Abstract
Objective
The aim of this study was to determine whether an increase of intracellular nitric oxide (NO) level signals postovulatory aging-induced abortive spontaneous egg activation (SEA) in rats.
Methods
Freshly ovulated eggs (arrested at metaphase-II stage; M-II) were cultured in vitro for 3 hours to induce postovulatory egg aging. The morphological changes, inducible nitric oxide synthase (iNOS) expression, NO, cytosolic free Ca2+, 3′,5′ cyclic guanosine monophosphate (cGMP), cell division cycle 25B (Cdc25B) and Wee1 levels, specific phosphorylation (pThr-14/Tyr-15) as well as total cyclin-dependent kinases-1 (Cdk1) (PSTAIRE) levels were analyzed.
Results
Postovulatory aging induced generation of NO possibly through an iNOS-mediated pathway. The increase in NO level was associated with augmented cytosolic free Ca2+ as well as cGMP levels in aged eggs. A significant increase in Wee1 level and decrease of Cdc25B level were observed in aged eggs. An accumulation of phosphorylated Cdk1 (pThr-14/Tyr-15) level was observed in aged eggs, while total Cdk1 (PSTAIR) level remained unchanged.
Conclusion
Our study demonstrates that generation of NO through an iNOS-mediated pathway increases cytosolic free Ca2+and cGMP levels. High levels of these signal molecules trigger the accumulation of phosphorylated Cdk1 in aged eggs. Thus, NO signals the accumulation of phosphorylated Cdk1 and induces postovulatory aging-induced abortive SEA in the rat.
Introduction
In most mammalian species, ovulated eggs remain arrested at the metaphase-II (M-II) stage of the meiotic cell cycle until fertilization. However, in few mammalian species including the rat, ovulated eggs do not wait for fertilization, instead undergo spontaneous exit from M-II arrest by initiating the extrusion of the second polar body, a morphological feature of spontaneous egg activation (SEA).Citation1–Citation4 Postovulatory egg aging generates a pathological condition and limits the outcome of assisted reproduction technologies (ARTs) in animals as well as in humans.Citation5 Although postovulatory aging-induced abortive SEA has been reported in several mammalian species, viz. rat,Citation2 mouse,Citation6 bovine,Citation7 porcine,Citation8 and humans,Citation9 the relevant factor(s) and mechanism that drives it remain to be elucidated.
A growing body of evidence suggests that nitric oxide (NO) acts as a signal molecule and modulates various aspects of cell functions. For example, NO induces the release of cytosolic free Ca2+ in interstitial cells,Citation10 macrophage cell line,Citation11 beta cells of the pancreas,Citation12 and eggs of sea urchin,Citation13 mouse,Citation14 and pig.Citation15 The involvement of cGMP/cADRP/RyR channels during NO-mediated Ca2+ mobilization has been proposed in several somatic cell typesCitation16 and during egg activation.Citation13,Citation15 Further, NO-mediated opening of RyR channels through S-nitrosylation has been demonstrated in skeletal musclesCitation17 and Purkinje cells.Citation18 The involvement of RyR channels has been reported during egg activation in pigsCitation15 and abortive SEA in rats.Citation4 However, the involvement of NO and 3′,5′ cyclic guanosine monophosphate (cGMP) in the regulation of the meiotic cell cycle during postovulatory aging remains poorly understood. Further, previous studies suggest that active Protein Kinase-A (PKA) increases cell division cycle 25B (Cdc25B) phosphorylation and inhibits cyclin-dependent kinases-1 (Cdk1) activity possibly by inducing dephosphorylation of Cdk1 at pThr-14/Tyr-15 residues.Citation19,Citation20
Maturation promoting factor (MPF) is a heterodimeric complex of a catalytic subunit, Cdk1, and a regulatory subunit, cyclin-B1. In general, Cdk1 is phosphorylated at Thr-14/Tyr-15 by the Wee1 kinase after association with cyclin-B1.Citation21 The activation of MPF depends upon the dephosphorylation of Cdk1 at Thr-14/Tyr-15 by Cdc25B phosphatase.Citation22 In mice, the inhibitory phosphorylation is catalyzed by the Wee1 kinase,Citation23 while dephosphorylation is mediated by Cdc25B phosphatase.Citation24 However, accumulation of phosphorylated Cdk1 (pThr-14/Tyr-15) and degradation of cyclin-B1 are two important events of MPF inactivation.
Downstream of NO, cGMP modulates cAMP level and signals meiotic resumption from diplotene arrest, possibly by regulating Cdc25B and Wee1 through a PKA-mediated pathway.Citation19,Citation20 Based on these studies, we hypothesized that the increase of intracellular NO through an iNOS-mediated pathway could mediate the increase of cytosolic free Ca2+ as well as cGMP levels in eggs. The increase of these signal molecules may induce accumulation of phosphorylated Cdk1 (pThr-14/Tyr-15), possibly by altering both Wee1 and Cdc25B levels. Therefore, this study was carried out to determine whether NO generated through an iNOS-mediated pathway can modulate Ca2+ and cGMP levels and phosphorylate Cdk1 (pThr-14/Tyr-15) during postovulatory aging-induced abortive SEA in rat eggs cultured in vitro.
