry 2002; CitationSchneckenberger et al. 2008; CitationTsai et al. 1997; CitationWitter and Kanal 1998). CitationSawada et al. (2008) suggested that a moderately acidic undisturbed Kazakh forest soil had a threshold concentration of glucose to increase the ratio of respired to utilized glucose C when lower concentrations of glucose than biomass C were added. Because in the actual soil environment, the concentrations of substrates are usually not high (CitationHill et al. 2008; CitationSchneckenberger et al. 2008), a detailed understanding of this threshold concentration is necessary to understand the actual efficiency of microbial substrate C use.
The main objective of the present study was to determine the threshold concentrations of glucose at which the ratio of respiration to assimilation by soil microorganisms begins to increase in three soils with different soil pH values and degrees of disturbance. The soils used were a Japanese arable soil disturbed by cultivation, a strongly acidic Japanese forest soil and a previously reported moderately acidic Kazakh forest soil (CitationSawada et al. 2008). Possible explanations for the threshold concentrations are discussed.
Materials and methods
Soils
Soil samples were collected from the 0–10 cm layer of two mineral soils in Japan after the removal of plant debris. One sample was from an arable soil in a Chinese cabbage field at the Experimental Farm of Kyoto University, Osaka (34°51′N, 135°38′E). This soil is developed on the alluvial fan of a small river and is classified as Fluvisols. The other sample was from a forest soil with a 5-cm thick O horizon under deciduous forest, predominantly oak (Quercus mongolica), on Tango Peninsula, Kyoto (35°38′N, 135°10′E). The physicochemical properties and microbial biomass C of the both soils are listed in . Microbial biomass C was determined by the chloroform fumigation–extraction method (CitationVance et al. 1987) using a conversion factor (kEC) of 0.45 (CitationWu et al. 1990). Freshly collected soil samples were sieved to pass through a 2 mm sieve and stored at 5°C until use. All soil measurements are expressed on an oven-dry weight basis.
Soil preparation for incubation
The soils were incubated at 25°C at moisture contents of 35% and 55% of the maximum water holding capacity for Japanese arable and forest soils, respectively. These water contents for the incubation experiment were selected to obtain maximum substrate-induced respiration rates and specific respiration increments (CitationNordgren et al. 1988; CitationSawada et al. 2009) based on a preliminary experiment in which substrate-induced respiration rates and specific respiration increments were determined at various water contents. Soil samples were pre-incubated in Erlenmeyer flasks for 1 week to reduce the influence of any disturbance resulting from collection and sieving. The water contents at pre-incubation were set to a value a little lower than that for incubation, that is, 30% and 50% of the maximum water-holding capacity for Japanese arable and forest soils, respectively. After pre-incubation, the soils were treated with glucose, NH4NO3 and KH2PO4. Glucose was added to the Japanese arable soil at seven rates: 30, 40, 50, 60, 85, 250 and 1000 μg C g−1 soil, and to the Japanese forest soil at nine rates: 100, 150, 200, 250, 300, 500, 1000, 2000 and 4000 μg C g−1 soil. The concentrations of added glucose were equivalent to 12, 16, 20, 24, 33, 98 and 393% of microbial biomass C and 10, 13, 17, 20, 28, 83 and 333 mmol L−1 in the soil solution for the Japanese arable soil, and 6, 9, 12, 16, 19, 31, 62, 125 and 250% of microbial biomass C and 6, 9, 12, 15, 18, 39, 61, 122 and 243 mmol L−1 in the soil solution for the Japanese forest soil. Controls with the addition of equivalent amounts of water without glucose were also included. Nitrogen and phosphorus were supplemented to give a C : N : P ratio of 10:1:1 at the maximum glucose addition rate, so that C uptake was not limited. Thus, NH4NO3 and KH2PO4 were added at rates of 100 μg N g−1 soil and 100 μg P g−1 soil to the Japanese arable soil and 400 μg N g−1 soil and 400 μg P g−1 soil to the Japanese forest soil. All the above were dissolved in water prior to addition, increasing the soil moisture by 35% and 55% of the maximum water-holding capacity for Japanese arable and forest soils, respectively. Supplemented soil samples were homogenized thoroughly by mixing for 1 min with a spatula. All measurements were conducted in triplicate.
