Abstract
We previously demonstrated that reactive oxygen species (ROS) could be involved in ultraviolet-C (UVC)-induced DNA damage in Escherichia coli cells. In the present study, we evaluated the involvement of the GO system proteins in the repair of the 8-oxo-7,8-dihydro-2′-deoxyguanosine (8-oxoG, GO) lesion, which is ROS-induced oxidative damage. We first found that the mutant strain Δfur, which produces an accumulation of iron, and the cells treated with 2,2′-dipyridyl, a iron chelator, were both as resistant to UVC-induced lethality as the wild strain. The 8-oxoG could be mediated by singlet oxygen (1O2). The Fpg protein repaired this lesion when it was linked to C (cytosine), whereas the MutY protein repaired 8-oxoG when it was linked to A (adenine). The survival assay showed that the Fpg protein, but not the MutY protein, was important to UVC-induced lethality and interacted with the UvrA protein, a nucleotide excision repair (NER) protein involved in UVC repair. The GC–TA reversion assay in the mutant strains from the ‘8-oxoG-repair’ GO system showed that UVC-induced mutagenesis in the fpg mutants, but not in the MutY strain. The transformation assay demonstrated that the Fpg protein is important in UVC repair. These results suggest that UVC could also cause indirect ROS-mediated DNA damage and the Fpg protein plays a predominant role in repairing this indirect damage.
Introduction
Despite the importance of the increased oxygen concentration on the Earth's surface to the maintenance of life, it induces some harmful effects through the formation of certain substances. This is due to the production of reactive oxygen species (ROS) during breathing,Citation1 which include the superoxide anion radical (O2•−), hydroxyl radical (•OH), hydrogen peroxide (H2O2), singlet oxygen (Citation1O2), and others,Citation2 which can cause damage to DNA and other macromolecules such as proteins, lipids, and carbohydrates.Citation3 This oxidative stress is an important agent of mutagenesis, carcinogenesis, aging, and a number of diseases.Citation4,Citation5
The ultraviolet (UV) radiation from the Sun that reaches the Earth's surface consists of near-UV radiation, ultraviolet-A (UVA, 400 < λ < 320 nm) and ultraviolet-B (UVB, 320 < λ < 290 nm). Far-UV radiation, or ultraviolet-C (UVC, 290 < λ < 100 nm), is filtered by the Earth's ozone layer and atmosphere.Citation6
It is known that UV radiation changes several chemical and biological processes, leading to an increased incidence of skin cancer, eye damage, and erythema.Citation7 DNA is the main chromophore of UV radiation, and is the target of its impacts or effects.Citation8 Therefore, exposing cells to UV radiation is one of the methods used to explore the biological consequences of damage to DNA, its repair, and tolerance.Citation4 DNA damage by UV radiation is produced by direct and indirect effects. The direct effect results from the interaction of the energy from the radiation with DNA, leading to lesions such as cyclobutane pyrimidine dimers, pyrimidinehydrates, and adducts of pyrimidine. The indirect effects result from the interaction of radiation with intermediate-energy molecules, such as tryptophan, cysteine, riboflavin, and porphyrins, leading to the generation of ROS.Citation4
The hydroxyl radical •OH causes oxidative DNA damage,Citation9,Citation10 and there is evidence to indicate that Citation1O2 is also involved in this oxidative damage.Citation11 This ROS is produced by several biological systemsCitation12 and can be formed by photosensitive reactions, which take place when chromophores are excited upon exposure to visible or UV light. This energy can be transferred to oxygen, converting it into Citation1O2.Citation2
It has been shown that Citation1O2 reacts preferentially with guanine residues, whether they are free or composing the DNA molecule, leading to a variety of lesions at specific guanine sites. The most commonplace of these lesions is 8-oxo-7,8-dihydro-2′-deoxyguanosine (8-oxoG)Citation13,Citation14 which is mutagenic and lethal if not repaired.Citation15,Citation16
Several studies show that near-UV (UVA and UVB) radiation may generate ROS.Citation17 In contrast, it has been demonstrated that the lethality of far-UV (UVC) radiation is mainly caused by the formation of adducts between neighboring pyrimidines (direct lesions), which are lethal if not repaired.Citation18
Aerobic organisms have developed complex defense and repair mechanisms to mitigate the harmful effects of ROS.Citation19 Base excision repair and nucleotide excision repair (NER) are two mechanisms for DNA damage repair.