Materials and methods
Chemicals and antibodies
Chemicals used in this study were purchased from Sigma Chemical Co. (St Louis, MO, USA), unless stated otherwise. All immunolocalization experiments were performed using primary antibodies specifically raised against iNOS, Cdc25B, phosphorylated Cdk1 (pThr-14/Tyr-15), total Cdk1 (PSTAIRE), and these as well as FITC- or TRITC-labelled secondary antibodies were purchased from Santa Cruz Biotechnology Inc., CA, USA.
Experimental animals and collection of ovulated eggs
Immature female (22–24 days old) rats of Rattus norvegicus (Charles-Foster strain) were kept in a light-controlled room, with food and water ad libitum. These rats were subjected to superovulation induction following a published protocol.Citation4 Freshly ovulated eggs were collected at 14 hours post human chorionic gonadotropin surge from the oviduct by puncturing with a 26-gauge needle and transferred to Ca2+-deficient medium (Medium-199, Cat. no. AL043A; HiMedia Laboratories, Mumbai, India) at 37 °C. Eggs were denuded with medium containing 0.01% w/v hyaluronidase at 37 °C for 3 minutes to remove encircling cumulus cells. All experiments were performed in accordance with the Guide for the Care and Use of Laboratory Animals, published by the US National Institutes of Health (NIH Publication No. 85-23, revised 1985). The experimental procedures used in this study were approved by the Animal Ethical Committee of the University.
Collection of aged eggs for in vitro studies
Eggs collected at 14 hours post human chorionic gonadotropin surge were cultured in Ca2+-deficient medium-199 for 3 hours at 37 °C in a CO2 incubator (Galaxy 170 R, Eppendorf Company, UK) to induce postovulatory egg aging in vitro. Eggs under in vitro culture conditions experienced postovulatory aging, which is morphologically observed by the initiation of extrusion of the second polar body.Citation4 Control eggs (eggs arrested at M-II) as well as eggs that underwent abortive SEA were separated and used for experiments.
Detection of iNOS expression
The phosphorylation status of iNOS was detected using an anti-iNOS polyclonal antibody. For this purpose, 10–15 eggs from each group were prefixed overnight with 3.7% v/v buffered formaldehyde. Eggs were then permeabilized with Triton X-100 (0.01% v/v in PBS) for 10 minutes at 37 °C and then washed three times with PBS. Nonspecific sites were blocked using blocking buffer (2.5% w/v BSA in PBS) at 37 °C for 30 minutes and then exposed to 100 µL of iNOS antibody (1:500 dilution in blocking buffer) at 37 °C for 1 hour. After five washes with PBS, the eggs were exposed to 100 µL of FITC-labeled secondary antibody (1:1000 dilution in blocking buffer) for 1 hour at 37 °C in a humidified chamber. At the end of the incubation, the eggs were washed five times with PBS, mounted with anti-fade mounting medium (Himedia laboratories, Mumbai, India) to prevent photobleaching during immunofluorescence analysis using an epi-fluorescence microscope (Model, Ni-U, Nikon Eclipse, Tokyo, Japan) at 520 nm at 400× magnification.
Quantitative analysis of NO concentration
The intracellular NO concentration was analyzed using a NO assay kit (R&D Systems, MN, USA). Egg lysate was prepared from the control as well as the abortive SEA group (100 eggs from each group) and the total nitrite level was analyzed according to the manufacturer's protocol. In brief, all reagents, working standards and samples were brought to room temperature before use. Then 50 µL of reaction diluent was added to the blank well and 50 µL of nitrate standards or samples to the remaining wells. NADH (25 µL) followed by 25 µL of diluted nitrate reductase were added to all wells. The plate was incubated at 37 °C for 30 minutes in the dark at room temperature. At the end of the incubation, 50 µL of Griess reagent-I followed by 50 µL of Griess reagent-II were added. The content was mixed well by tapping the side of the plate and then incubated for 10 minutes at room temperature. At the end of the incubation period, the optical density was measured using a microplate reader (Micro Scan MS5608A, ECIL, Hyderabad, India) set at 540 nm with wavelength correction at 690 nm.
Analysis of cytosolic free Ca2+ level
The cytosolic free Ca2+ level was analyzed in eggs of different groups following a previously published protocol.Citation4 In brief, 10–15 eggs from each group (control as well as abortive SEA samples) were transferred to Ca2+-free medium containing 50 µM Fluo-3 AM and incubated for 20 minutes at 37 °C in a CO2 incubator. After 20 minutes, eggs were removed and washed three times with Ca2+-free medium and observed under an epi-fluorescence microscope (Nikon, Eclipse; E-80i, Japan) using ex488 nm and em525 nm. The corrected total cell fluorescence (CTCF) was calculated using Image J Software (version 1.44 from National Institutes of Health, USA).
Quantitative analysis of cGMP concentration
The intracellular cGMP level was measured using a cGMP assay kit (R&D Systems, MN, USA) according to the manufacturer's protocol. In brief, egg lysate was prepared from each group (100 eggs each from control as well as abortive SEA group) using 100 µL of hypotonic lysis buffer (5 mM Tris, 20 mM EDTA, 0.5% v/v Triton X-100, pH 8) on ice. The lysate was centrifuged at 10 000× g at 4 °C for 15 minutes and the supernate was used to quantify intracellular cGMP concentration. All reagents were prepared (working standards and samples) as per the manufacturer's protocol. Then 150 µL of diluent was added to nonspecific binding (NSB) wells and 100 µL to the zero standard (B0) wells. Then 100 µL of standard, control, or samples were added to the remaining wells. Subsequently, 50 µL of cGMP conjugate was added to each well followed by 50 µL of primary antibody (except NSB wells). The plate was incubated for 3 hours at room temperature and then washed five times with washing buffer. After the last wash, 200 µL of substrate solution was added to all wells and incubated in the dark for 30 minutes at room temperature. At the end of the incubation period, 50 µL of stop solution was added to all wells and the optical density was measured (within 30 minutes) using a microplate reader (Micro Scan MS5608A, ECIL, Hyderabad, India) set to 450 nm with wavelength correction at 540 nm.