Table 1 Physicochemical and microbial properties of the soils
Microbial analysis
The respiration rate, K2SO4-extractable C, chloroform-labile C and substrate-induced respiration rate for the Japanese arable and forest soils were measured according to the method described by CitationSawada et al. (2008) with minor modifications. The respiration rates in 10 g (dry weight) control samples without glucose for the Japanese arable soil could not be clearly detected because the microbial biomass C was lower than that in the Kazakh forest soils used in the previous report (CitationSawada et al. 2008). In addition, the respiration rates in the 10 g samples after the addition of glucose were similar to those in 30 g samples in the Japanese arable soil. Therefore, 30 and 10 g (dry weight) samples for the Japanese arable and forest soils, respectively, were supplemented with glucose and nutrients and placed in 100 mL Erlenmeyer flasks. Respiration rates were measured every 1 or 2 h after the addition of glucose. The supplemented 5 g and 3 g (dry weight) samples for the Japanese arable and forest soils, respectively, were placed in 50 mL glass vials, covered with aluminum foil and K2SO4-extractable C was measured every 3 h after the addition of glucose. The chloroform-labile C and substrate-induced respiration rate were determined 6 h and 12 h after the addition of glucose for the Japanese arable and forest soils, respectively.
Statistics
All results are expressed as means with respective standard errors from triplicate determinations. Statistical analysis of the means of two independent samples was conducted using the a t-test with a significance level of 95% (P < 0.05).
Results
Ratio of respired to utilized glucose C
The dynamics of the respiration rates over time (respiration profiles) are shown in . Respiration profiles after the addition of glucose can be classified into zero-order and growth-associated types (CitationSawada et al. 2008; CitationStenström et al. 2001). The results of 30, 40, 50 and 60 μg C g−1 soil and 100, 150, 200, 250 and 300 μg C g−1 soil glucose additions for the Japanese arable and forest soils, respectively, represent the zero-order type, in which the respiration rate initially remains constant at a maximum initial respiration rate, and then subsequently decreases with glucose depletion (). The results of 85, 250 and 1000 μg C g−1 soil and 500, 1000, 2000 and 4000 μg C g−1 soil glucose addition for the Japanese arable and forest soils, respectively, represent the growth-associated type, in which the respiration rate initially remains constant at a maximum, and then increases as a result of microbial growth ().
To estimate when the microorganisms had utilized all of the glucose, K2SO4-extractable C was measured over time after the addition of various concentrations of glucose (). As it was assumed that decreases in K2SO4-extractable C reflect the glucose utilized by microbes, the time when all glucose was utilized was estimated to be when K2SO4-extractable C in the supplemented sample was not significantly different from that in the control sample. As the K2SO4-extractable C after the addition of 2000 and 4000 μg C g−1 soil of glucose to the Japanese forest soil was always significantly higher than that in the control sample, it was assumed that the time when the K2SO4-extractable C became constant after the addition of 2000 and 4000 μg C g−1 glucose was the time when all glucose was utilized according to CitationSawada et al. (2008).
The extra C respired after the addition of glucose was estimated as the difference in CO2 evolution between the supplemented and non-supplemented samples until the time when all the glucose was utilized. The concentrations of added glucose C, the respiration profiles, the time until all glucose was utilized and the ratios of respired to utilized glucose C are summarized in . The respired C was approximately 20% of the utilized glucose C for both soils when the respiration profiles were of the zero-order type (). The respired C was >20% of utilized glucose C and increased as the concentrations of added glucose increased for both soils, when respiration profiles were of the growth-associated type ().
Chloroform-labile C and substrate-induced respiration rates
Chloroform-labile C and substrate-induced respiration rates were measured at 6 and 12 h after the addition of various concentrations of glucose for the Japanese arable and forest soils respectively (). The results show that glucose additions caused increases in chloroform-labile C with both zero-order and growth-associated respiration rates. No significant differences between chloroform-labile C at zero glucose addition and low concentrations of glucose addition (i.e. 30, 40 and 50 μg C g−1 glucose addition and 100, 150 and 200 μg C g−1 glucose addition for the Japanese arable and forest soils, respectively) were detected because of the small differences in extractable C with and without chloroform fumigation at low concentrations of added glucose. No increase in the substrate-induced respiration rate was observed with the zero-order respiration type, although there was an increase with the growth-associated respiration type.