The UvrABC complex can undertake NER in a variety of lesions induced by ionizing radiation and oxidizing agents, such as AP sites, thymine glycols, pyrimidine dimers, and platinum adducts.Citation20
When 8-OxoG is misincorporated during DNA replication this causes GC to TA transversions. The removal of 8-oxoG, in Escherichia coli, involves a repair mechanism called the ‘GO system’.Citation21 The formamidopyrimidine glycosylase (Fpg) enzyme encoded by the mutM geneCitation22,Citation23 excises 8-oxoG from the 8-oxoG/C pairs with cytosine, whereas the MutY protein excises misincorporated A from 8-oxoG/A pairs. Meanwhile, in prokaryotes, direct UVC-induced damage is repaired by the UvrABC exonuclease, which carries out the entire process of damage recognition and excision.Citation4
In contrast, some studies have shown that UVC causes oxidative damage, such as 8-oxoG,Citation24 8-hydroxy-deoxiguanine (8-OHdG),Citation25 and thymine glycol.Citation26 Moreover, it has been suggested that UVC could generate ROS via lipid peroxidation in mammalian cells.Citation27 Our results corroborate this suggestion that UVC can also cause indirect DNA damage.Citation28
Materials and methods
Bacterial strains and plasmids
The E. coli strains and plasmids used in this work are described in . The DNA plasmid pUC 9.1, hosted by E. coli DH5aF'Iq (rec−), was extracted according to Sambrook and Russell.Citation29
Table 1. E. coli K-12 bacterial strains and plasmids used in this work
Growth conditions
Cells were grown overnight (in a shaking incubator at 37°C) in LB (Luria-Bertani)-rich medium.Citation30 A starting inoculum was taken from these cultures and cells were grown in the same medium until the mid-exponential growth phase (2 × 108 cells/ml).
Survival experiments
The experiments were performed with cells in the mid-exponential growth phase. The cells grown in LB medium were centrifuged and resuspended in saline (NaCl 0.9%) to the same density, then irradiated with different doses of UVC (254 nm, G15T8, 15 W General Electric germicidal lamp, New York, NY, USA). Dosimetry was performed using an ultra-sensitive DC micro-ammeter (Mount Vernon, New York, NY, USA) with a dose rate of 0.5 J/m2 s. 0.1 ml samples were collected, diluted in saline, and placed (in duplicate) in LB medium solidified with 1.5% agar. The colonies formed were counted after overnight incubation at 37°C. Additionally, cells grown to mid-exponential phase in LB medium were pre-treated for 20 minutes in the presence or absence of 1 mM dipyridyl (Sigma), then centrifuged, resuspended in saline, and irradiated with different doses of UVC. Survival data were analyzed using the analysis of variance (ANOVA) test to detect significant differences (P < 0.05) between the tested strains. To identify the differences, the Tukey–Kramer multiple comparison test was employed for all the strains using the InStat statistical program version 4.0 (GraphPad Software, San Diego, CA, USA).
