Abstract
Rice paddy soil has been shown to have strong denitrifying activity. However, the microbial populations responsible for the denitrification in various rice paddy soils are not well known. In the present study, key denitrifiers were identified in six rice paddy soils by comparing the bacterial community structures in soils with strong denitrifying activity with those in soils without the activity. The effect of crop rotation on the denitrifier populations was also examined. Our study revealed three notable findings: (1) community structures of total bacteria were similar among the six rice paddy soils with different soil characteristics, (2) the populations that responded to denitrification-inducing conditions differed with soil type (Andosol, Gley soil or Gray lowland soil), (3) the populations that responded to denitrification-inducing conditions were similar within each type of soil, regardless of the cropping system (e.g. continuous or rotation).
Introduction
Denitrification is a microbial respiratory process in which nitrogen oxides (NO3 − and NO2 −) are reduced stepwise to gaseous end products (NO, N2O and N2) (CitationPhilippot et al. 2007). Nitrous oxide (N2O) is considered to be one of the major greenhouse gases (CitationPhilippot et al. 2007) and a most significant threat to ozone layer destruction (CitationRavishankara et al. 2009). Nitrous oxide is frequently produced as an end product of denitrification in upland crop fields; whereas little N2O is emitted from rice paddy soils (CitationAkiyama et al. 2006). The latter most likely occurs because of complete nitrate/nitrite reduction to N2, as rice paddy soil is known to have strong denitrifying activity (CitationNishimura et al. 2004).
Recently in Japan, large areas of rice paddy fields have been restructured for efficient land and water management. This has been done in response to decreases in the population of farmers and in rice consumption in Japan. As restructured rice paddy fields drain water more efficiently, upland crops such as soybean can be grown on drained rice paddy fields. To adjust for the overproduction of rice, rotational cropping systems (e.g. 2 years of rice cropping followed by 2 years of soybean cropping) have been introduced to the restructured rice paddy fields (CitationNishimura et al. 2005). As a result, 84% of the soybean cultivation area in Japan was drained rice paddy soils in 2002 (CitationNishimura et al. 2005). Although emission of N2O has been observed on upland crops grown on drained rice paddy fields (CitationNishimura et al. 2005), it is not clear if the denitrifier populations have changed with the change in the cropping system.
Various bacteria, as well as some archaea and fungi, have denitrifying ability (CitationHayatsu et al. 2008). Previous culture-independent studies have revealed that bacteria belonging to Burkholderiales and Rhodocyclales might be involved in denitrification in rice paddy soil collected from Nishitokyo City, Tokyo (CitationIshii et al. 2009b; CitationSaito et al. 2008; CitationYoshida et al. 2009). However, it is unclear if these bacteria are also involved in denitrification in rice paddy soils collected from other areas because bacterial community structures might differ with location (CitationSuzuki et al. 2009). CitationIshii et al. (2009b) showed that key denitrifiers can be identified by comparing community structures in soils with strong denitrifying activity with those in soils without denitrifying activity. The polymerase chain reaction-denaturing gradient gel electrophoresis (PCR-DGGE) technique has been shown to be useful in detecting populations responsive to specific conditions (CitationIshii et al. 2009a,b; CitationMorimoto et al. 2008).
Consequently, the objectives of the present study were: (1) to characterize the bacterial communities of six rice paddy soils using PCR-DGGE analysis, (2) to identify key denitrifiers in these rice paddy soils by comparative analysis, (3) to examine the impact of a rotational cropping system on denitrifier populations.
Materials and methods
Soil samples
Sampling sites were located at the Kumamoto Prefectural Agricultural Research Center (32º89′N, 130º77′E), the Niigata Agricultural Research Institute (37º20′N, 138º45′E) and the Yamagata General Agricultural Research Center (38º25N, 140º24′E). At each site, two soil samples were collected from the top 10 cm of the rice field with different cropping systems: continuous rice cropping and rice–soybean rotation systems. The latter started in 2006 as a 4-year crop rotation system, including 2 years each of rice and soybean cultivations. Soybean and rice were grown in 2006–2007 and 2008–2009, respectively. Until 2005, rice had been grown in these fields. Sampling was done in late April to early May (before water irrigation) in 2008. Soil samples were sieved through 2 mm mesh and stored at 4°C in the dark until required (∼1 month). The physicochemical characteristics of the soil samples are shown in .
