Abstract
Objectives: Leukocyte NADPH oxidase, which is active in neutrophils, is a membrane-bound enzyme that catalyzes the reduction of oxygen to O2− by using NADPH as an electron donor. Previously, we reported that casein kinase 2 (CK2), a ubiquitous and highly conserved Ser/Thr kinase, is responsible for p47phox phosphorylation and that phosphorylation of p47phox by CK2 regulates the deactivation of NADPH oxidase.
Methods: Here, we report that the residue Cys196 of p47phox is a target of S-nitrosylation by S-nitrosothiol and peroxynitrite and that this modification enhanced phosphorylation of p47phox by CK2. Results: S-Nitrosylated p47phox enhanced CK2 b subunit binding, presumably due to alterations in protein conformation.
Discussion: Taken together, we propose that S-nitrosylation of p47phox regulates the deactivation of NADPH oxidase via enhancement of p47phox phosphorylation by CK2
Introduction
The NADPH oxidase of phagocytes is an important element of the host defense system against microbial infection, and it catalyzes the reduction of oxygen to superoxide anions (.) by using NADPH as the electron donor.Citation1 The oxidase is dormant in resting neutrophils but acquires catalytic activity when cells are exposed to the appropriate stimuli. Oxidase activity is located in the plasma membrane; however, in resting cells, the oxidase components are distributed between the membrane fraction and the cytosol. When activation occurs, the oxidase components p47phox and p67phox migrate to the membraneCitation2 where the p47phox–p67phox complex associates with the phagocyte-specific membrane-integrated flavocytochrome b558, a heterodimer of p22phox and gp91phox, to assemble the functioning oxidase.Citation3
The cytosolic oxidase component p47phox is a basic protein that becomes extensively phosphorylated when the oxidase is activated.Citation4 The phosphorylation target is known to be a group of serines (residues 303–379) in the highly basic carboxyl-terminal quarter of the peptide. Protein kinase C (PKC) has been suggested as a candidate kinase responsible for the phosphorylation of p47phox and activation of NADPH oxidase.Citation5
Although the activation mechanism of NADPH oxidase has been extensively investigated, little information is available about its mechanism of deactivation. Reactive species produced by active NADPH oxidase are not only bactericidal but also toxic to the surrounding host cells. Therefore, improper activation of NADPH oxidase must be prevented, and when an infection has been cleared, a rapid NADPH deactivation mechanism is imperative.Citation3 Previously, we reported that casein kinase 2 (CK2), a ubiquitous and highly conserved Ser/Thr kinase, is the p47phox kinase and that phosphorylation of p47phox by CK2 regulates deactivation of NADPH oxidase.Citation6
It has been reported that nitric oxide (NO) and the peroxynitrite (PN) anion (ONOO−) inhibit generation of the superoxide anion by neutrophils.Citation7,Citation8 Since NO reacts with reduced thiol to form S-nitrosothiol, the focus on the biological effects of NO has expanded to include RSNO. RSNO is biologically active and significantly more stable than NO; therefore, this adduct has been proposed as a biologically active intermediate of NO.Citation9 In addition, PN is a potent oxidant generated from the interaction of NO and .Citation10 At physiological concentrations, NO is the only known biological molecule that can out-compete endogenous superoxide dismutase for available
,Citation11 and formation of PN mediates both
and NO-dependent biological effects.Citation12
In this communication, we provide evidence that S-nitrosylation of p47phox at Cys196, catalyzed by S-nitrosothiol and PN, enhanced p47phox phosphorylation by CK2, presumably, via a p47phox protein conformational change. These results help elucidate a possible mechanism underlying the regulation of NADPH oxidase deactivation via S-nitrosylation of p47phox.
Materials and methods
Materials
The pGEX-1lambdaT vector was purchased from Pharmacia (Uppsala, Sweden). Glutathione (GSH), GSH–agarose, carboxymethyl (CM)–Sepharose, dithiothreitol (DTT), S-nitroso-N-acetylpenicillamine (SNAP), and human plasma thrombin were obtained from Sigma (St Louis, MO, USA). Antibodies were obtained from Santa Cruz Biotechnology (Santa Cruz, CA, USA). PN was synthesized from hydrogen peroxide and isoamyl nitrite, as described previously.Citation13 Contaminating hydrogen peroxide was eliminated with manganese dioxide, and PN concentration was determined at 302 nm (epsilon = 1.67/(mm cm)).