E. coli lacZ reversion mutation assay
This mutagenic assay was described by Cupples and MillerCitation31 and very well explained by Josephy.Citation32 Cells were grown overnight at 37°C with shaking in a minimal LB medium consisting of a C-saltCitation33 medium enriched with lactose supplemented with 0.5% glucose, 0.2% casamino acids, and 2 µg/ml thiamine. When the cultures reached the mid-exponential growth phase (2 × 108 cells/ml) they were irradiated with different doses of UVC (254 nm), centrifuged, and resuspended in the same volume of C-salts medium without glucose. 0.1 ml aliquots diluted to 10−6 were plated on LB plates and 0.1 ml aliquots were plated on C-salt plates enriched with 0.5% lactose and 2 µg/ml thiamine. Plates were incubated at 37°C and screened after 1 day for viable cells, or after 2 days for Lac+ revertants. Only Lac+ revertants produce readily visible colonies on selective plates. Reversion frequencies were calculated by dividing the number of Lac+ revertants by the total number of viable cells. This assay was repeated at least five times and the data were analyzed using the ANOVA test, followed by Tukey's multiple comparison test (P < 0.05) using the InStat statistical program, version 4.0 (GraphPad Software, San Diego, CA, USA).
Bacterial plasmid pUC 9.1 transformation efficiency
The evaluation of bacterial transformation efficiency obtained from plasmid pUC 9.1 is determined by calculating the percentage of colony-forming units (CFUs) transformed by the plasmid (200 ng) by comparing the control sample and treated samples. The bacterial suspension (approximately 109 cells/ml) was mixed with pretreated plasmid DNA and incubated on ice for 20 minutes. Afterwards, the tubes were placed in a water bath at 42°C for 4 minutes followed by incubation on ice for 1 minutes, then 500 µl liquid LB was added. The cultures were then incubated for 1 hour at 37°C, and aliquots were plated onto LB-agar containing ampicilin (60 µg/ml) and incubated at 37°C overnight, after which time the number of CFUs was counted.Citation29 The sample control had a transformation efficiency of 100%. This assay was repeated at least five times and the collected data were submitted to the chi-square test (2), adopting 5% as the significance level.
Results and discussion
UVC radiation is one of the models used as a positive control in studies of direct DNA damage, such as pyrimidine dimers, which are generated by the interaction of this agent with the cellular genome. Whether UVC can generate indirect damage is as yet a moot point to the scientific community. However, our previous results indicate that ROS mediate the lethality induced by UVC in E. coli cells.Citation28,Citation34 Other studies have also shown that UVC (254 nm) radiation can induce the formation of oxidative DNA lesions.Citation24,Citation25
ROS such as O2•− and Citation1O2, are formed in cells during aerobic metabolism and during oxidative stress.Citation2 The generation of these species by endogenous and exogenous sources may result in DNA damage. Thus ROS may be mutagenic and carcinogenic.Citation35,Citation36
However, under physiological conditions, O2•− does not produce DNA damage.Citation37,Citation38 Thus, the toxicity of this ROS is a result of its ion-catalyzed conversion into •OH, a highly reactive radical.Citation2
Likewise, the indirect damage mediated by ROS is mainly associated with •OH generation, through the Fenton reaction, which is dependent on transition metals such as Fe and Cu, resulting in serious damage to DNA.Citation2
Fur controls iron metabolism, repressing several genes involved in iron uptake by cells.Citation39 So, cells carrying a non-functional fur locus (Δfur mutant) present high levels of iron, which is sensitive to oxidative agents such as UVA.Citation40 2–2′-dipyridyl, is a Fe2+ chelator, which diminishes •OH formation through the Fenton reaction.
Then, by checking the survival fraction, we verified the influence of the bacterial Fur protein and the chelator dipyridyl on UVC-induced lethality, and we showed that Δfur mutant and dipyridyl treatment cells were as resistant to UVC-induced lethality as the wild-type strain (; P < 0.05). These data suggest that the background iron level does not interfere in the effect of indirect UVC-induced damage, which indicates that •OH was not the main UVC-induced ROS.
Figure 1. Survival fraction of E. coli Δfur mutant QC1732 (squares), wild-type GC4468 (diamonds) and wild-type pretreated with dipyridyl 1 mM, a Fe2+ chelator (circles) irradiated with different doses of UVC (254 nm). Values are the mean of at least five experiments and were submitted to ANOVA test followed Tukey's comparison test (P > 0.05).