Table 1 Physicochemical characteristics of the soil samples
Soil microcosm
A previously established reproducible soil microcosm setup (CitationIshii et al. 2009b; CitationSaito et al. 2008) was used in the present study. In brief, 1 g of fresh soil was placed in a 10-mL glass serum vial (Nichiden-Rika Glass, Kobe, Japan) and submerged in sterile water for 1 week at 30°C (pre-incubation) to simulate the water irrigation process. After pre-incubation, 1.8 mL of the upper soil-free water was removed and 0.1 mg N-nitrate and/or 0.5 mg C-succinate was added. The vial was anaerobically incubated with Ar–C2H2 (90:10) gas at 30°C for 24 h. For each soil, three treatments were examined. In the NS treatment the vials were incubated with added nitrate and succinate, whereas in the SU treatment the vials were incubated with only added succinate. In the BA treatment the vials were not supplemented with any substrate and were not incubated. Three replicate vials were prepared for each treatment. The production of CO2 and N2O was measured after 24-h incubation in each vial by using gas chromatography as described previously (CitationSaito et al. 2008). Incubation with nitrate and succinate has been shown to greatly enhance denitrification activity (CitationSaito et al. 2008). Previous studies have also shown that succinate can be used by various denitrifiers, but is rarely used for other functions, such as fermentation, metal reductions and dissimilatory nitrate reduction to ammonium (DNRA), within 24-h incubation in the presence of nitrate (CitationIshii et al. 2009b; CitationSaito et al. 2008). In addition to denitrification, nitrate respiration, which converts NO3 − to NO2 − and does not produce gaseous end products, may also occur under the conditions used (CitationIshii et al. 2009b).
DNA extraction, PCR-DGGE and sequencing analyses
The DNA was extracted from the soil (0.8 g) by using an ISOIL for Beads Beating kit (Nippon Gene, Tokyo, Japan) as previously described (CitationIshii et al. 2009b). The PCR was carried out with 357F-GC and 520R primers (CitationIshii et al. 2009b; CitationMuyzer et al. 1993) to amplify the V3 region of the 16S rRNA gene. Aliquots of 750 ng of the PCR products were loaded onto 8% acrylamide gel with 35–65% denaturant concentrations and electrophoresis was carried out at 60 V for 800 min. The gel was stained with SYBR Green II (Invitrogen, Carlsbad, CA, USA) and the gel image was captured using a Printgraph GX CCD camera (ATTO, Tokyo, Japan). Principal component analysis (PCA) was carried out based on the digitized DGGE banding profiles by using R software version 2.9.0. (http://www.r-project.org) as previously described (CitationIshii et al. 2009a).
The DGGE bands that were unique to the samples amended with nitrate and succinate were excised from the gel by using a razor blade. The DNA was eluted from the gel and used for PCR with 357F and 520R primers (CitationMuyzer et al. 1993; CitationOtsuka et al. 2008). The PCR products were ligated into the pGEM-T Easy vector system (Promega, Madison, WI, USA) and transformed into Escherichia coli JM101 competent cells according to the manufacturer’s instructions. Cloned insert DNA were amplified by PCR with vector primers T7-1 and SP6 and used for the sequencing reaction as described previously (CitationSaito et al. 2008). Four clones per DGGE band were sequenced and their taxonomic assignment was examined using Naïve Bayesian Classifier version 2.0 (CitationWang et al. 2007). Nucleotide sequences of multiple clones were aligned and a neighbor-joining tree was constructed using the ClustalW program as previously described (CitationIshii et al. 2009b). The nucleotide sequences determined in the present study were deposited into DNA Data Bank of Japan under accession numbers AB535663–AB535679.
