Abstract
Using granulocyte-macrophage colony stimulating factor, interleukin4 and tumor necrosis factor α, we generated dendritic cells (DCs) from mononuclear cells isolated from the peripheral blood (PB) of eight patients with acute lymphoblastic leukemia (ALL), who were in complete remission (CR), and pulsed these DCs with leukemic cell lysates. Specific cytotoxicity assays were performed by incubation of effector cells (lymphocytes generated from cryopreserved mononuclear cells isolated in CR state of ALL) and targets (cryopreserved leukemic cells at diagnosis). Patients showing decreased cytotoxicity had poorer clinical courses. When we measured lymphocyte subsets, we found positive correlations between cytotoxicity levels and the proportions of T lymphocytes and CD8+ T lymphocytes, but negative correlations between cytotoxicity levels and the proportions of NK cells and regulatory T lymphocytes. In conclusion, we show here that leukemia-specific autologous DCs can be generated from the PB of ALL patients in CR, that the incubation of these DCs with leukemic cell lysates can generate lymphocytes potentiated against leukemic cells, and that relationships are evident among all of cytotoxicity, lymphocyte subsets, and patient prognosis.
Introduction
Dendritic cells (DCs) are the most potent form of antigen-presenting cells. They activate T lymphocytes in a two-step process. In the first step, T-cell receptors (TCRs) on T cells recognize and bind to human leukocyte antigen (HLA)/peptide complexes on the surface of DCs. This is followed by the binding of B7 on DCs to CD28 on T cells, resulting in a co-stimulatory signal that causes T-cell activation and proliferation. Leukemic blasts produce cytokines such as tumor growth factor-beta (TGFβ) and interleukin (IL)10, generating a microenvironment favourable for survival.Citation1 In such a microenvironment, DCs are inactivated and suppressed.Citation2 As down-regulated DCs have been found in patients with acute myeloid leukemia (AML),Citation3 it is important to generate a sufficient number of DCs specific for leukemic blasts, by incubating immature DCs with peptides from leukemia-specific antigens, such as overexpressed MUCI,Citation4 WTI,Citation5 and PML-RARa.Citation6 DCs can also be stimulated with antigens that are not leukemia-specific, such as leukemic cell lysates,Citation7 apoptotic material,Citation8 and acid-eluted peptides.Citation9 Incubation of DCs with both AML tumor lysate and AML-specific tumor antigen was more effective than was incubation with either alone,Citation10 and DCs stimulated by leukemic antigen were shown to be the most potent primers of T cells.Citation11
DCs can be differentiated from leukemic cells by virtue of production of specific cytokines such as GM-CSF, TNFα, IL3, FL, SCF, and CD40L.Citation12–Citation14 In 30–40% of patients, however, DCs could not be differentiated and induced DCs had low levels of co-stimulatory molecules.Citation15 Moreover, these leukemic cell-induced DCs were short-lived and may have been contaminated with other cell types. CD14+ monocytes have also been used to generate DCs, which were found to exhibit a more homogeneous mature DC phenotype than did leukemic blast-induced DCs.Citation16
In the present study, we utilized cytokines to induce DCs from mononuclear cells (MNCs) separated from the peripheral blood (PB) of patients with acute lymphoblastic leukemia (ALL), who were in complete remission (CR). These DCs were incubated with lysates of leukemic cells obtained at diagnosis and used to induce leukemia-specific cytotoxic T-lymphocytes from MNCs isolated from PB of ALL patients in CR. Finally, we measured the cytotoxic activity of such T cells ().
Figure 1. Schematic diagram of the method employed. Leukemic cells isolated from bone marrow aspirates (BM) or peripheral blood (PB) at diagnosis were used as target cells and to prepare leukemic cell lysates. When complete remission (CR) was achieved after chemotherapy, mononuclear cells (MNCs) were isolated from PB and used to generate dendritic cells (DCs) and cytotoxic lymphocytes. Leukaemic cell lysates were used to pulse DCs. MNCs from PB of patients in CR were co-cultured with pulsed DCs and IL2. Effector cells (cytotoxic T lymphocytes obtained from the above co-cultures of MNCs and pulsed DCs) were co-cultured with target cells (leukemic cells obtained from patients at diagnosis), and cytotoxicity was evaluated using a lactate dehydrogenase release assay.