Discussion
Threshold concentrations of glucose that increase the ratio of respiration to assimilation
The ratios of respired to utilized glucose C were constant at approximately 20% when glucose C was added at rates giving the zero-order respiration type, and the ratios were >20% and increased when increasing concentrations of added glucose C gave the growth-associated respiration type for both the Japanese arable and forest soils (). The ratios of respired to utilized glucose C plotted as a logarithmic plot of added glucose C concentrations against biomass C (%) are shown in , which includes data for the Kazakh forest soil from table 2 in CitationSawada et al. (2008). Our results suggest that there are threshold glucose concentrations at which the ratio of respiration to assimilation increases, not only in the moderately acidic Kazakh forest soil (pH (H2O) of 5.7) (CitationSawada et al. 2008), but also in the Japanese arable soil disturbed by cultivation and the strongly acidic Japanese forest soil.
CitationBremer and Kuikman (1994) proposed a conceptual model in which the glucose acquired by soil microbes first enters a small intermediate pool and is then incorporated into either storage compounds or storage compounds together with structural compounds only if sufficient glucose is available for growth. This model also suggests that glucose incorporation into storage compounds requires less respiration and more assimilation than that needed for incorporation into structural compounds. Our results suggest that glucose C that was not respired was incorporated into the storage pool for the zero-order respiration type, and was used to synthesize structural compounds only for the growth-associated respiration type. In all three soils, there was a threshold glucose concentration above which structural compounds start to be synthesized in the soil microbes and the ratio of respiration to assimilation increases. This is supported by , in which glucose additions caused increases in chloroform-labile C with both zero-order and growth-associated respiration rates, but showed increases in the substrate-induced respiration rate only with the growth-associated respiration type for both Japanese arable and forest soils. It is assumed that although chloroform-labile C includes storage compounds (CitationBremer and Kuikman 1994; CitationNguyen and Guckert 2001), the substrate-induced respiration rate does not because these compounds would be a passive biomass component and would not respond to glucose. Therefore, it appears that, although the addition of glucose caused increases in the storage pool with both zero-order and growth-associated respiration rates, structural compounds were synthesized for just the growth-associated type not only in the Kazakh forest soil (CitationSawada et al. 2008) but also in the Japanese arable and forest soils.
Figure 2 K2SO4-extractable C as a function of time after the addition of various concentrations of glucose for Japanese arable (a) and forest (b,c) soils. The numbers in the key show the concentrations of added glucose (μg C g−1 soil). Error bars indicate standard errors (n = 3). Significant differences between extractable-C with and without the addition of glucose at *P = 0.05.
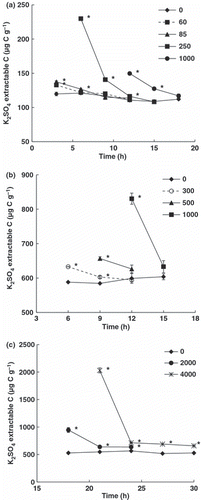
Most previous studies that have examined the effect of added glucose on the ratio of respired to utilized glucose C have shown that these ratios increased with increasing amounts of added glucose (CitationBremer and Kuikman 1994; CitationMarstorp and Witter 1999; CitationNguyen and Henry 2002; CitationTsai et al. 1997; CitationWitter and Kanal 1998). In contrast, our study found that there is a threshold concentration of glucose above which these ratios increase because the tested concentrations were generally less than those in the microbial biomass C. Recently, CitationSchneckenberger et al. (2008) investigated the ratio of respired to added glucose 14C after the addition of a wide range of glucose 14C concentrations ranging from 0.29 μmol L−1 to 80 mmol L−1 and from 0.0003 to 77.6% of biomass C. They observed a positive correlation between the concentrations of added glucose and these ratios 22 days after the addition of glucose. This conclusion differs from our study, where the respired C was constant at approximately 20% of the utilized glucose C when the added glucose was <20.0, 18.2 and 40.5 mmol L−1 in the soil solution and 23.6, 18.7 and 46.7% of biomass C for the Japanese arable and forest, and Kazakh forest soils, respectively. As in our study, CitationHill et al. (2008) also observed that there was no concentration-related trend in the respired to utilized glucose 14C ratio 1 h after the addition of low concentrations of glucose ranging from 1 μmol L−1 to 10 mmol L−1. These differences can be attributed to differences in the time over which the experiments were measured. As CitationSchneckenberger et al. (2008) made measurements 22 days after the addition of glucose, 14C assimilated into the biomass would probably have been re-mineralized for maintenance energy. In our study and in the study by CitationHill et al. (2008), the measurements were made over a very short time period and thus respiration came mainly from the energy required for growth and less from maintenance energy requirements.