Results and discussion
The DGGE banding profiles were similar within the replicates (data not shown), as found in a previous study (CitationIshii et al. 2009b). Therefore, we combined each set of replicate DNA samples to compare the bacterial community structures of the six soil samples with the three treatments (total of 18 DNA samples) in a single denaturing gradient gel. The banding profiles showed that the bacterial community structures were similar among the six soils in the BA and SU treatments (). These samples were also tightly clustered in a PCA plot (), suggesting that the bacterial community structures were similar under paddy conditions, regardless of soil type. Similar results were obtained from several field rice paddy soils using DGGE analysis (CitationHiramatsu et al. 2007).
Samples amended with nitrate and succinate (NS treatment) had different community structures than the BA and SU treatments (), suggesting that several groups of bacteria may have been enriched by the NS treatment. Denitrification activity, as measured by the accumulation of N2O gas under the presence of 10% C2H2, was detected in these NS-treated samples (data not shown). When vials were incubated with 100% Ar gas, instead of Ar–C2H2 (90:10) gas, no N2O accumulated (data not shown), suggesting that denitrifiers in the NS-treated samples reduced NO3 − completely to N2 gas. These results indicate that the bacteria represented by the distinct DGGE bands (shown by arrows in ) may have increased their population by performing complete denitrification. Interestingly, these enriched populations in the NS-treated samples differed between the soils, although the background bacterial community structures were similar. Based on the sequence analysis of the excised bands, clones related to the genus Chromobacterium were common in the NS-treated Kumamoto and Niigata soils; whereas clones related to the genus Ammoniphilus were common in the NS-treated Niigata and Yamagata soils (). Clones obtained from different soils were phylogenetically closely related, and some had 100% identical sequences (). In contrast to Chromobacterium and Ammoniphilus, clones related to the order Rhodocyclales (e.g. Dechloromonas sp. and Azoarcus sp.) were only seen in Kumamoto soil. Previous studies have shown that members of Burkholderiales and Rhodocyclales respond to the addition of nitrate and succinate in rice paddy soil collected from Tanashi, Tokyo (CitationIshii et al. 2009b; CitationSaito et al. 2008). As both the Tanashi and Kumamoto soils were classified as Andosol, the appearance of Rhodocyclales bacteria in response to additions of nitrate and succinate may be related to the characteristics of the Andosol. Similar to our study, CitationSuzuki et al. (2009) reported that bacterial communities in soil grown with upland crops were more dependent on soil type (e.g. Andosol or grey lowland soil) than fertilizer type (e.g. chemical fertilizer or manure). As Andosol is derived from volcanic ash, this soil is well-drained compared with soils of alluvial origin (e.g. Gley soil and Gray-lowland soil) (CitationNishimura et al. 2005). In addition, Andosol is usually rich in organic matter, as is the case with the Kumamoto soil. These Andosol characteristics may have selected Rhodocyclales, which is a facultative–anaerobic and metabolically versatile bacteria (some Rhodocyclales can degrade recalcitrant organic matter) (CitationLoy et al. 2005), as major denitrifiers responsive to the NS treatment. More Andosol samples need to be examined to draw further conclusions about the appearance of Rhodocyclales bacteria. Collectively, these results indicate that Chromobacterium sp. and Ammoniphilus sp. are common denitrifiers in several rice paddy soils, whereas some other denitrifiers (e.g. Dechloromonas sp. and Azoarcus sp.) are more specific to a particular soil, under the conditions used in the present study. As 16S rRNA gene-based analysis alone cannot identify the ecological role of bacteria, isolation of these bacteria is necessary to confirm that they perform denitrification for growth.
Figure 1 Bacterial community structures assessed by denaturing gradient gel electrophoresis (DGGE) analysis. (a) The DGGE banding profiles of the six soil samples and three treatments. Lanes 1–3, soil sample collected from the continuous rice field from Niigata (NC); lanes 4–6, soil sample collected from the rice–soybean rotation field from Niigata (NR); lanes 7–9, soil sample collected from the continuous rice field from Yamagata (YC); lanes 10–12, soil sample collected from the rice–soybean rotation field from Yamagata (YR); lanes 13–15, soil sample collected from the continuous rice field from Kumamoto (KC); lanes 16–18, soil sample collected from the rice–soybean rotation field from Kumamoto (KR); BA, samples without incubation; SU, samples incubated with added succinate; NS, samples incubated with added nitrate and succinate. (b) Principal component analysis (PCA) plot based on the DGGE profile. The normalized location and intensity of each DGGE band were used in the PCA analysis. The numbers in the plot correspond to the lane numbers in (a). The numbers in parentheses are the percentages of variation explained by each component.