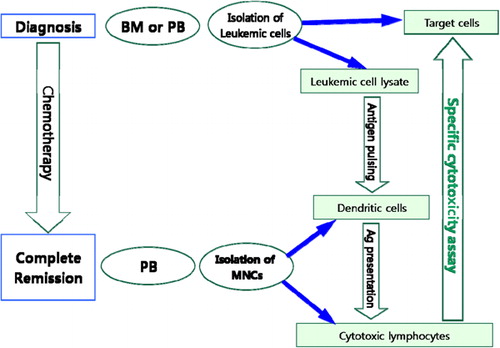
Materials and methods
Patients
Our patients consisted of eight newly diagnosed ALL patients, including seven with chromosomal abnormalities, who achieved CR after chemotherapy. During the course of this investigation, two patients relapsed and one died. Patient characteristics are summarized in .
Table 1. Clinical characteristics of patients with ALL
Upon diagnosis, bone marrow (BM) aspirates were collected from each patient into preservative-free heparinized tubes. After chemotherapy, when patients were in CR, samples of 10–15 mL PB were collected into preservative-free heparinized tubes. This study was approved by the institutional review board of Asan Medical Center and informed consent was obtained from all patients or their parents according to the Declaration of Helsinki.
Collection and cryopreservation of leukemic cells
Leukemic cells were isolated from BM aspirates at diagnosis by Histopaque-1077 density gradient (Sigma, St Louis, MO, USA) centrifugation for 30 minutes at room temperature. The MNCs were washed with RPMI 1640 medium (GIBCO, Grand Island, NY, USA) and cryopreserved at −70°C, in aliquots of ⩽1·5 mL of freezing medium containing 15% (v/v) fetal bovine serum (FBS, GIBCO) and 7·5% (v/v) DMSO (Sigma).
Collection of MNCs from patients in CR
MNCs were isolated from the heparinized blood of patients who achieved CR using Histopaque-1077 density gradient centrifugation for 30 minutes at room temperature, and washed in RPMI 1640 medium (GIBCO). Some MNCs were used for production of DCs and others were cryopreserved as above to produce potentiated lymphocytes.
Lymphocyte subset tests
Lymphocyte subsets in the PB of patients who achieved CR were analyzed by multi-color flow cytometry (FACScanTM flow-cytometer running CELLQuestTM software [Becton Dickinson]) using a combination of monoclonal antibodies directed against CD3-FITC/CD4-PE, CD3-FITC/CD8-PE, CD19-FITC, and CD3-FITC/CD16/56-PE (Becton Dickinson, San Jose, CA, USA), according to the manufacturer’s instructions.
Production of DCs
PB MNCs (1×106 cells/mL) were cultured for 8 days in 100-mm-diameter culture dishes (Nunc, Roskilde, Denmark) in RPMI 1640 supplemented with 1% (v/v) antibiotic-antimycotic solution (10 000 units/mL penicillin G sodium, 10 000 μg/mL streptomycin sulfate, 23 μg/mL amphotericin B, in 0·85% [w/v] saline), 10% (v/v) heat-inactivated FBS, 30 ng/mL recombinant granulocyte macrophage colony-stimulating factor (GM-CSF, R&D Systems, Abingdon, UK), and 20 ng/mL IL4 (R&D Systems), in a total volume of 10 mL. The medium was changed on day 3, and to the above medium plus 30 ng/mL TNFα on day 6, to induce DC maturation.
In a pilot study, we induced DCs from MNCs isolated from PB of normal volunteers and analyzed the extent of maturation of DCs on days 6 and 8 using monoclonal antibodies directed against CD83-FITC, CD1a-PE, CD86-PE, and HLA-DR-PerCP (Becton Dickinson).