Analyses of Wee1 and Cdc25B expression levels
The Wee1 as well as Cdc25B levels were detected in eggs using anti-Wee1 as well as anti-Cdc25B polyclonal antibodies. These primary antibodies are highly specific and developed against respective epitopes. For this purpose, 10–15 eggs (from each group) were prefixed, permeabilized, washed, and blocked as described earlier and then exposed to 100 µL of anti-Wee1 or anti-Cdc25B primary antibodies (1:500 dilution in blocking buffer) at 37 °C for 1 hour. After five washes with PBS, the eggs were exposed separately to 100 µL of FITC-labeled secondary antibody (1:1000 dilutions in blocking buffer) for 1 hour at 37 °C in humidified chamber. After incubation, the slides were washed five times with PBS, mounted with anti-fade mounting medium and analyzed using the epi-fluorescence microscope at 520 nm at 400× magnification.
Analyses of total Cdk1 and Thr-14/Tyr-15 phosphorylated Cdk1 levels
The phosphorylation status of Cdk1 at Thr-14/Tyr-15 and PSTAIR was detected using an anti-Thr-14/Tyr-15, anti-PSTAIR polyclonal antibody. For this purpose, 10–15 eggs (from each group) were prefixed, permeabilized, and washed PBS as described. The nonspecific sites were blocked using blocking buffer (2.5% PBS–BSA solution) at 37 °C for 30 minutes and then exposed to 100 µL of their respective primary antibody (1:500 dilution in blocking buffer) at 37 °C for 1 hour. After five washes with PBS, the eggs were exposed to 100 µl of either TRITC- or FITC-labeled secondary antibody (1:1000 dilutions in blocking buffer) for 1 hour at 37 °C in humidified chamber. At the end of the incubation, the eggs were washed five times with PBS, mounted with anti-fade mounting media and analyzed using the epi-fluorescence microscope at 520 nm at 400× magnification.
Statistical analyses
Data are expressed as mean ± standard error of mean (SEM) of three independent experiments. Before statistical analysis, arcsine square-root transformation was performed for all percentage data. Student's t-test was used for data analysis using SPSS software, Version 17.0 (SPSS, Inc. Chicago, IL, USA). A probability of P < 0.05 was considered as statistically significant.
Results
Postovulatory aging increases iNOS expression and NO level
Freshly ovulated eggs were arrested at the M-II stage of the meiotic cell cycle and possessed first polar body with normal morphology (A and B, blue arrow head). On the other hand, abortive SEA (EM-II) was morphologically characterized by initiation of extrusion of the second polar body (C and D, red arrow head). The iNOS expression was increased in eggs that underwent abortive SEA (EM-II) as evidenced by increased immunofluorescence intensity in egg cytoplasm (B, white arrow) as compared to control egg (A). This observation was further confirmed by CTCF analysis (C). The quantitative analysis of NO level suggested that postovulatory aging significantly (P < 0.05) increased NO level (0.73 ± 0.03 pmol/egg) in eggs that underwent abortive SEA, compared to control eggs arrested at M-II stage (0.45 ± 0.02 pmol/egg) of the meiotic cell cycle ().
Figure 1. Representative phase-contrast photographs of eggs in culture medium showing postovulatory aging-induced abortive SEA. (A) Control eggs arrested at the M-II stage showing the first polar body. (B) Egg arrested at the M-II stage showing degenerating first polar body (blue arrow head). (C) Eggs undergoing postovulatory aging-induced abortive SEA. (D) Egg showing initiation of the second polar body (red arrow head) and first polar body (blue arrow head).
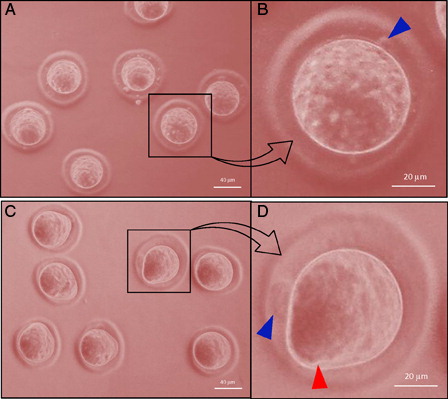
Figure 2. Representative photographs showing fluorescence intensity of iNOS during postovulatory aging-induced abortive SEA. (A) Control egg (M-II). (B) An increase of iNOS fluorescence intensity in egg that underwent postovulatory aging-induced abortive SEA (EM-II). (C) Bars showing CTCF analysis of fluorescence intensity of three independent experiments. Values are mean ± SEM of three independent experiments. Data analyzed by Student's t-test, *P < 0.001.