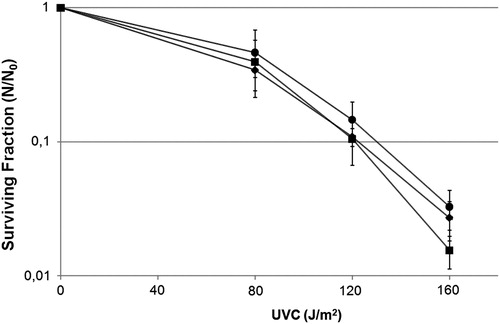
Our previous results showed that the mutant strain fpg was more resistant to UVC-induced lethality. Zhang et al.Citation24 showed that UVC could induce 8-oxoG oxidative damage, which indicates the participation of the GO system in UVC-induced damage.
The GO system was repaired, mainly with the participation of two proteins, the Fpg or MutM protein and the MutY protein. The former protein removes 8-oxoG paired with cytosine, whereas the latter removes 8-oxoG paired with A.
In order to verify the GO system's participation in UVC-induced lethality, experiments were conducted which showed that the MutY strain did not seem to influence the repair of UVC-induced lesions, since their survival was similar to that of the wild strain (; P < 0.05). However, the MutM mutant strain was more resistant than the wild-type strain to the cytotoxic damage induced by UVC (; P > 0.001). Insertion of the pFPG plasmid in all the transformed strains made the cells more sensitive to UVC (; P > 0.001). These results could be explained by the participation of Fpg in the repair mechanisms of UVC-induced damage, which involve the generation of many gaps, besides the ones generated by the NER pathway.Citation41 As such, the fpg− mutant strains could produce fewer DNA breaks than the wild-type strain.
Figure 2. Survival fraction of E. coli wild-type CC104 (diamonds), mutM− mutant BH540 (squares), mutY− mutant BH980 (triangles), mutM− mutY− mutant BH990 (circles) original strains (filled symbols), or transformed with pFPG plasmid (open symbols) irradiated with different doses of UVC (254 nm). Values are the mean of at least five experiments and were submitted to ANOVA test followed Tukey's comparison test (P > 0.05, wild strain compared with mutY− mutant or mutM− mutY− mutant; P < 0.001 for all other comparisons).
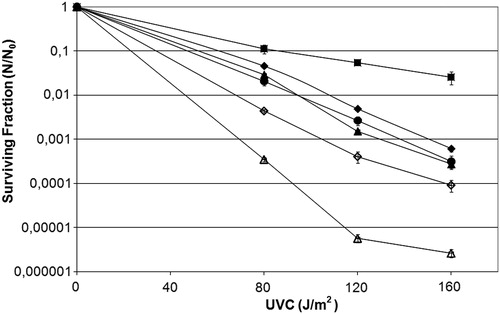
8-oxoG is a major lesion arising from oxidative stress in prokaryotes and eukaryotes, and is associated with cancer, aging, infertility, and others diseasesCitation42 and is therefore a lethal, mutagenic lesion. Citation1O2 is known to modify guanine residuals, and the major type of Citation1O2-induced DNA lesion is precisely 8-oxoG.Citation43 Citation1O2-induced mutagenecity damage mainly takes the form of base substitutions affecting G:C base pairs. The ability of 8-oxoG to mispair with dG causes GC–TA tranversions.Citation44
To verify the participation of 8-oxoG in UVC-induced mutagenesis, the lacZ mutation assay was performed. We used the E. coli strains created by Cupples and Miller,Citation31 which have a specific mutation in the lacZ gene that allows the rapid determination of GC–TA transversion, a transversion that could be induced by 8-oxoG.Citation45 The CC104 strain lost its ability to metabolize lactose (Lac− phenotype). The reversion to Lac+ recovered β-galactosidase synthesis.Citation46,Citation47
We observed that UVC induced the 8-oxoG mutagenic lesion and the Fpg protein was important in repairing this lesion (; P > 0.001). However, despite being part of the GO system repair, the MutY protein does not seem as important as MutM in UVC-induced mutagenesis (, P > 0.001).