Leukemic cell lysate pulsing of DCs
To prepare leukemic cell lysates, cryopreserved leukemic blasts obtained from patients at diagnosis were washed, resuspended in RPMI 1640 medium, and distributed into cryovials 1·8 mL in volume, which were alternately immersed in liquid nitrogen and a 37°C water bath for 10 minutes each time, for four cycles. The contents of each vial were centrifuged, and the supernatants were filtered through a 0·22-μm pore-sized syringe filter (Millipore, Bedford, MA, USA). Leukaemic cell lysates (400 μg/mL in complete medium) were added to DCs on day 8 of DC culture, and the DCs were incubated for one further day.
Priming of lymphocytes with DCs
Cryopreserved MNCs from PB of ALL patients in CR were thawed in a 37°C water bath, washed, resuspended in complete medium to which 400 IU/mL IL2 was also added, and co-cultured with DCs pulsed with leukemic cell lysate for 10 days. The culture medium was changed every 5 days, with addition of fresh IL2.
Lymphocyte cytotoxicity tests
Lymphocyte cytotoxicity was measured in vitro using a Cytotoxicity Detection KitPLUS (a lactate dehydrogenase [LDH] release assay) (Roche Applied Science, Mannheim, Germany),Citation17 as directed by the manufacturer. Primed lymphocytes, at 5×104/mL, 1×105/mL, or 2×105/mL were resuspended in RPMI medium containing 10% (v/v) FBS, and 50 μL aliquots were distributed into the wells of 96-microwell plates. Leukaemic blasts obtained from PB or BM aspirate at diagnosis were thawed in a 37°C water bath, and specimens showing >95% viability were included in the experiment. A 50 μL aliquot of leukemic blasts (1×104/mL) was added to each microwell containing primed lymphocytes, to yield final effector-target ratios of 5∶1, 10∶1, or 20∶1. Three types of control wells were employed, containing lymphocytes alone (to measure spontaneous release by effector cells), leukemic blasts alone (to measure spontaneous release by target cells), and target cells plus 5 μL lysis solution (to measure maximal release). The 96-microwell plates were incubated for 4 hours at 37°C in a 5% (v/v) CO2 incubator. LDH release was measured by adding 100 μL of reaction mixture to each well, incubating the plates for 10 minutes in the dark at room temperature, followed by addition of 50 μL reaction stop solution to each well. After 10 seconds of agitation, the absorbance of each well was measured at 490 nm and 650 nm using a microplate reader (Dynatech MR5000, Dynatech Laboratories Inc., VA, USA). All experiments were performed in triplicate. Cytotoxicity was calculated according to the formula.where E is LDH release by effector-target cell incubation, St is spontaneous release by target cells, Se is spontaneous release by effector cells, and M is maximal release by target cells.
Results
Induction of DCs from PB of normal volunteers
In a pilot study, we induced DCs from MNCs isolated from the PB of two normal volunteers and analyzed DC maturation on days 6 and 8 using monoclonal antibodies. After stimulation, the cells showed the immunophenotype characteristic of DCs ().
Table 2. Immunophenotypic changes during generation of dendritic cells in two normal volunteers
Cytotoxicity results
Cytotoxicity was tested using primed lymphocytes with leukemic cell lysate-pulsed DCs (effector cells) and leukemic blasts from patients (target cells), at effector-target cell ratios of 5∶1, 10∶1, and 20∶1. We found that cytotoxicity increased as the effector-target cell ratio rose (). Specimens with viability <95% were discarded.
Table 3. Cytotoxicity tests and patient characteristics
We compared the cytotoxicity against leukemic cells of unprimed effector cells and effector cells primed with leukemic cell lysate-pulsed DCs using cells from patients 6 and 8. The primed effectors from patient 6 displayed higher cytolytic activities (39·55%, 44·26%, and 49·39% at ratios of 5∶1, 10∶1 and 20∶1, respectively) against leukemic target cells (p = 0·002) than did unprimed effectors (20·3%, 22·84%, and 30·96% at ratios of 5∶1, 10∶1, and 20∶1, respectively). Primed effectors from patient 8, who had a poor clinical course, showed no difference in cytotoxicity compared to unprimed effectors, with both having cytotoxicity values <5% ().