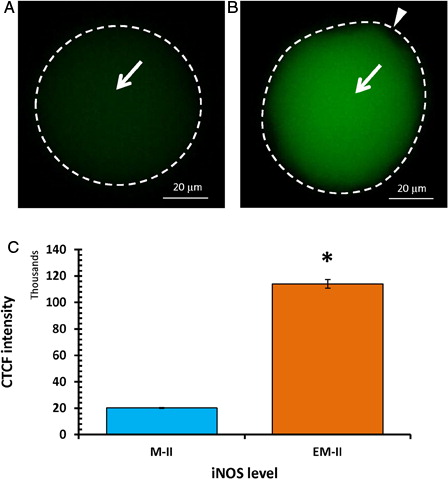
Postovulatory aging increases cytosolic free Ca2+ and cGMP levels
As shown in , an increase in fluorescence intensity of Fluo-3 was observed in eggs that underwent postovulatory aging-induced abortive SEA (B, white arrow), compared to control eggs (M-II) (A, white arrow). The CTCF analysis using Image J software of three independent experiments further supported our observations that the cytosolic free Ca2+ level significantly increased in eggs that underwent postovulatory aging-abortive SEA (EM-II) (C). Similarly, postovulatory aging-induced abortive SEA was associated with a significant (P < 0.05) increase of cGMP (0.132 ± 0.036 pmol/egg) level, compared to control eggs (0.064 ± 0.013 pmol/egg) that were arrested at M-II stage ().
Figure 4. Representative photographs of postovulatory aging-induced increase of cytosolic free Ca2+ level. Cytosolic free Ca2+ level in ovulated eggs was measured using Fluo-3 AM. (A) Ovulated egg arrested at M-II stage. (B) Egg underwent postovulatory aging-induced abortive SEA (EM-II). (C) Bars showing the CTCF analysis of three independent experiments. Values are mean ± SEM of three independent experiments. Data analyzed by Student's t-test, *P < 0.05.
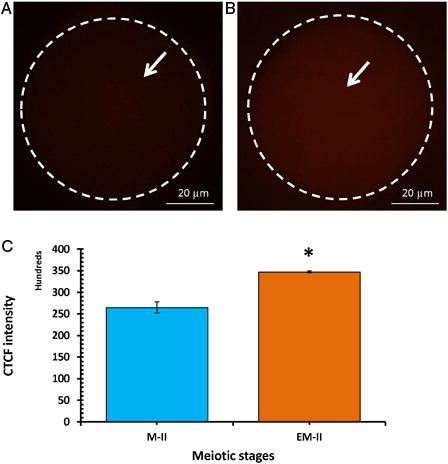
Postovulatory aging increases Wee1 level and decreases Cdc25B level
Wee1 kinase triggers Thr-14/Tyr-15 phosphorylation of Cdk1 and makes MPF inactive. Hence, we analyzed the level of Wee1. As shown in , postovulatory egg aging significantly increased Wee1 fluorescence intensity (4.6 folds) in eggs that underwent abortive SEA (EM-II) (B, white arrow) compared to control eggs (M-II) (A, white arrow). The CTCF analysis using Image J software further confirmed the above observations (C). On the other hand, a significant decrease of Cdc25B level was observed in eggs that underwent postovulatory aging-induced abortive SEA (B, arrow) compared to control eggs (M-II) (A, white arrow). The CTCF analysis using Image J software further confirmed the observations (C).
Figure 6. Representative photographs showing fluorescence intensity of Wee1 during postovulatory aging-induced abortive SEA. (A) Control egg (M-II). (B) Postovulatory aging increased Wee1 fluorescence intensity in eggs (EM-II). (C) Bars showing the CTCF analysis of fluorescence intensity of three independent experiments. Values are mean ± SEM of three independent experiments. Data analyzed by Student's t-test, *P < 0.001.
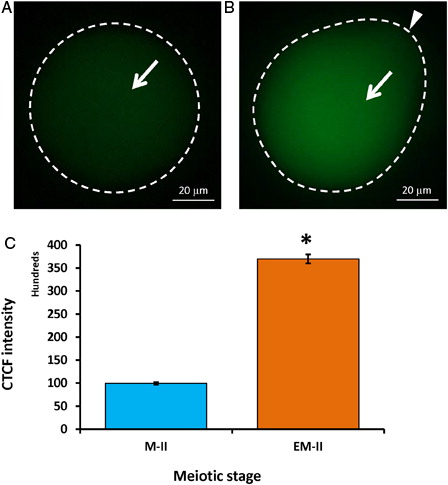
Figure 7. Representative photographs showing fluorescence intensity of Cdc25B during postovulatory aging-induced abortive SEA. (A) Control egg (M-II). (B) Postovulatory aging increased fluorescence intensity of Cdc25B in eggs (EM-II). (C) Bars showing the CTCF analysis of fluorescence intensity of three independent experiments. Values are mean ± SEM of three independent experiments. Data analyzed by Student's t-test, *P < 0.001.