Figure 3. Mutagenesis LacZ of E. coli wild-type CC104, mutM− mutant BH540 and mutY− mutant BH980 not treated (black column) or irradiated with 120 J/m2 of UVC (254 nm) (gray column). Values are the mean of at least five experiments and were submitted to ANOVA test followed Tukey's comparison test (P < 0.001).
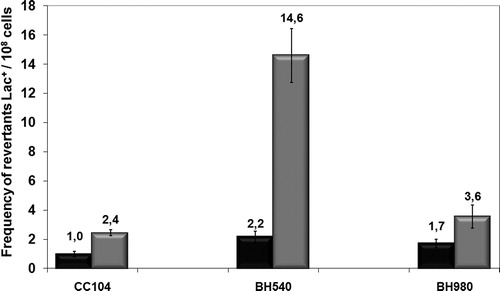
Boiteux et al.Citation48 suggest that mismatch repair mediated by MutY, acting synergistically with MutM, plays a major role in the prevention of 8-oxoG mutagenic effects. According to our results, the Fpg protein seemed to be very important in the repair of UVC-induced DNA lesions, indicating that UVC induces 8-oxoG in bacterial cells by generating Citation1O2, mediated by GC–TA transversion. However, the MutY protein would appear not to be so important in UVC-induced mutagenesis and lethality.
NER is able to repair a wide spectrum of DNA lesions. Proteins including UvrA, -B, -C, and -D are involved in the recognition of the lesion, cleavage at the 3′ and 5′ ends of that lesion, and the release of the patch of DNA including the damaged base.Citation49 Other researchers suggest that Fpg and UvrABC complement each other in the repair of lethal damage, 8-oxog, induced by Citation1O2.Citation20,Citation50
To confirm the importance of Fpg in the repair of UVC-induced lesions mediated by ROS, a survival assay was undertaken using uvrA− and uvrA− fpg− mutant strains transformed with pFPG plasmid. We observed that UVC damage induced nucleotide and base excision repair simultaneously, represented by Fpg and the UvrABC system (). In contrast, uvrA− fpg− mutant was more sensitive to UVC-induced lethality and the cells transformed with pFPG plasmid acquired significantly more resistance to this agent than the original strain (; P > 0.001).
Figure 4. Survival fraction of E. coli uvrA− mutant AB1886 (diamonds), uvrA− mutM− mutant RJF624 (squares) original strains (filled symbols), or transformed with pFPG plasmid (open symbols) irradiated with different doses of UVC (254 nm). Values are the mean of at least five experiments and were submitted to ANOVA test followed Tukey's comparison test (P > 0.05, uvrA− mutant compared with uvrA− mutM− mutant transformed with pFPG plasmid; P < 0.001 for all other comparisons).
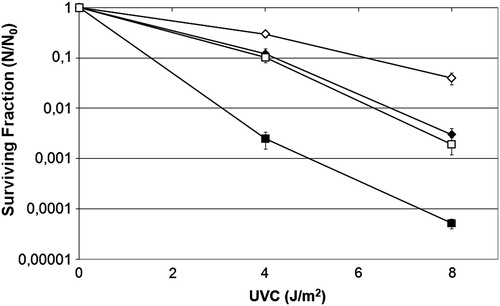
Although the above results may seem controversial, we can explain them by the fact under this circumstance when the uvrA strain is far more UVC-sensitive than the wild strain, although the UvrABC system does not generate any gaps, there is a build-up of unrepaired lesions, and the presence of Fpg acts in the repair of the lesions, reducing the overall number of lesions in the DNA, ultimately helping the cell to survive ().
Still, from the level of protection acquired by the strains transformed with plasmid pFPG (; P > 0.001), we could conjecture that the Fpg protein may not just be mediating 8-oxoG repair by Citation1O2, but could also be involved in the repair of direct damage produced by UVC, such as pyrimidine dimers. As such, the UvrABC system could also repair 8-oxoG lesions.