Figure 2. Cytotoxicity against leukemic cells of unprimed effector cells and of effector cells primed with leukemic cell lysate-pulsed DCs from two patients with acute lymphoblastic leukemia. (a) Patient 6, (b) Patient 8. Leukemic cell lysate-pulsed DCs (▪) displayed higher cytotoxicity against leukemic target cells of patient 6 than did unprimed effector cells (•). In patient 8, who had a poor clinical course, there was no difference in cytotoxicity between unprimed and primed effector cells.
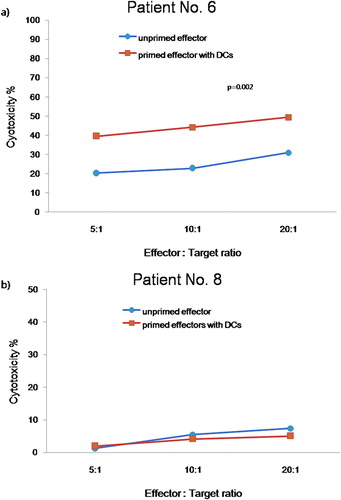
We found that cytotoxicity tended to increase as the effector/target cell ratio rose, except for cells from patient 2 (). Patients with poorer clinical courses (i.e., those who relapsed or died; patients 7 and 8) had lymphocytes of lower cytotoxicity, with no increase in cytotoxicity being evident as the effector/target cell ratio rose. Cytotoxicity was higher in patients with a normal karyotype and hyperdiploidy (patients 2, 3, and 4) than in other patients.
Figure 3. Cytotoxicity against leukemic cells of effector cells from patients with acute lymphoblastic leukemia. Cytotoxicity tended to increase as the effector/target cell ratio rose. Patients (7 and 8) with low cytotoxicity against their leukemic cells showed poor prognoses, as shown by relapse and death.
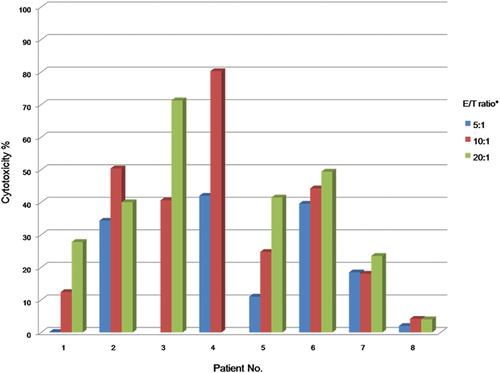
Lymphocyte subset tests
We observed an inverse relationship between cytotoxicity and the proportions of NK cells (r = −0·811, P = 0·047), and regulatory T lymphocytes (r = −0·500, P = 0·221), but positive relationships between cytotoxicity and the proportions of total T lymphocytes (r = 0·536, P = 0·189) and CD8+ T lymphocytes (r = 0·607, P = 0·137) (, ).
Figure 4. Correlation between cytotoxicity and proportions of lymphocyte subsets; (a) NK cells (r = −0·811, P = 0·047); (b) regulatory T lymphocytes (r = −0·500, P = 0·221); (c) total T lymphocytes (r = 0·536, P = 0·189), and (d) CD8+ T lymphocytes (r = 0·607, P = 0·137).