Site-directed mutagenesis and preparation of recombinant proteins
The mutations C98A, C111A, C196A, and C398A were constructed by single-strand mutagenesis of wild type p47phox.Citation14 DNA fragments encoding p47phox–SH3 (amino acid residues 154–285) were obtained as previously described.Citation15 The transformed Escherichia coli containing the pGEX-1lambdaT plasmid with either the p47phox cDNA or mutant cDNA insert was cultured, and the fusion protein was purified by affinity chromatography on GSH–agarose, as previously described.Citation16 The fusion protein was cleaved by treatment with thrombin (10 U/ml) in elution buffer containing 150 mM NaCl and 2.5 mM CaCl2 for 2 hours at room temperature. The protein mixture of recombinant p47phox was further purified on a CM–Sepharose column equilibrated with 5 mM phosphate buffer (pH 7.0) containing 0.1 mM PMSF and was eluted with a 40-ml gradient of 0–0.4 M NaCl in the same buffer. The concentration of proteins was determined with the Bio-Rad assay kit (Hercules, CA, USA), using bovine serum albumin (BSA) as a standard.
Purification of CK2
Human CK2 holoenzyme was purified to homogeneity via four chromatography steps, using DEAE-cellulose, phosphocellulose, heparin–agarose, and gel filtration, from the lysate of E. coli that expressed both alpha and beta subunits of CK2.Citation6
S-Nitrosylation of p47phox
Recombinant p47phox (1 μg) in 40 mM Tris–HCl (pH 8.0) was incubated with various concentrations of SNAP or PN at room temperature for 30 minutes. Samples were subjected to SDS–polyacrylamide gel (PAGE) and immunoblotting with an anti-nitrocysteine IgG antibody.
Phosphorylation of p47phox by CK2
Phosphorylation of recombinant p47phox by CK2 was performed in a reaction mixture containing 20 mM Tris–HCl (pH 7.5) with 100 mM KCl, 10 mM MgCl2, 1 mM DTT, 1 mM EGTA, 0.1 mM ATP, 5 μCi of [gamma-32P]ATP, and 1 μg of p47phox in a total volume of 30 μl, unless otherwise indicated. The reactions were initiated by addition of purified CK2 and incubated for 15 minutes at 30°C. After terminating the phosphorylation reactions by addition of 10 μl of 4× SDS-sample buffer, the samples were subjected to SDS–PAGE, using an 8% running gel. The labeled proteins were visualized by autoradiography.
Immunoblot analysis
The proteins were separated on a 10% SDS– polyacrylamide gel, transferred to nitrocellulose membranes, and then subjected to immunoblotting with appropriate antibodies. Primary antibodies were then incubated with horseradish peroxidase (HRP)-labeled anti-rabbit IgG and detected with an enhanced chemiluminescence detection kit (Amersham Pharmacia Biotech, UK).
After spotting S-nitrosylated p47phox recombinant proteins onto a nitrocellulose membrane, the membrane was blocked in intracellular buffer (IB; 110 mm KCl, 5 mm NaHCO3, 5 mm MgCl2, 1 mm EGTA, 0.1 mm CaCl2, 20 mm Hepes, 1 mm dithiothreitol, and 5 μg/mL leupeptin, pH 7.4) containing 5% BSA for 4 h at 22°C. Hybridization was performed for 16 h at 4°C in IB/1% BSA supplemented with the recombinant CK2 b subunit. The membrane was extensively washed, and the bound CK2 b subunit was detected using a CK2 b-specific IgG antibody.
Measurement of S-nitrosylation
Determination of S-nitrosylated proteins with 2,3-diaminonaphthalene (DAN) assay was carried out, as previously described.Citation8 S-Nitrosylated p47phox recombinant proteins with 1 mM SNAP or 1 mM PN (30 minutes, room temperature) were incubated with 100 μM HgCl2 and 100 μM DAN for 30 minutes at room temperature, following which 1 M NaOH was added. The quantity of fluorescent triazole generated from the reaction between DAN and NO released from S-nitrosylated p47phox was monitored by a fluorometer (Perkin-Elmer HTS 7000, Perkin-Elmer, Boston, MA, USA) with an excitation wavelength of 375 nm and an emission wavelength of 450 nm. The fluorescence intensity generated in the absence of HgCl2 was then subtracted from the fluorescence intensity generated in the presence of HgCl2. Fluorescence intensity above background was normalized to the amount of protein.
Structural analysis
Steady-state intrinsic fluorescence measurements were performed using a Shimadzu RF-5301 PC spectrofluorophotometer with the sample compartment maintained at 22°C. A 150-W xenon light source was used. The slit-width was fixed at 5 nm for excitation and emission. Samples were subjected to excitation at 278 nm, and the emission was monitored between 300 and 400 nm.