Another approach to assess UVC-induced genotoxicity and UVC repair mechanism was the bacterial transformation assay, used to check the transformation capacity of E. coli AB1157 and fpg− mutant (BH20) by pUC 9.1 DNA plasmid, which has a gene that is resistant to the antibiotic ampicillin. As such, we observed not only the capacity of the plasmid to penetrate cells, but also the capacity of the E. coli strain to repair a damaged plasmid. The transformation efficiency of the BH20 strain was significantly lower than that of the wild-type strain (, P > 0.001).
Figure 5. Efficiency of bacterial transformation with plasmid pUC 9.1 on strains AB1157 (black) and BH20 (gray) irradiated with 120 J/m2 of UVC. Values are the mean of at least five experiments and were submitted to chi square, adopting 5% as the significance level (P < 0.001).
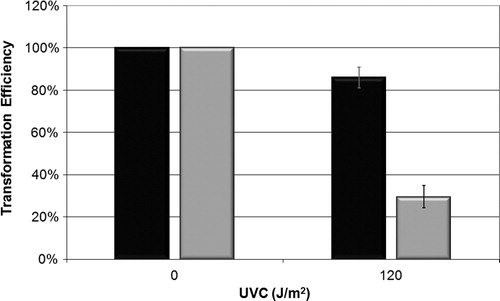
We therefore suggest that the repair of UVC-induced lesions was achieved with the participation of the Fpg protein, and that this repair process could generate apyrimidinic sitesCitation4 which made DNA strand breaks in E. coli cells, leading to an increase in lethality. Meanwhile, the presence of this protein seen through the insertion of the plasmid pFPG reduces the mutagenesis induced by this agent.
Acknowledgements
Research supported by Coordenação de Aperfeiçoamento de Pessoal de Nível Superior (CAPES), Fundação Carlos Chagas Filho de Amparo à Pesquisa do Estado do Rio de Janeiro (FAPERJ), Conselho Nacional de Desenvolvimento Científico e Tecnológico (CNPq), and Universidade do Estado do Rio de Janeiro (UERJ).
References
- Davies KJA. Oxidative stress: the paradox of aerobic life. Biochem Soc Symp 1994;61:1–31.
- Halliwell B, Gutteridge JMC. Free radicals in biology and medicine. 4th ed. New York, USA: Oxford University Press; 2007.
- Riley PA. Free radicals in biology: oxidative stress and the effects of ionizing radiation. Int J Radiat Biol 1994;65:27–33.
- Friedberg EC, Walker GC, Siede W, Wood RD, Schultz RA, Ellenberger T. DNA repair and mutagenesis. 2nd ed. Washington, DC: ASM Press; 2006.
- Farr SB, Kogoma T. Oxidative stress response in Escherichia coli and Salmonella typhimurium. Microbiol Rev 1991;55:561–85.
- Serafini DM, Schellhorn HE. Endonuclease III and endonuclease IV protect Escherichia coli from the lethal and mutagenic effects of near-UV radiation. Can J Microbiol 1999;45:632–7.
- Mandronich S, McKenzie RL, Björn LO, Caldwell MM. Changes in biologically active ultraviolet radiation reaching the Earth's surface. J Photochem Photobiol 1998;46:5–19.
- Eisenstark A. Mutagenic and lethal effects of near-ultraviolet radiation (290–400 nm) on bacteria and phage. Environm Mol Mut 1987;10:317–37.
- Cadet J, Teoule R. Comparative study of oxidation of nucleic acid components by hydroxyl radicals, singlet oxygen and superoxide anion radicals. Photochem Photobiol 1978;28:661–7.
- Ames BA, Gold LS. Endogenous mutagens and the cause of aging and cancer. Mutat Res 1991;250:3–16.
- Piette J. Biological consequences associated with DNA oxidation mediated by singlet oxygen. Photochem Photobiol 1991;11:241–60.
- Briviba K, Klotz LO, Sies H. Toxic and signaling effects of photochemically or chemically generated singlet oxygen in biological systems. Biol Chem 1997;378:1259–65.