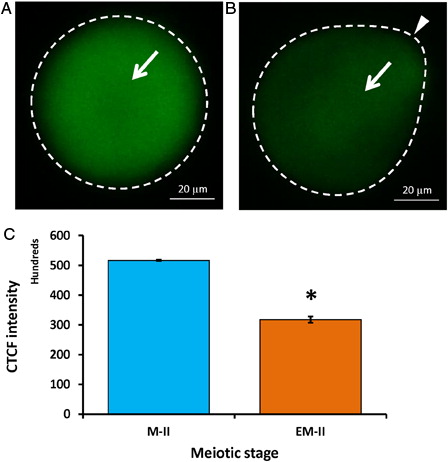
Postovulatory aging increases phosphorylated Cdk1 level
The decrease of Cdc25B level affects the phosphorylation status of Cdk1. Hence, we analyzed total (PSTAIR) as well as specific phosphorylation status of Cdk1 (pThr-14/Tyr-15) using their respective specific antibodies. Our results suggest that postovulatory aging induced accumulation of phosphorylated Cdk1 (pThr-14/Tyr-15) in eggs that underwent abortive SEA (EM-II) (B, arrow) compared to control eggs (M-II) (A, arrow). These observations were further supported by CTCF analysis of fluorescence intensity (C). However, total Cdk1 (PSTAIR) remained unchanged during postovulatory egg aging-induced abortive SEA (EM-II) (B, arrow) compared to control eggs (M-II) (A, arrow). This observation was further supported by the CTCF analysis (C).
Figure 8. Representative photographs showing fluorescence intensity of phosphorylated Cdk1 (pThr-14/Tyr-15) during postovulatory aging-induced abortive SEA. (A) Control egg (M-II). (B) Postovulatory aging increased fluorescence intensity of phosphorylated Cdk1 (pThr-14/Tyr-15) in eggs (EM-II). (C) Bars showing the CTCF analysis of fluorescence intensity of three independent experiments. Values are mean ± SEM of three independent experiments. Data analyzed by Student's t-test, *P < 0.05.
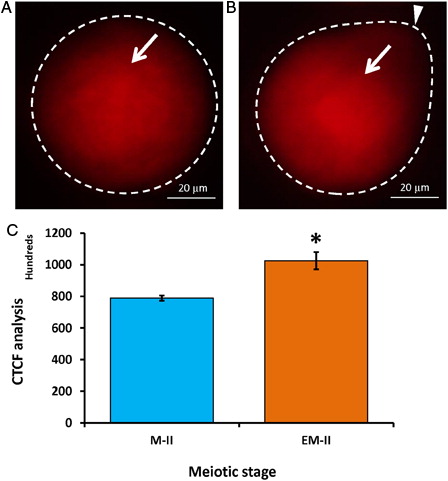
Figure 9. Representative photographs showing fluorescence intensity of Cdk1 (PSTAIRE) during postovulatory aging-induced abortive SEA. (A) Control egg (M-II). (B) Postovulatory aging increased fluorescence intensity of Cdk1 (PSTAIRE) in eggs (EM-II). (C) Bars showing CTCF analysis of fluorescence intensity of three independent experiments. Values are mean ± SEM of three independent experiments.
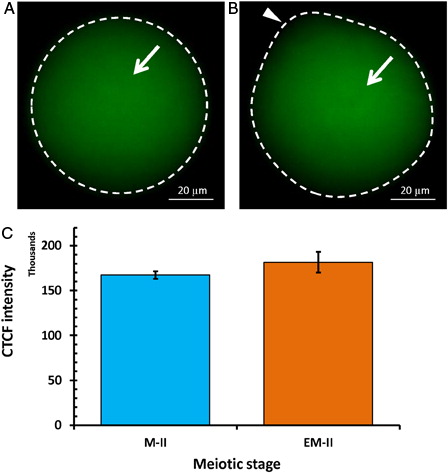
Discussion
The rat is an interesting animal model among mammals to study the regulation of the meiotic cell cycle in ovulated eggs. Unlike non-rodent mammalian species, diplotene-arrested rat oocytes resume meiosis and reach metaphase-I (M-I) stage and do not extrude the first polar body under in vitro culture conditions. Further, ovulated rat eggs undergo abortive exit from M-II arrest in vivo as well as in vitro.Citation1–Citation4 The reason why rat eggs undergo spontaneous meiotic exit from M-II arrest remains poorly understood. This atypical situation has also been observed in non-rodent species like buffaloCitation7, pig,Citation8 and human.Citation9 The abortive SEA limits ART outcome in these species. Hence, it is important to understand the role of these signaling molecules and their downstream impact on MPF level as well as meiotic cell cycle regulation. NO is one of the major signaling molecules that modulate ovarian functions, including the meiotic cell cycle, in mammals. iNOS expression and NO presence are necessary for meiotic resumption from diplotene arrest.Citation26,Citation27 Although a role of NO during meiotic resumption from diplotene arrest has been documented,Citation25,Citation26,Citation28–Citation31 its involvement during postovulatory aging-induced abortive SEA remains to be elucidated. This study reveals that postovulatory aging significantly increased iNOS expression. Quantitative analysis of NO level further revealed that NO level is significantly increased during postovulatory aging-induced abortive SEA.