Results and discussion
Regulation of protein properties and function by post-translational modification is the central molecular mechanism that mediates signal transduction.Citation17 Proteins containing cysteine residues are susceptible to protein S-nitrosylation, mediating ubiquitous influence of NO and PN on various biological functions in eukaryotic cells.Citation17. In this study, we present evidence indicating that recombinant p47phox is modified by S-nitrosylation. A previous study demonstrated that a polyclonal anti-nitrosocystein antibody could be used to detect S-nitrosylation.Citation13 As shown in A, immunoblot analysis of SNAP- or PN-treated p47phox using this anti-nitrocysteine antibody revealed dose-dependent increases in S-nitrosocysteine expression, thus confirming the S-nitrosylation of cysteine residues in p47phox. Wild type p47phox contains four cysteine residues at positions 98, 111, 196, and 378. Although a previous study proposed that none of the four cysteines in p47phox is indispensable for protein function,Citation14 the regulatory role of these residues has not yet been evaluated. To determine that the p47phox cysteine residue is the putative target for S-nitrosylation, mutants were created in which each one of the four codons encoding a cysteine residue was converted to an alanine. S-Nitrosylation of p47phox was assessed using the DAN assay in controls without addition of SNAP or PN, wild type, and mutant samples. A significant increase in DAN fluorescence was observed in wild type, C98A, C111A, and C396A p47phox mutants compared to the control sample (B). However, no significant increase in DAN fluorescence was observed in the C196A p47phox mutant compared to the control sample, indicating that Cys196 is a target of p47phox S-nitrosylation. These results were further confirmed with immunoblot analysis of SNAP- and PN-treated p47phox C196A, using the anti-nitrocysteine antibody (C).
Figure 1. S-Nitrosylation of p47phox. (A) Immunoblot analysis of recombinant p47phox. After incubating p47phox with various concentrations of SNAP or PN for 30 minutes at room temperature, the samples were subjected to SDS–PAGE and immunoblotting with the anti-nitrocysteine IgG antibody. (B) Identification of the S-nitrosylation target cysteine in p47phox by using site-directed mutagenesis. The S-nitrosylation of wild type and p47phox C→A mutants was detected using the DAN reagent. The fluorescence signal of the untreated control protein was subtracted as background. Values correspond to three independent assays with the standard deviation indicated. *P < 0.01 versus wild type, C98A, C111A, and C396 p47phox mutant proteins. (C) After incubation with 1 mM SNAP or 1 mM PN for 30 minutes at room temperature, the wild type and the p47phox C196A mutant were subjected to SDS–PAGE, followed by immunoblotting with the anti-nitrocysteine IgG antibody.
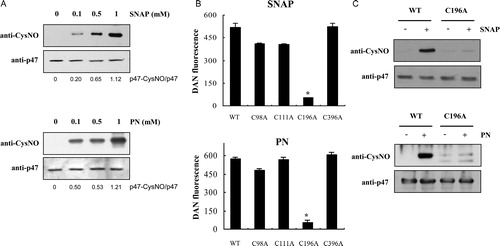
CK2 is a ubiquitous and highly conserved Ser/Thr kinase which is found in multiple subcellular compartments of all eukaryotes examined.Citation18 CK2 is a heterodimer comprised of two catalytic (alpha and/or alpha′) and two regulatory (beta) subunits. It has been shown that the alpha and alpha′ subunits are different gene products and retain the catalytic activity of the enzyme. The beta subunit is a regulatory subunit that modulates the catalytic activity of the alpha subunit and mediates tetramer formation and substrate recognition.Citation19 Although its precise physiological role and regulatory mechanism remain largely unknown, CK2 is known to phosphorylate a broad spectrum of substrates. We have shown that CK2 catalyzes the phosphorylation of p47phox, and the phosphorylation of p47phox by CK2 is one of the mechanisms regulating NADPH oxidase deactivation.Citation6 As shown in A, when p47phox was incubated with CK2 in the presence of [gamma-32P]ATP, the increase of a single, and heavily phosphorylated band was observed in a concentration-dependent manner, after treatment with PN or SNAP. Decomposed PN or SNAP did not enhance phosphorylation of p47phox by CK2. In addition, molecules known to inhibit PN-dependent modification of cysteine residues such as DTT abolished enhanced p47phox phosphorylation (B). Enhanced p47phox phosphorylation by CK2 after S-nitrosylation was not observed in the p47phox C196A mutant, indicating that S-nitrosylation of Cys196 is critical for the regulation of CK2-mediated p47phox phosphorylation (C). Although the identity of the kinases that phosphorylate p47phox in vivo has not been clearly established, PKC is one of the enzymes capable of converting p47phox into a functionally active molecule in intact neutrophil cellsCitation5 as well as in a cell-free system.Citation20 In contrast to p47phox phosphorylation by CK2, S-nitrosylation did not change PKC-mediated p47phox phosphorylation (D).