- Tchou J, Grollman AP. Repair of DNA containing the oxidatively-damaged base, 8-oxoguanine. Mutat Res 1993;299:277–87.
- Agnez-Lima LF, Oliveira RLC, Di Mascio P, Menck CFM. Involvement of Escherichia coli exonuclease III and endonuclease IV in the repair of singlet oxygen-induced DNA damage. Carcinogenesis 1996;17:1183–5.
- Imlay JA, Linn S. DNA damage and oxygen radical toxicity. Science 1988;240:1302–9.
- Maki H, Sekiguchi M. MutT protein specifically hydrolyses a potent mutagenic substrate for DNA synthesis. Nature 1992;355:273–5.
- Rosen JE, Prahalad AK, Williams GM. 8-oxodeoxyguanosine formation in the DNA of cultured cells after exposure to H2O2 alone or with UVB or UVA irradiation. Photochem Photobiol 1996;64:117–22.
- Hart RW, Setlow RB, Woodhead AD. Evidence that pyrimidine dimers in DNA can give rise to tumors. Proc Natl Acad Sci USA 1977;74:5574–8.
- Beyer WF, Imlay J, Fridovich I. Superoxide dismutases. Prog Nucleic Acid Res Mol Biol 1991;40:221–53.
- Czeczot H, Tudek B, Lambert B, Laval J, Boiteux S. Escherichia coli Fpg protein and UvrABC endonuclease repair DNA damage induced by methylene blue plus visible light in vivo and in vitro. J Bacteriol 1991;173:3419–24.
- Michaels ML, Miller JH. The GO system protects organisms from the mutagenic effect of the spontaneous lesion 8-hydroxyguanine (7,8-dihydro-8-oxoguanine). J Bacteriol 1992;174:6321–5.
- Boiteux S, Radicella JP. Base excision repair of 8-hydroxyguanine protects DNA from endogenous oxidative stress. Biochimie 1999;81:59–67.
- Fowler RG, White SJ, Koyama C, Moore SC, Dunn RL, Schaaper RM. Interactions among the Escherichia coli mutT, mutM, and mutY damage prevention pathways. DNA Repair (Amst) 2003;2:159–73.
- Zhang X, Rosenstein BS, Wang Y, Lebwohl M, Wei H. Identification of possible reactive oxygen species involved in ultraviolet radiation-induced oxidative DNA damage. Free Rad Biol Med 1997;23:980–5.
- Wei H, Cai Q, Rahn R, Zhang X. Singlet oxygen involvement in ultraviolet (254 nm) radiation induced formation of 8-hydroxy-deoxyguanosine in DNA. Free Rad Biol Med 1997;23:148–54.
- Demple B, Linn S. 5,6-Satured thymine lesions in DNA: production by ultraviolet light or hydrogen peroxide. Nucleic Acids Res 1982;10:3781–9.
- Devary Y, Gottlieb RA, Smeal T. The mammalian ultraviolet response is triggered by activation of Src tyrosine kinase. Cell 1992;71:1081–91.
- Silva-Júnior ACT, Asad LMBO, Felzenszwalb I, Asad NR. Mutagenicity induced by UVC in Escherichia coli cells: reactive oxygen species involvement. Redox Report 2011;16(5):187–92.
- Sambrook J, Russell DW. Molecular cloning: a laboratory manual. 3rd ed. Plainview, NY: Cold Spring Harbor Laboratory Press; 2001.
- Miller JH. A short course in bacterial genetics. Cold Spring Harbor, NY: Cold Spring Harbor Laboratory Press; 1992.
- Cupples CG, Miller JH. A set of lacZ mutations in Escherichia coli that allow rapid detection of each of the six base substitutions. Proc Natl Acad Sci USA 1989;86:5345–9.
- Josephy PD. The Escherichia coli lacZ reversion mutagenicity assay. Mut Res 2000;455:71–80.
- Vogel HJ, Bonner DM. Acetylornithinase of Escherichia coli: partial purification and some properties. J Biol Chem 1956;218(1):97–106.