The underlying mechanism by which NO signals abortive SEA remains poorly understood. There are two possibilities by which NO could mediate postovulatory aging-induced abortive SEA. The first possibility is that the NO can modify thiol groups in proteins (RyR channels) by S-nitrosylationCitation32–Citation34 that results in the mobilization of caged Ca2+ through RyR channels as demonstrated in skeletal musclesCitation17 and Purkinje cells.Citation18 The second possibility is that the increased level of NO can generate cGMP through the activation of guanyl cyclaseCitation35,Citation36 that results in the reduction of Cdc25B level via cAMP–PKA-mediated pathway.Citation37 Further, increased cGMP level may signal the inhibition of phosphatases (Cdc25B) that are involved in the maintenance of low MPF activity.Citation38
During fertilization, NO is sufficient for egg activation,Citation39 and NO donors induced egg activation by increasing the cytosolic free Ca2+ level.Citation40 Petr et al.Citation41 have also shown the involvement of NO in mobilizing Ca2+ through RyR channels during egg activation in pigs. It is reported that an insufficient increase of cytosolic free Ca2+ level through RyR channels results in abortive SEA.Citation4,Citation42 Based on these previous reports on somatic cells,Citation43,Citation44 we hypothesized that an increase of NO can modify the cysteine residues of RyR channels of internal stores and could lead to release of caged Ca2+. This hypothesis was further supported by our observation that increased cytosolic free Ca2+ level induced abortive SEA during postovulatory aging. These data further confirmed the role of RyR channels in the increase of cytosolic free Ca2+ level during postovulatory aging.Citation42 An increased cytosolic free Ca2+ may activate CaMK-II and active CaMK-II could increase Wee1 level during postovulatory aging-induced abortive SEA. Although CaMK-II activity was not analyzed in this study, previous studies on rat eggs suggest the involvement of CaMK-II activity during postovulatory egg aging-induced SEA.Citation45 These results suggest that NO is likely to modify RyR channels and thereby increase Wee1 level possibly by increasing cytosolic free Ca2+ during postovulatory aging-induced abortive SEA.
It is well established that cGMP is another major signaling molecule that regulates the meiotic cell cycle in mammalian eggs.Citation46 Studies suggest that an increase of cGMP induces the egg activation process in pigs.Citation15,Citation40 However, any role for cGMP during postovulatory aging-induced abortive SEA remained obscure. Our results suggest that an increase of intracellular cGMP triggers postovulatory aging-induced abortive SEA. The increased cGMP level may reduce Cdc25B level possibly through a PKA-dependent pathway.Citation19,Citation20 This possibility was further strengthened by our results that postovulatory aging reduced Cdc25B level in eggs that underwent abortive SEA. These results suggest that increased intracellular cGMP level reduces Cdc25B level, the activity of which is required for the dephosphorylation of Cdk1 (pThr-14/Tyr-15).
MPF is regulated by the phosphorylation of two highly conserved residues (pThr-14/Tyr-15) of Cdk1.Citation47 The phosphorylation is catalyzed by Wee1,Citation23 whereas dephosphorylation is mediated by Cdc25B (dual specificity phosphatases).Citation24 The decrease of Cdc25B level as well as an increase of Wee1 level could have led to the increase and/or maintenance of high phosphorylated Cdk1 (pThr-14/Tyr-15) level. This possibility was further supported by our results that postovulatory aging induced an accumulation of phosphorylated Cdk1 (pThr-14/Tyr-15) level, while total Cdk1 (PSTAIR) level did not change significantly. Similarly, changes in the phosphorylation status of Cdk1 have been reported during egg activation in mice.Citation48–Citation50
In summary, this study clearly suggests that an increase of NO, through iNOS-mediated pathway, induced the increase of cytosolic free Ca2+ level and raised cGMP level in rat eggs. The increased cGMP level induced accumulation of phosphorylated Cdk1 (pThr-14/Tyr-15) by reducing the Cdc25B level. An increase of cytosolic free Ca2+ level was associated with increased Wee1 that resulted in the accumulation of phosphorylated Cdk1 (pThr-14/Tyr-15). High phosphorylated Cdk1 (pThr-14/Tyr-15) level triggered postovulatory aging-induced abortive SEA in rat eggs cultured in vitro (). Our study clearly indicates the involvement of NO during postovulatory aging-induced abortive SEA in rat. We further emphasize that the use of iNOS inhibitors could block abortive SEA during postovulatory egg handling and maintain M-II arrest, so that a good number of eggs are available during various ART programs.
Figure 10. A schematic model showing the possible mechanism during postovulatory aging whereby increased NO induces phosphorylation at pThr-14/Tyr-15 of Cdk1 in the rat. NO operates RyR channels through nitrosylation and increases cytosolic free Ca2+ level. High cytosolic free Ca2+ level increases Wee1, possibly through CaMK-II, resulting in the accumulation of phosphorylated Cdk1 (pThr-14/Tyr-15). The high cGMP level results in the increase of Cdc25B level and accumulation of phosphorylated (pThr-14/Tyr-15) Cdk1. Hence, the increase of NO level leads to the accumulation of phosphorylated Cdk1 (pThr-14/Tyr-15) that signals postovulatory aging-induced abortive SEA in rat eggs cultured in vitro.
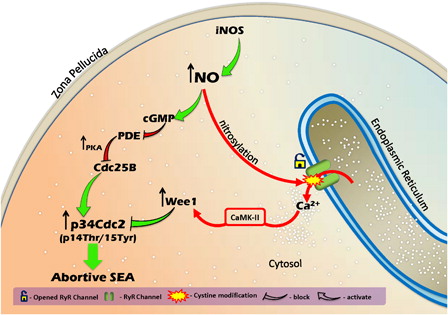
Acknowledgment
This study was financially supported by the Department of Biotechnology, Ministry of Science and Technology, Government of India.
Disclaimer statements
Contributors KVP performed all experiments under the guidance of SKC.
Funding None.
Conflicts of interest Authors declare no conflict of interest.