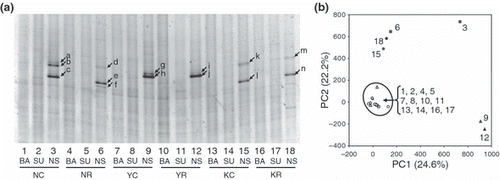
Table 2 Taxonomic assignment of the clones obtained from the excised denaturing gradient gel electrophoresis bands
Figure 2 Neighbor-joining tree showing the phylogenetic relationship between the clones obtained from the excised denaturing gradient gel electrophoresis band shown in Fig. 1. Bootstrap values (%) were generated from 1,000 replicates and only values >70% are shown. α, Alphaproteobacteria; β, Betaproteobacteria; B, Bacilli.
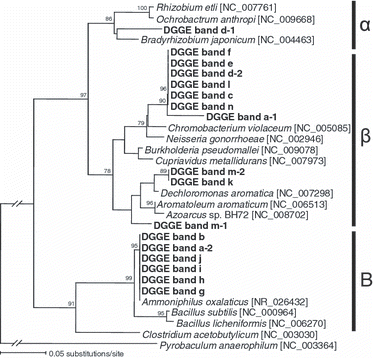
Our results also suggest that the cropping system (e.g. continuous or rotation) did not substantially influence the denitrifier populations. Similar populations responded to the NS treatment in the continuous and rotational cropping systems (,), indicating that the same groups of bacteria may carry out denitrification under the conditions used in the present study. This result implies that the denitrifiers acting under paddy conditions may have survived in the following 2 years of soybean cultivation (rotational cropping) and re-emerged when conditions become favorable. Although denitrification and the resulting N2O emission could occur during soybean cultivation in fields converted from rice paddy (CitationNishimura et al. 2005), it is not clear from our study if N2-generating denitrifiers under rice paddy conditions can produce N2O under well-drained conditions. This needs to be clarified in a future field study.
In conclusion, our results suggest that the fundamental bacterial populations were similar among the rice paddy soils examined in the present study, but that the denitrifier populations might be affected by soil type. In addition, our results suggest that the impact of the cropping system on denitrifier populations is minimal. These results are important when establishing strategies to mitigate N2O emission from upland crop fields converted from rice paddy. As our results were obtained using a soil microcosm experiment, further study is necessary to investigate whether the “key denitrifiers” identified in the present study really perform denitrification in field rice paddy soils by using rRNA-based and/or mRNA-based analysis.
Acknowledgments
We thank Shigeto Sudo and Kazuyuki Yagi (National Institute for Agro-Environmental Sciences), Hiroyuki Mizukami and Noriaki Gunjikake (Kumamoto Prefectural Agricultural Research Center), Yuichiro Furukawa and Yutaka Shiratori (Niigata Agricultural Research Institute) and Masamichi Adachi, Yuichiro Hurukawa, Hiroshi Saito, Hiroyuki Shiono and Katsuki Kumagai (Yamagata General Agricultural Research Center) for their help in collecting soil samples. This work was supported by the Program for the Promotion of Basic Research Activities for Innovative Biosciences (PROBRAIN) from the Bio-oriented Technology Research Advancement Institution, Tokyo, Japan. Additional financial support was provided from a Grand-in-Aid (Soil eDNA) from the Ministry of Agricultural, Forestry and Fisheries of Japan.
References
- Akiyama , H , Yan , X and Yagi , K . 2006 . Estimations of emission factors for fertilizer-induced direct N2O emissions from agricultural soils in Japan: summary of available data . Soil Sci. Plant Nutr. , 52 : 774 – 787 .