- Gomes AA, Silva-Júnior ACT, Oliveira EB, Asad LMBO, Reis NCSC, Felzenszwalb I, et al. Reactive oxygen species mediate lethality induced by far-UV in Escherichia coli cells. Redox Rep 2005;10:91–5.
- Ames BN. Dietary carcinogens and anticarcinogens. Science 1983;22:1256–64.
- Breimer LH. Molecular mechanisms of oxygen radicalcarcinogenesis and mutagenesis: the role of DNA base damage. Mol Carcinog 1990;3:188–97.
- Aruoma OI, Halliwell B, Dizdaroglu M. Iron ion-dependent modification of bases in DNA by the superoxide radical-generating system hypoxanthine/xanthine oxidase. J Biol Chem 1989;264(22):13024–8.
- Blakely WF, Fuciarelli AF, Wegher BJ, Dizdaroglu M. Hydrogen peroxide-induced base damage in deoxyribonucleic acid. Radiat Res 1990;121:338–43.
- Touati D, Jacques M, Tardat B, Bouchard L, Despied S. Lethal oxidative damage and mutagenesis are generated by iron in Δfur mutants of Escherichia coli: protective role of superoxide dismutase. J Bacteriol 1995;177(9):2305–14.
- Hoerter JD, Arnold AA, Ward CS, Sauer MG, Johnson S, Fleming TJ, et al. Reduced hydroperoxidase (HPI and HPII) activity in the Δfur mutant contributes to increased sensitivity to UVA radiation in Escherichia coli. J Photochem Photobiol B: Biol 2005;79:151–7.
- Caldeira de Araújo A, Favre A. Near ultraviolet DNA damage induces the SOS response in Escherichia coli. EMBO J 1986;5:175–9.
- Zang H, Irimia A, Choi J, Angel KC, Loukachevitch LV, Egli M, et al. Efficient and high fidelity incorporation of dCTP opposite 7,8-dihydro-8-oxodeoxyguanosine by sulfolobus solfataricus DNA polymerase Dpo4. J Biol Chem 2006;281(4):2358–72.
- Cadet J, Berger M, Douki T, Morin B, Raoul S, Ravanat JL, et al. Effects of UV and visible radiation on DNA – final base damage. Biol Chem 1997;378:1275–86.
- Menck CFM, Di Mascio P, Agnez LF, Ribeiro DT, Costa de Oliveira R. Genetic deleterious effects of singlet oxygen. Quimica Nova 1993;16:328–36.
- Cheng KC, Cahill DS, Kasai H, Nishimura S, Loeb LA. 8-Hydroxyguanine, an abundant form of oxidative DNA damage, causes G-T and A-C substitutions. J Biol Chem 1992;267:166–72.
- Cupples CG, Cabrera M, Cruz C, Miller JH. A set of lacZ mutations in Escherichia coli that allow rapid detection of specific frameshift mutations. Genetics 1990;125:275–80.
- Borden A, O'Grady PI, Vandewiele D, Fernandez de Henestrosa AR, Lawrence CW, Woodgate R. Escherichia coli DNA Polymerase III can replicate efficiently past a T-T cis-syn cyclobutane dimer if DNA Polymerase V and the 3′ to 5′ exonuclease proofreading function encoded by dnaQ are inactivated. J Bacteriol 2002;184:2674–81.
- Boiteux S, Gellon L, Guibourt N. Repair of 8-oxoguanine in Saccharomyces cerevisiae: interplay of DNA repair and replication mechanisms. Free Rad Biol Med 2002;32:1244–53.
- Batty DP, Wood RD. Damage recognition in nucleotide excision repair of DNA. Gene 2000;241:193–204.
- Agnez-Lima LF, Napolitano RL, Fuchs RPP, Di Mascio P, Muotri AR, Menck CFM. DNA repair and sequence context affect 1O2-induced mutagenesis in bacteria. Nucleic Acids Res 2001;29:2899–903.