Table 2 Respiration profiles, time taken until all glucose was utilized (h) and the ratio of extra C respired to utilized glucose C (%) after the addition of various concentrations of glucose to the Japanese arable and forest soils
Ratio of respired to utilized glucose C in Japanese arable and forest soils
It is widely recognized that in acid and/or disturbed soils the efficiency of substrate C use by soil microbes is relatively low based on the observation that qCO2 is usually high in these soils, and the assumption that qCO2 is an indicator of the relative efficiency of soil microbial C use (CitationWardle and Ghani 1995). Although qCO2 may be an indirect index, several authors have directly measured the ratio of respiration to assimilation of glucose C in soils subject to stress, such as by heavy metal contamination and in acid soils. These authors have typically measured the ratio of respired to increased biomass C using the chloroform–fumigation extraction method after the addition of glucose (e.g. CitationChander and Joergensen 2002). However, the recoveries of glucose C as biomass C plus the respired C and the remaining unutilized glucose C were often below 100%. It is unclear whether recoveries lower than 100% can be explained either by the non-inclusion of non-biomass microbial residual C as a result of microbial turnover, or by the use of a low extractability (kEC) value. Therefore, we focused on the ratio of respired to utilized glucose C in the present study. CitationChander and Brookes (1991) and CitationBardgett and Saggar (1994) observed that the ratio of respired 14C to added glucose 14C in soils subject to stress was higher than that in non-stressed soils. However, they did not adjust the concentration of added glucose to the microbial biomass C. Therefore, CitationWitter and Dahlin (1995) examined these ratios when the concentrations of added glucose were regulated to correspond approximately with the concentrations of biomass C. They observed that, in soils subject to stress, these ratios were not significantly different from those in non-stressed soils. In addition, CitationDahlin and Witter (1998) also observed that the ratios of total C (12C + 14C) respired to added glucose 14C in soils subject to stress were not significantly different from those in non-stressed soils when the concentrations of added glucose were regulated to be approximately three times the concentration of the biomass C. In the present study, these measurements were made a few days after the addition of the glucose, during which time all the added glucose should have been utilized because glucose is not adsorbed by soil particles (CitationJones and Edwards 1998) and turns over very rapidly (as much as 100 to 1000 times each day; CitationHill et al. 2008). Therefore, the ratios of respired to utilized glucose C should not be affected by stress when the concentrations of added glucose are regulated to be approximately a few times the concentration of biomass C. Our results support this, showing no clear effect of acidity or disturbance on these ratios when the added glucose C exceeded biomass C (above 100% on the x-axis in ).
Figure 3 Chloroform-labile C (♦) and substrate-induced respiration rates (▀) plotted against the concentrations of added glucose at 6 and 12 h after the addition of various concentrations of glucose for Japanese arable (a) and forest (b) soils, respectively. Error bars indicate standard errors (n = 3). Significant differences between chloroform-labile C or substrate-induced respiration rates with and without the addition of glucose at *P = 0.05. G, growth-associated respiration type; Z, zero-order respiration type.
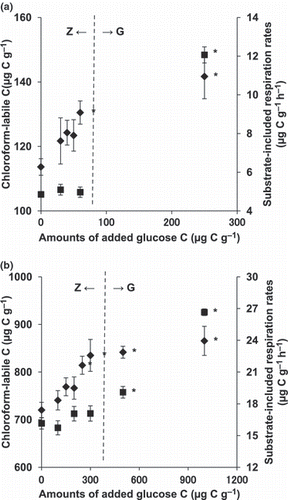
The concentrations of readily available substrate are usually <50 μmol L−1 in the root-free soil, with the highest concentrations of monosaccharides and disaccharides in soils being found in root cells, ranging from 10 to 90 mmol L−1 (CitationJones and Darrah 1996). These concentrations are usually less than those in the biomass C. Therefore, the ratio of respiration to assimilation after the addition of more glucose C than is in the biomass C will not reflect actual events. In the present study, these ratios were examined after the addition of various concentrations of glucose C less than those in the biomass C. When the added glucose was below the threshold concentrations, approximately 20% of the utilized glucose C was respired not only in the moderately acidic Kazakh forest soil, but also in the cultivated Japanese arable soil and the strongly acidic Japanese forest soil (). Thus, the efficiency of soil microbes in substrate C use was not affected by acidity or disturbance in root-free soils where the concentrations of the readily available substrate were low.