- Hayatsu , M , Tago , K and Saito , M . 2008 . Various players in the nitrogen cycle: diversity and functions of the microorganisms involved in nitrification and denitrification . Soil Sci. Plant Nutr. , 54 : 33 – 45 .
- Hiramatsu , M , Fujii , T and Watanabe , Ket al . Bacterial communities in rice paddy soils with long-term application of organic fertilizers . as analyzed by PCR-DGGEAbstracts of the Annual Meeting . Vol. 53 , pp. 38 Japanese Society of Soil Science and Plant Nutrition . (in Japanese
- Ishii , S , Kadota , K and Senoo , K . 2009a . Application of a clustering-based peak alignment algorithm to analyze various DNA fingerprinting data . J. Microbiol. Meth. , 78 : 344 – 350 .
- Ishii , S , Yamamoto , M Kikuchi , M . 2009b . Microbial populations responsive to denitrification-inducing conditions in rice paddy soil, as revealed by comparative 16S rRNA gene analysis . Appl. Environ. Microbiol. , 75 : 7070 – 7078 .
- Loy , A , Schulz , C Lücker , S . 2005 . 16S rRNA gene-based oligonucleotide microarray for environmental monitoring of the Betaproteobacterial order “Rhodocyclales” . Appl. Environ. Microbiol. , 71 : 1373 – 1386 .
- Morimoto , S , Ogawa , N , Hasebe , A and Fujii , T . 2008 . Isolation of effective 3-chlorobenzoate-degraders in soil using community analyses by PCR-DGGE . Microbes Environ. , 23 : 285 – 292 .
- Muyzer , G , De Waal , EC and Uitterlinden , AG . 1993 . Profiling of complex microbial populations by denaturing gradient gel electrophoresis analysis of polymerase chain reaction-amplified genes coding for 16S rRNA . Appl. Environ. Microbiol. , 59 : 695 – 700 .
- Nishimura , S , Sawamoto , T , Akiyama , H , Sudo , S , Cheng , W and Yagi , K . 2005 . Continuous, automated nitrous oxide measurements from paddy soils converted to upland crops . Soil Sci. Soc. Am. J. , 69 : 1977 – 1986 .
- Nishimura , S , Sawamoto , T , Akiyama , H , Sudo , S and Yagi , K . 2004 . Methane and nitrous oxide emissions from a paddy field with Japanese conventional water management and fertilizer application . Glob. Biogeochem. Cycles , 18 : GB2017
- Otsuka , S , Sudiana , I Komori , A . 2008 . Community structure of soil bacteria in a tropical rainforest several years after fire . Microbes Environ. , 23 : 49 – 56 .
- Philippot , L , Hallin , S and Schloter , M . 2007 . Ecology of denitrifying prokaryotes in agricultural soil . Adv. Agron. , 96 : 249 – 305 .
- Ravishankara , AR , Daniel , JS and Portmann , RW . 2009 . Nitrous oxide (N2O): the dominant ozone-depleting substance emitted in the 21st century . Science , 326 : 123 – 125 .
- Saito , T , Ishii , S , Otsuka , S , Nishiyama , M and Senoo , K . 2008 . Identification of novel Betaproteobacteria in a succinate-assimilating population in denitrifying rice paddy soil by using stable isotope probing . Microbes Environ. , 23 : 192 – 200 .
- Suzuki , C , Nagaoka , K , Shimada , A and Takenaka , M . 2009 . Bacterial communities are more dependent on soil type than fertilizer type, but the reverse is true for fungal communities . Soil Sci. Plant Nutr. , 55 : 80 – 90 .
- Wang , Q , Garrity , GM , Tiedje , JM and Cole , JR . 2007 . Naïve Bayesian classifier for rapid assignment of rRNA sequences into the new bacterial taxonomy . Appl. Environ. Microbiol. , 73 : 5261 – 5267 .
- Yoshida , M , Ishii , S , Otsuka , S and Senoo , K . 2009 . Temporal shifts in diversity and quantity of nirSand nirKin a rice paddy field soil . Soil Biol. Biochem. , 41 : 2044 – 2051 .