Ethics approval The experimental procedures used in the present study were approved by the Institutional Animal Ethical Committee of the University wide approval letter no. Dean/11–12/CAEC/266.
References
- Keefer CL, Schuetz AW. Spontaneous activation of ovulated rat oocytes during in vitro culture. J Exp Zool 1982;224:371–77.
- Zernika-Goetz M. Spontaneous and induced activation of rat oocytes. Mol Reprod Dev 1991;28:169–76.
- Ross PJ, Yabuuchi A, Cibelli JB. Oocyte spontaneous activation in different rat strains. Cloning Stem Cells 2006;8:275–82.
- Premkumar KV, Chaube SK. An insufficient increase of cytosolic free calcium level results postovulatory aging-induced abortive spontaneous egg activation in rat. J Assist Reprod Genet 2013;30:117–23.
- Fissore RA, Kurokawa M, Knott J, Zhang M, Smyth J. Mechanisms underlying oocyte activation and postovulatory ageing. Reproduction 2002;124:745–74.
- Kubiak JZ. Mouse oocytes gradually develop the capacity for activation during the metaphase II arrest. Dev Biol 1989;136:537–45.
- Sergeev IN, Norman AW. Calcium as a mediator of apoptosis in bovine oocytes and preimplantation embryos. Endocrine 2003;22:169–76.
- Ito J, Shimada M, Terada T. Effect of protein kinase C activator on mitogen-activated protein kinase and p34cdc2; kinase activity during parthenogenetic activation of porcine oocytes by calcium ionophore. Biol Reprod 2003;69:1675–82.
- Lu Q, Zhao Y, Gao X, Li Y, Ma S, Mullen S, et al. Combination of calcium ionophore A23187 with puromycin salvages human unfertilized oocytes after ICSI. Eur J Obstet Gynecol Reprod Biol 2006;126:72–76.
- Publicover NG, Hammond EM, Sanders KM. Amplification of nitric oxide signaling by interstitial cells isolated from canine colon. Proc Natl Acad Sci USA 1993;90:2087–91.
- Kong SK, Choy YM, Lee CY. The nitric oxide donor, sodium nitroprusside, increased intranuclear and cytosolic free calcium concentration in single PU5-1.8 cells. Biochem Biophys Res Commun 1994;199:234–40.
- Willmott NJ, Galione A, Smith PA. Nitric oxide induces intracellular Ca2+ mobilization and increases secretion of incorporated 5-hydroxytryptamine in rat pancreatic beta-cells. FEBS Lett 1995;371:99–04.
- Leckie C, Empson R, Becchetti A, Thomas J, Galione A, Whitaker M. The NO pathway acts late during the fertilization response in sea urchin eggs. J Biol Chem 2003;278:12247–54.
- Hyslop LA, Nixon VL, Levasseur M, Chapman F, Chiba K, McDougall K, et al. Ca2+-promoted cyclin B1 degradation in mouse oocytes requires the establishment of a metaphase arrest. Dev Biol 2004;269:206–19.
- Petr J, Rajmon Lanska V, Sedmikova M, Jilek F. Nitric oxide-dependent activation of pig oocytes: role of calcium. Mol Cell Endocrinol 2005;242:16–22.
- Wilmut I, Schnieke AE, McWhir J, Kind AJ, Campbell KHS. Viable offspring derived from fetal and adult mammalian cells. Nature 1997;385:810–13.
- Durham WJ, Aracena-Parks P, Long C, Rossi AE, Goonasekera SA, Boncompagni S, et al. Ryr1 S-nitrosylation underlies environmental heat stroke and sudden death in Y522s RyR1 knock in mice. Cell 2008;133:53–65.
- Kakizawa S, Yamazawa T, Chen Y, Ito A, Murayama T, Oyamada H, et al. Nitric oxide-induced calcium release via ryanodine receptors regulates neuronal function. EMBO J 2012;31:417–28.
- Sun QY, Miao YL, Schatten H. Towards a new understanding on the regulation of mammalian oocyte meiosis resumption. Cell Cycle 2009;8:2741–47.
- Solc P, Schultz RM, Motlik J. Prophase I arrest and progression to metaphase I in mouse oocytes: comparison of resumption of meiosis and recovery from G2-arrest in somatic cells. Mol Hum Reprod 2010;16:654–64.
- Hagting A, Jackman M, Simpson K, Pines J. Translocation of cyclin B1 to the nucleus at prophase requires a phosphorylation-dependent nuclear import signal. Curr Biol 1999;9:680–89.
- Timofeev O, Cizmecioglu O, Settele F, Kempf T, Hoffmann I. Cdc25 phosphatases are required for timely assembly of CDK1-cyclin B at the G2/M transition. J Biol Chem 2010;285:16978–90.
- Han SJ, Chen R, Paronetto MP, Conti M. Wee1B is an oocyte-specific kinase involved in the control of meiotic arrest in the mouse. Curr Biol 2005;15:1670–76.
- Lincoln AJ, Wickramasinghe D, Stein P, Schultz RM, Palko ME, De Miguel MP, et al. Cdc25b phosphatase is required for resumption of meiosis during oocyte maturation. Nat Genet 2002;30:446–49.
- Chaube SK, Tripathi A, Khatun S, Mishra SK, Prasad PV, Shrivastav TG. Extracellular calcium protects against verapamil-induced metaphase-II arrest and initiation of apoptosis in aged rat eggs. Cell Biol Int 2009;33:337–43.