Figure 2. Effect of S-nitrosylation on in vitro phosphorylation of recombinant p47phox by CK2. (A) Recombinant p47phox (1 μg) was incubated with various concentrations of SNAP or PN (30 minutes at room temperature), followed by incubation with [gamma-32P]ATP and CK2. The labeled proteins were separated by SDS–PAGE. Proteins were visualized using the anti-p47phox IgG antibody, and 32P incorporation (p-p47phox) was monitored by autoradiography. (B) Effect of 1 mM DTT and degraded SNAP or PN on the phosphorylation of S-nitrosylated p47phox by CK2. (C) SNAP- or PN-treated wild type and p47phox C196A mutant samples were phosphorylated by CK2 with [gamma-32P]ATP. The labeled proteins were separated by SDS–PAGE, and 32P incorporation was monitored by autoradiography. (D) Recombinant p47phox (1 μg) was incubated with various concentrations of PN (30 minutes at room temperature) and then with [gamma-32P]ATP and PKC. The labeled proteins were separated by SDS–PAGE. Proteins were visualized with anti-p47phox IgG, and 32P incorporation was monitored by autoradiography.
![Figure 2. Effect of S-nitrosylation on in vitro phosphorylation of recombinant p47phox by CK2. (A) Recombinant p47phox (1 μg) was incubated with various concentrations of SNAP or PN (30 minutes at room temperature), followed by incubation with [gamma-32P]ATP and CK2. The labeled proteins were separated by SDS–PAGE. Proteins were visualized using the anti-p47phox IgG antibody, and 32P incorporation (p-p47phox) was monitored by autoradiography. (B) Effect of 1 mM DTT and degraded SNAP or PN on the phosphorylation of S-nitrosylated p47phox by CK2. (C) SNAP- or PN-treated wild type and p47phox C196A mutant samples were phosphorylated by CK2 with [gamma-32P]ATP. The labeled proteins were separated by SDS–PAGE, and 32P incorporation was monitored by autoradiography. (D) Recombinant p47phox (1 μg) was incubated with various concentrations of PN (30 minutes at room temperature) and then with [gamma-32P]ATP and PKC. The labeled proteins were separated by SDS–PAGE. Proteins were visualized with anti-p47phox IgG, and 32P incorporation was monitored by autoradiography.](/cms/asset/f455bb9a-d212-49ef-a769-44000c66fa62/yrer_a_11646844_f0002_b.jpg)
Previously, we demonstrated that CK2 phosphorylation sites are localized in p47phox–SH3 domains (amino acid residues of 154–285).Citation6 In contrast, PKC phosphorylation sites of p47phox reside at the C-terminus (residues 303–379).Citation5 The two SH3 domains are normally masked by an intramolecular interaction, with an autoinhibitory region residing at the C-terminus of the p47phox protein.Citation21 In contrast to many kinases responsible for p47phox phosphorylation, CK2 is not known to respond to secondary messengers. The present study demonstrates a significant increase in p47phox–SH3 phosphorylation by CK2 (A). However, this phosphorylation was not modulated by S-nitrosylation (B), indicating that S-nitrosylation may induce a conformational change in wild type p47phox. Modification of protein thiols may affect protein conformation.Citation17 Indeed, modulation of the intrinsic fluorescence of S-nitrosylated p47phox indicates a structural alteration. We previously developed a fluorescence spectroscopy approach to monitor conformational changes in p47phox.Citation22 Upon excitation of native p47phox at 278 nm, a fluorescence emission spectrum typical for tryptophan residues, with a maximum emission at 338 nm, was observed. The intrinsic fluorescence of wild type p47phox was significantly quenched by S-nitrosylation, whereas the fluorescence of p47phox–SH3 was not altered by S-nitrosylation (C). The effect of the S-nitrosylation-induced conformational changes in p47phox on the binding affinity of recombinant p47phox to the CK2-beta subunit was evaluated by the dot blot technique. Results indicated that S-nitrosylation of p47phox enhanced binding of p47phox and the CK2 beta subunit, which is consistent with increased CK2 phosphorylation by S-nitrosylation (D). This is interesting, as Cys196 is the only cysteine located within the SH3 domain. Cys196, therefore, seems to be a critical target for preventing protein–protein interactions necessary for NADPH oxidase deactivation.