Figure 4 Ratio of respired to utilized glucose C plotted on a logarithmic scale of the concentrations of added glucose C to biomass C (%). Error bars indicate standard errors (n = 3). Data for the Kazakh soil are from Sawada et al. (2008).
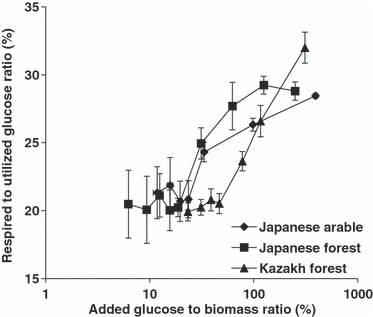
The threshold concentrations of glucose were 23.6–33.4%, 18.7–31.2% and 46.7–77.8% of biomass C and 20.0–28.3 mmol L−1, 18.2–30.4 mmol L−1 and 40.5–67.4 mmol L−1 in the soil solution in the Japanese arable and forest soils and the Kazakh forest soil, respectively; these concentrations were lowerin the Japanese arable and forest soils than in the Kazakh forest soil. Therefore, the ratios of respired to utilized glucose C in the Japanese arable and forest soils were higher than in the Kazakh forest soil when the concentrations of substrates were approximately 20–80% of biomass C, and approximately 20–70 mmol L−1 in soil solution (). The efficiency of substrate C use in the Japanese arable and forest soils would decrease more readily than in the Kazakh forest soil if higher concentrations of easily available substrate were added, for example, if soils experience temporal C ‘flushes’, which can occur in the rhizosphere or around plant residues.
Effect of pH and disturbance on the threshold concentration of glucose
The threshold concentrations of glucose in both the Japanese arable and forest soils were lower than the concentrationin the Kazakh forest soil; that is, the structural compounds in the Japanese arable and forest soils were synthesized at lower concentrations of added glucose than in the Kazakh forest soil. To examine the synthesis of structural compounds, the growth characteristics of the microbial biomass after glucose addition were estimated using the dynamics of respiration rates over time after the addition of 1000 μg C g−1 soil of glucose to the Japanese arable soil and 4000 μg C g−1 soil glucose to the Japanese and Kazakh forest soils. To compare the three soils, the respiration rates were divided by the biomass C (). To provide growth characteristics of the biomass, the substrate-induced respiration rate per unit of biomass C, the specific respiration increment and the lag period were estimated according to the method of CitationSawada et al. (2009) ().
Figure 5 Respiration rates divided by biomass C as a function of time after the addition of the highest concentrations of glucose. Error bars indicate standard errors (n = 3). Data for the Kazakh soil were obtained by recalculating the respiration rate after the addition of the highest concentration of glucose in in Sawada et al. (2008).
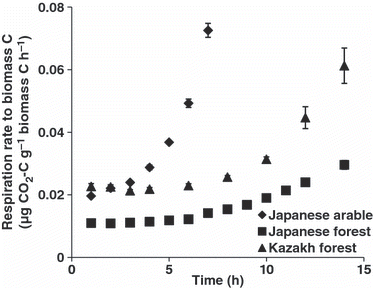
Comparison of the moderately and strongly acidic forest soils shows that, although respiration rates for the Japanese forest soil increased similarly to those in the Kazakh forest soil, the substrate-induced respiration rate per unit of biomass C for the Japanese forest soil was lower than that for the Kazakh forest soil (; ). Therefore, structural compounds in the microbial biomass of the Japanese forest soil were synthesized when lower concentrations of glucose were added, and thus the threshold concentration of glucose in the Japanese forest soil was lower than that in the Kazakh forest soil. CitationAnderson and Joergensen (1997) and CitationSawada et al. (2009) also reported that the ratios of substrate-induced respiration rate to chloroform-labile C were significantly correlated with soil pH, with lower pH corresponding to a lower ratio of substrate-induced respiration rate to biomass C. Therefore, a lower ratio of the substrate-induced respiration rate to biomass C caused a lower threshold concentration of glucose in acid-stressed soils compared with the non-stressed soils.