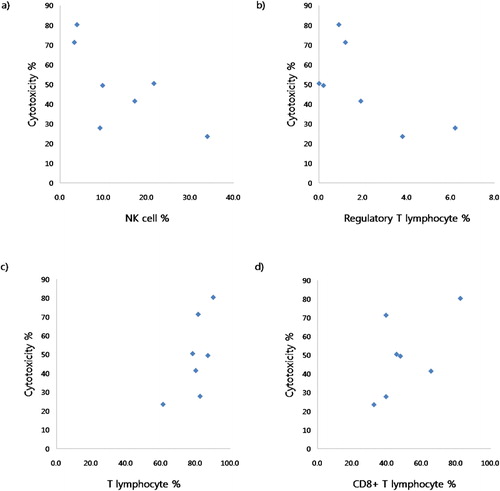
Table 4. Subset tests of lymphocytes in the peripheral blood of patients in complete remission
Discussion
We have shown here that DCs can be induced by cytokines from MNCs separated from PB of ALL patients in CR. Such DCs could be used to induce potentiated leukemia-specific cytotoxic lymphocytes, showing patient-specific cytotoxic activity.
DCs can be induced from normal CD34+ stem cells using GM-CSF and TNFα,Citation18 or GM-CSF, TNFα, and IL4.Citation19,Citation20 We used a combination of GM-CSF, TNFα and IL4 to induce DCs from MNCs. After stimulation, these cells showed the immunophenotype characteristic of mature DCs.
To induce anti-leukemic effects, DCs must present appropriate antigens to cytotoxic T lymphocytes. We used leukemic cell lysates as an antigen mixture to stimulate immature DCs. Such lysates, prepared using freeze–thaw cycles with removal of cell debris by centrifugation, contain several leukemia-specific antigens as well as antigens that may induce autoimmune reactions. In line with our method, othersCitation21 have used leukemic cell lysates to generate mature DCs specific for leukemic blasts.
Leukaemic cells used as target cells were separated from MNCs at diagnosis, cryopreserved, and thawed when patients achieved CR. After thawing of leukemic cells, we tested cell viability. We compared the cytotoxicity of primed (i.e., leukemic cell lysate-pulsed DCs) and unprimed effector cells against leukemic cells of patients 6 and 8. Leukaemic cell lysate-pulsed DCs from patient 6 displayed a higher cytolytic activity against leukemic target cells than did unprimed effector cells, a finding in agreement with data reported previously.Citation7,Citation9,Citation21–Citation23 However, primed and unprimed effector cells from patient 8 did not differ in cytotoxicity. These findings indicate that cytotoxicity tests using pulsed DCs may be useful before DC immunotherapy of ALL patients. Patients showing cytotoxicity differences between primed and unprimed effector cells would be candidates for immune cell therapy, whereas patients showing no differences in cytotoxicity would be excluded from immune cell therapy.
ALL relapses occurred in patients 7 and 8, and patient 8 died. Patient 7 showed a complex karyotype with t(8;14), and patient 8 had MLL gene rearrangement. In both patients, effector/target cell ratios had little effect on cytotoxicity. In contrast, patients who did not relapse showed relatively high cytotoxicity, with increases being observed as the effector/target ratio rose in patients 1, 3, 4, 5 and 6. Relatively high cytotoxicity was observed in patients 2 and 6. These results indicate that patients with low cytotoxicity had a poorer clinical course, whereas patients with high cytotoxicity had a good clinical course.
Regulatory T cells can control DC function by modulating surface antigen expression and cytokine secretion to regulate immune responses.Citation24,Citation25 We observed negative correlations between cytotoxicity and the proportions of NK cells (r = −0·811, P = 0·047) and regulatory T lymhocytes (r = −0·500, P = 0·221), but positive correlations between cytotoxicity and the proportions of T lymphocytes (r = 0·536, P = 0·189) and CD8+ T lymphocytes (r = 0·607, P = 0·137). The small number of patients might be the cause of less statistical significance (P values >0·05) in regulatory T cells, T lymphocytes and CD8+ T cells. Patients with increased proportions of CD8+ T lymphocytes showed elevated cytotoxicity and had a good prognosis. In contrast, patients with decreased cytotoxicity may have a higher proportion of activated regulatory T lymphocytes, thus inhibiting development of the antigen-presenting function of DCs. And patients with decreased cytotoxicity may have a higher proportion of NK cells to compensate for the reduced cytotoxic T-cell function induced by the drop in DC function, and the anti-leukemic function may be maintained by an increased proportion of NK cells. Reinforcing the potency of DCs by reducing the activity of regulatory T lymphocytes or employing cell therapy using NK cells may be another option in patients with decreased specific cytotoxicity against leukemic cells. We found that interactions among lymphocyte subsets were important, in that the proportions of lymphocytes in various subsets correlated better with cytotoxicity than did absolute cell numbers.