- Bilodeau-Goeseels S. Effects of manipulating the nitric oxide/cyclic GMP pathway on bovine oocyte meiotic resumption in vitro. Theriogenology 2007;68:693–701.
- Viana KS, Caldas-Bussiere MC, Matta SGC, Faes MR, de Carvalho CSP, Quirino CR. Effect of sodium nitroprusside, a nitric oxide donor, on the in vitro maturation of bovine oocytes. Anim Reprod Sci 2007;102:217–27.
- Jablonka-Shariff A, Olson LM. Nitric oxide is essential for optimal meiotic maturation of murine cumulus-oocyte complexes in vitro. Mol Reprod Dev 2000;55:412–21.
- Kazuo SK, Naoyuki T, Michiharu HK, Keiko T, Harumi K, Dinara S, et al. Requirement of nitric oxide for murine oocyte maturation, embryo development, and trophoblast outgrowth in vitro. Mol Reprod Dev 2001;58:262–68.
- Bu S, Xia G, Tao Y, Lei L, Zhou B. Dual effects of nitric oxide on meiotic maturation of mouse cumulus cell-enclosed oocytes in vitro. Mol Cell Endocrinol 2003;207:21–30.
- Amidi F, Abbasi M, Akbari1 M, Sato E, Dehpour AR, Ejtemaei-Mehr S, et al. In vitro meiotic maturation of mouse oocytes: role of nitric oxide. Acta Medica Iranica 2007;45:55.
- Hess DT, Stamler JS. Regulation by S-nitrosylation of protein post-translational modification. J Biol Chem 2012;287:4411–18.
- Radi R, Peluffo G, Alvarez MN, Naviliat M, Cayota A. Unraveling peroxynitrite formation in biological systems. Free Radic Biol Med 2001;30:463–88.
- Shahani N, Sawa A. Nitric oxide signaling and nitrosative stress in neurons: role for S-nitrosylation. Antioxid Redox Signal 2011;14:1493–504.
- Nathan C. Nitric oxide as a secretory product of mammalian cells. FASEB J 1992;6:3051–64.
- Schmidt HW, Walter U. NO at work. Cell 1994;78:919–25.
- Pirino G, Wescott MP, Donovan PJ. Protein kinase A regulates resumption of meiosis by phosphorylation of Cdc25B in mammalian oocytes. Cell Cycle 2009;8:665–70.
- Wang S, Ning G, Chen X, Yang J, Ouyang H, Zhang H, et al. PDE5 modulates oocyte spontaneous maturation via cGMP–cAMP but not cGMP–PKG signaling. Front Biosci 2008;13:7087–95.
- Kuo RK, Baxter GT, Thompson SH, Stricker SA, Patton C, Bonaventura J, Epel D. NO is necessary and sufficient for egg activation at fertilization. Nature 2000;406:633–36.
- Petr J, Rajmon R, Chmelikova E, Tomanek M, Lanska V, Pribanova M, et al. Nitric oxide-dependent activation of pig oocytes: the role of the cGMP signalling pathway. Zygote 2006;14:9–16.
- Petr J, Chmelikova E, Dorflerova A, Jeseta M, Kuthanova Z. Effects of protein kinase C on parthenogenetic activation of pig oocytes using calcium ionophore or nitric oxide-donor. Czech J Anim Sci 2007;52:415–22.
- Premkumar KV, Chaube SK. RyR channel-mediated increase of cytosolic free calcium level signals cyclin B1 degradation during abortive spontaneous egg activation in rat. In Vitro Cell Dev Biol Anim 2014;50:640–47.
- Hidalgo C, Donoso P, Carrasco MA. The ryanodine receptors Ca2+ release channels: cellular redox sensors? IUBMB Life 2005;57:315–22.
- Willmott N, Sethi JK, Walseth TF, Lee HC, White AM, Galionei A. Nitric oxide-induced mobilization of intracellular calcium via the cyclic ADP-ribose signaling pathway. J Biol Chem 1996;271:3699–705.
- Yoo JC, Smith LC. Extracellular calcium induces activation of Ca2+/calmodulin dependent protein kinase II and mediates spontaneous activation in rat oocytes. Biochem Biophys Res Commun 2007;359:854–59.
- Yang CR, Wei Y, Qi ST, Chen L, Zhang QH, Ma JY, et al. The G protein coupled receptor 3 is involved in cAMP and cGMP signaling and maintenance of meiotic arrest in porcine oocytes. PLoS One 2012;7:e38807.
- Lew DJ, Kornbluth S. Regulatory roles of cyclin dependent kinase phosphorylation in cell cycle control. Curr Opin Cell Biol 1996;8:795–804.
- Strausfeld U, Labbe JC, Fesquet D, Cavadore JC, Picard A, Sadhu K, et al. Dephosphorylation and activation of a p34cdc2/cyclin B complex in vitro by human CDC25 protein. Nature 1991;351:242–45.
- Cui C, Zhao H, Zhang Z, Zong Z, Feng C, Zhang Y, et al. CDC25B acts as a potential target of PRKACA in fertilized mouse eggs. Biol Reprod 2008;79:991–98.
- Xiao J, Liu C, Hou J, Cui C, Wu D, Fan H, et al. Ser149 is another potential PKA phosphorylation target of Cdc25B in G2/M transition of fertilized mouse eggs. J Biol Chem 2011;286:10356–66.