Soil disturbance from cultivation also considerably modifies the microbial response. shows that respiration rates for the Japanese arable soil increased faster than those for both forest soils. shows that the specific respiration increments for the Japanese arable soil were higher than those for the forest soils, and that the lag period was also shorter in the former than in the latter. Thus, structural compounds in the microbial biomass of the Japanese arable soil were synthesized faster than in the forest soils. CitationSawada et al. (2009) also reported that the lag periods in six disturbed soils were significantly shorter than those in six non-disturbed soils, although the specific respiration increments in the disturbed soils were not significantly different from those in natural undisturbed soils. Therefore, it is considered that the shorter lag period observed in the arable soil in the present study will be applicable to different disturbed soils and will cause lower threshold concentrations of glucose in disturbed soils compared with non-disturbed soils; however, the number of samples in CitationSawada et al. (2009) was too limited to comprehensively elucidate the process.
The results of the present study suggest that a higher proportion of substrates may be readily mineralized in acid and/or disturbed soils in cases where higher concentrations of substrates are occasionally supplied; for example, if soils experience temporal C ‘flushes’, such as those that can occur in the rhizosphere or in the vicinity of plant residues. The possibility of changing the efficiency of substrate C use by soil microorganisms in acid and/or disturbed soils should be taken into account when soil organic matter dynamics are described mechanistically based on the activity of soil microorganisms.
Table 3 Threshold concentrations of glucose and microbial properties of the soils studied
References
- Anderson , TH and Domsch , KH . 1993 . The metabolic quotient for CO2(qCO2) as a specific activity parameter to assess the effects of environmental conditions, such as pH, on the microbial biomass of forest soils . Soil Biol. Biochem. , 25 : 393 – 395 .
- Anderson , TH and Joergensen , RG . 1997 . Relationship between SIR and FE estimates of microbial biomass C in deciduous forest soils at different pH . Soil Biol. Biochem. , 29 : 1033 – 1042 .
- Bardgett , RD and Saggar , S . 1994 . Effects of heavy metal contamination on the short-term decomposition of labelled [14C] glucose in a pasture soil . Soil Biol. Biochem. , 26 : 727 – 733 .
- Bremer , E and Kuikman , P . 1994 . Microbial utilization of 14C[U] glucose in soil is affected by the concentration and timing of glucose . Soil Biol. Biochem. , 26 : 511 – 517 .
- Bremer , E and van Kessel , C . 1990 . Extractability of microbial 14C and 15N following addition of variable rates of labelled glucose and (NH4)2SO4to soil . Soil Biol. Biochem. , 22 : 707 – 713 .
- Chander , K and Brookes , PC . 1991 . Microbial biomass dynamics during the decomposition of glucose and maize in metal-contaminated and non-contaminated soils . Soil Biol. Biochem. , 23 : 917 – 925 .
- Chander , K and Joergensen , RG . 2002 . Decomposition of 14C labelled glucose in a Pb-contaminated soil remediated with synthetic zeolite and other amendments . Soil Biol. Biochem. , 34 : 643 – 649 .
- Dahlin , S and Witter , E . 1998 . Can the low microbial biomass C-to-organic C ratio in an acid and a metal contaminated soil be explained by differences in the substrate utilization efficiency and maintenance requirements? . Soil Biol. Biochem. , 30 : 633 – 641 .
- Fritze , H , Smolander , A , Levula , T , Kitunen , V and Mälkönen , E . 1994 . Wood-ash fertilization and fire treatments in a Scots pine forest stand: Effects on the organic layer, microbial biomass, and microbial activity . Biol. Fertil. Soils , 17 : 57 – 63 .
- Hill , PW , Farrar , JF and Jones , DL . 2008 . Decoupling of microbial glucose uptake and mineralization in soil . Soil Biol. Biochem. , 40 : 616 – 624 .