In conclusion, we have shown here that leukemia-specific autologous DCs may be generated using PB from ALL patients in CR and that pulsing of such DCs with leukemic cell lysates can generate lymphocytes potentiated against leukemic cells. The cytotoxicity of such lymphocytes tended to increase as the effector/target cell ratio rose. Patients with decreased cytotoxicity had poorer clinical courses. Moreover, we observed correlations between the degree of cytotoxicity and the proportions of lymphocyte subsets.
This study was supported by grant no. 06–179 from the Asan Institute for Life Science, Seoul, Korea.
References
- Buggins AG, Milojkovic D, Arno MJ, Lea NC, Mufti GJ, Thomas NS, et al.. Microenvironment produced by acute myeloid leukemia cells prevents T cell activation and proliferation by inhibition of NF-kappaB, c-Myc, and pRb pathways. J Immunol. 2001;167(10):6021–30.
- Yang L, Carbone DP. Tumor-host immune interactions and dendritic cell dysfunction. Adv Cancer Res. 2004;92:13–27.
- Mohty M, Jarrossay D, Lafage-Pochitaloff M, Zandotti C, Briere F, et al.. Circulating blood dendritic cells from myeloid leukemia patients display quantitative and cytogenetic abnormalities as well as functional impairment. Blood. 2001;98(13):3750–6.
- Brossart P, Schneider A, Dill P, Schammann T, Grunebach F, Wirths S, et al.. The epithelial tumor antigen MUC1 is expressed in hematological malignancies and is recognized by MUC1-specific cytotoxic T-lymphocytes. Cancer Res. 2001;61(18):6846–50.
- Gaiger A, Reese V, Disis ML, Cheever MA. Immunity to WT1 in the animal model and in patients with acute myeloid leukemia. Blood. 2000;96(4):1480–9.
- Osman Y, Takahashi M, Zheng Z, Toba K, Liu A, Furukawa T, et al.. Dendritic cells stimulate the expansion of PML-RAR alpha specific cytotoxic T-lymphocytes: its applicability for antileukemia immunotherapy. J Exp Clin Cancer Res. 1999;18(4):485–92.
- Weigel BJ, Panoskaltsis-Mortari A, Diers M, Garcia M, Lees C, Krieg AM, et al.. Dendritic cells pulsed or fused with AML cellular antigen provide comparable in vivo antitumor protective responses. Exp Hematol. 2006;34(10):1403–12.
- Spisek R, Chevallier P, Morineau N, Milpied N, Avet-Loiseau HHarousseau, et al.. Induction of leukemia-specific cytotoxic response by cross-presentation of late-apoptotic leukemic blasts by autologous dendritic cells of nonleukemic origin. Cancer Res. 2002;62(10):2861–8.
- Delluc S, Tourneur L, Fradelizi D, Rubio MT, Marchiol-Fournigault CChiocchia, et al.. DC-based vaccine loaded with acid-eluted peptides in acute myeloid leukemia: the importance of choosing the best elution method. Cancer Immunol Immunother. 2007;56(1):1–12.
- Decker WK, Xing D, Li S, Robinson SN, Yang H, Yao X, et al.. Double loading of dendritic cell MHC class I and MHC class II with an AML antigen repertoire enhances correlates of T-cell immunity in vitro via amplification of T-cell help. Vaccine. 2006;24(16):3203–16.
- Grabrucker C, Liepert A, Dreyig J, Kremser A, Kroell T, Freudenreich M, et al.. The quality and quantity of leukemia-derived dendritic cells from patients with acute myeloid leukemia and myelodysplastic syndrome are a predictive factor for the lytic potential of dendritic cells-primed leukemia-specific T cells. J Immunother. 2010;33(5):523–37.