- Jones , DL and Darrah , PR . 1996 . Re-sorption of organic compounds by roots of Zea maysL. and its consequences in the rhizosphere . Plant Soil , 178 : 153 – 160 .
- Jones , DL and Edwards , AC . 1998 . Influence of sorption on the biological utilization of two simple carbon substrates . Soil Biol. Biochem. , 30 : 1895 – 1902 .
- Killham , K . 1985 . A physiological determination of the impact of environmental stress on the activity of microbial biomass . Environ. Pollut. (A) , 38 : 283 – 294 .
- Marstorp , H and Witter , E . 1999 . Extractable dsDNA and product formation as measures of microbial growth in soil upon substrate addition . Soil Biol. Biochem. , 31 : 1443 – 1453 .
- Nguyen , C and Guckert , A . 2001 . Short-term utilisation of 14C-[U]glucose by soil microorganisms in relation to carbon availability . Soil Biol. Biochem. , 33 : 53 – 60 .
- Nguyen , C and Henry , F . 2002 . A carbon–14–glucose assay to compare microbial activity between rhizosphere samples . Biol. Fertil. Soils , 35 : 270 – 276 .
- Nordgren , A , Bååth , E and Söderström , B . 1988 . Evaluation of soil respiration characteristics to assess heavy metal effects on soil microorganisms using glutamic acid as a substrate . Soil Biol. Biochem. , 20 : 949 – 954 .
- Sawada , K , Funakawa , S and Kosaki , T . 2008 . Soil microorganisms have a threshold concentration of glucose to increase the ratio of respiration to assimilation . Soil Sci. Plant Nutr. , 54 : 216 – 223 .
- Sawada , K , Funakawa , S and Kosaki , T . 2009 . Different effects of pH on microbial biomass carbon and metabolic quotients by fumigation extraction and substrate induced respiration methods in soils under different climatic conditions . Soil Sci. Plant Nutr. , 55 : 363 – 374 .
- Schneckenberger , K , Demin , D , Stahr , K and Kuzyakov , Y . 2008 . Microbial utilization and mineralization of [14C] glucose added in six orders of concentration to soil . Soil Biol. Biochem. , 40 : 1981 – 1988 .
- Scow , KM . 1997 . “ Soil microbial communities and carbon flow in agroecosystems ” . In Ecology in Agriculture , Edited by: Jackson , LE . 367 – 413 . London : Academic Press .
- Stenström , J , Svensson , K and Johansson , M . 2001 . Reversible transition between active and dormant microbial states in soil . FEMS Microbiol. Ecol. , 36 : 93 – 104 .
- Tsai , CS , Killham , K and Cresser , MS . 1997 . Dynamic response of microbial biomass, respiration rate and ATP to glucose addition . Soil Biol. Biochem. , 29 : 1249 – 1256 .
- Vance , ED , Brookes , PC and Jenkinson , DS . 1987 . An extraction method for measuring soil microbial biomass C . Soil Biol. Biochem. , 19 : 703 – 707 .
- Wardle , DA and Ghani , A . 1995 . A critique of the microbial metabolic quotient (qCO2) as a bioindicator of disturbance and ecosystem development . Soil Biol. Biochem. , 27 : 1601 – 1610 .
- Witter , E and Dahlin , S . 1995 . Microbial utilization of [U-14C]-labelled straw and [U-13C]-labelled glucose in soils of contrasting pH and metal status . Soil Biol. Biochem. , 27 : 1507 – 1516 .
- Witter , E and Kanal , A . 1998 . Characteristics of the soil microbial biomass in soils from a long–term field experiment with different level of C input . Appl. soil Ecol. , 10 : 37 – 49 .
- Wu , J , Joergensen , RG , Pommerening , B , Chaussod , R and Brookes , PC . 1990 . Measurement of soil microbial biomass C by fumigation-extraction—an automated procedure . Soil Biol. Biochem. , 22 : 1167 – 1169 .
- Xu , X , Han , L , Wang , Y and Inubushi , K . 2007 . Influence of vegetation types and soil properties on microbial biomass carbon and metabolic quotients in temperate volcanic and tropical forest soils . Soil Sci. Plant Nutr. , 53 : 430 – 440 .