- Kohler T, Plettig R, Wetzstein W, Schmitz M, Ritter M, Mohr B, Schaekel U, et al.. Cytokine-driven differentiation of blasts from patients with acute myelogenous and lymphoblastic leukemia into dendritic cells. Stem Cells. 2000;18(2):139–47.
- Mohty M, Isnardon D, Blaise D, Mozziconacci MJ, Lafage-Pochitaloff M, Briere F, Gastaut JA, et al.. Identification of precursors of leukemic dendritic cells differentiated from patients with acute myeloid leukemia.Leukemia. 2002;16(11):2267–74.
- Tsuchiya T, Hagihara M, Shimakura Y, Ueda Y, Gansuvd B, Munkhbat B, et al.. The generation of immunocompetent dendritic cells from CD34+ acute myeloid or lymphoid leukemia cells. Int J Hematol. 2002;75(1):55–62.
- Harrison BD, Adams JA, Briggs M, Brereton ML, Yin JA. Stimulation of autologous proliferative and cytotoxic T-cell responses by ‘leukemic dendritic cells’ derived from blast cells in acute myeloid leukemia. Blood. 2001;97(9):2764–71.
- Draube A, Beyer M, Wolf J. Activation of autologous leukemia-specific T cells in acute myeloid leukemia: monocyte-derived dendritic cells cocultured with leukemic blasts compared with leukemia-derived dendritic cells. Eur J Haematol. 2008;81(4):281–8.
- Decker T, Lohmann-Metthes ML. A quick and simple method for the quantitation of lactate dehydrogenase release in measurements of cellular cytotoxicity and tumor necrosis factor (TNF) activity. J Immunol Methods. 1988;115:61–9.
- Caux C, Dezutter-Dambuyant C, Schmitt D, Banchereau J. GM-CSF and TNF-alpha cooperate in the generation of dendritic Langerhans cells. Nature. 1992;360(6401):258–61.
- Choudhury A, Gajewski JL, Liang JC, Popat U, Claxton DF, Kliche KO, et al.. Use of leukemic dendritic cells for the generation of antileukemic cellular cytotoxicity against Philadelphia chromosome-positive chronic myelogenous leukemia. Blood. 1997;89(4):1133–42.
- Coleman S, Throp D, Fisher J, Bailey-Wood R, Lim SH. Cytokine enhancement of immunogenicity in chronic myeloid leukaemia. Leukemia. 1997;11(12):2055–9.
- Lee JJ, Kook H, Park MS, Nam JH, Choi BH, Song WH, et al.. Immunotherapy using autologous monocyte-derived dendritic cells pulsed with leukemic cell lysates for acute myeloid leukemia relapse after autologous peripheral blood stem cell transplantation. J Clin Apher. 2004;19(2):66–70.
- Lee JJ, Nam CE, Nam JH, Lee HC, Chung IJ, Park MS, et al.. Generation of cytotoxic donor CD8+ T cells against relapsing leukemic cells following allogeneic transplantation by stimulation with leukemic cell- or leukemic lysate pulsed donor cell-derived dendritic cells. Leuk Res. 2004;28(5):517–24.
- Maggio R, Peragine N, Calabrese E, De Propris MS, Intoppa S, Della Starza I, et al.. Generation of functional dendritic cells (DC) in adult acute lymphoblastic leukemia: rationale for a DC-based vaccination program for patients in complete hematological remission. Leuk Lymphoma. 2007;48(2):302–10.
- Mahnke K, Ring S, Johnson TS, Schallenberg S, Schonfeld K, Storn V, et al.. Induction of immunosuppressive functions of dendritic cells in vivo by CD4+CD25+ regulatory T cells: role of B7-H3 expression and antigen presentation. Eur J Immunol. 2007;37(8):2117–26.
- Veldhoen M, Moncrieffe H, Hocking RJ, Atkins CJ, Stockinger B. Modulation of dendritic cell function by naive and regulatory CD4+ T cells. J Immunol. 2006;176(10):6202–10.