Figure 3. Conformational change in p47phox protein by S-nitrosylation. (A) Recombinant wild type and SH3 domains of p47phox (1 μg) were incubated with [gamma-32P]ATP and CK2. The labeled proteins were separated by SDS–PAGE. Proteins were visualized using the anti-p47phox IgG antibody, and 32P incorporation was monitored by autoradiography. (B) The SH3 domain of p47phox (1 μg) was incubated with PN (30 minutes at room temperature) and then with [gamma-32P]ATP and CK2. The labeled proteins were separated by SDS–PAGE. Proteins were visualized using the anti-p47phox IgG antibody, and 32P incorporation (p-SH3) was monitored by autoradiography. (C) Changes in p47phox structure following S-nitrosylation. Steady-state emission spectra of intrinsic fluorescence of native p47phox and SH3 domains treated with SNAP or PN were analyzed with a spectrophotometer at an excitation wavelength of 278 nm. The maximum protein fluorescence intensity was determined at 338 nm. Data are representative of three experiments. *P < 0.01 versus untreated p47phox. (D) Visualization of binding of CK2-beta subunit to S-nitrosylated p47phox using dot blot analysis.
![Figure 3. Conformational change in p47phox protein by S-nitrosylation. (A) Recombinant wild type and SH3 domains of p47phox (1 μg) were incubated with [gamma-32P]ATP and CK2. The labeled proteins were separated by SDS–PAGE. Proteins were visualized using the anti-p47phox IgG antibody, and 32P incorporation was monitored by autoradiography. (B) The SH3 domain of p47phox (1 μg) was incubated with PN (30 minutes at room temperature) and then with [gamma-32P]ATP and CK2. The labeled proteins were separated by SDS–PAGE. Proteins were visualized using the anti-p47phox IgG antibody, and 32P incorporation (p-SH3) was monitored by autoradiography. (C) Changes in p47phox structure following S-nitrosylation. Steady-state emission spectra of intrinsic fluorescence of native p47phox and SH3 domains treated with SNAP or PN were analyzed with a spectrophotometer at an excitation wavelength of 278 nm. The maximum protein fluorescence intensity was determined at 338 nm. Data are representative of three experiments. *P < 0.01 versus untreated p47phox. (D) Visualization of binding of CK2-beta subunit to S-nitrosylated p47phox using dot blot analysis.](/cms/asset/4087fd2e-7165-42f4-8175-5a5e2420df3d/yrer_a_11646844_f0003_b.jpg)
In this communication, we provide evidence that S-nitrosylation of p47phox at Cys196 by RSNO or PN enhanced p47phox phosphorylation by CK2, presumably, via a conformational change in p47phox that increased binding affinity between nitrosylated p47phox and the CK2-beta subunit (). These results help elucidate a possible mechanism underlying the regulation of NADPH oxidase deactivation via S-nitrosylation of p47phox. A mechanistic understanding of how S-nitrosylated Cys196 in p47phox leads to enhanced CK2 binding will likely be provided by determining the X-ray crystal structure. In addition, the effect of p47phox post-translational modification on the in vivo regulation of NADPH oxidase deactivation warrants further investigation.
Acknowledgments
This work was supported by the National Research Foundation of Korea (NRF) grants funded by the Korean government (2014001483 and 2014023638). We are grateful to the late B.M. Babior for help and advice.
Disclaimer statements
Contributors All authors contributed equally.
Funding None.
Conflicts of interest None.
Ethics approval None.
References
- Babior BM. The respiratory burst oxidase. Adv Enzymol Relat Areas Mol Biol 1992;65:49–95.
- Park JW, Ma M, Ruedi JM, Smith RM, Babior BM. The cytosolic components of the respiratory burst oxidase exist as a M(r) approximately 240,000 complex that acquires a membrane-binding site during activation of the oxidase in a cell-free system. J Biol Chem 1992;267:17327–32.
- Brandes RP, Weissmann N, Schroder K. Nox family NADPH oxidases: molecular mechanisms of activation. Free Radic Biol Med 2014;76:208–26.
- Rotrosen D, Leto TL. Phosphorylation of neutrophil 47-kDa cytosolic oxidase factor. Translocation to membrane is associated with distinct phosphorylation events. J Biol Chem 1990;265:19910–5.
- El Benna J, Faust LP, Babior BM. The phosphorylation of the respiratory burst oxidase component p47phox during neutrophil activation. Phosphorylation of sites recognized by protein kinase C and by proline-directed kinases. J Biol Chem 1994;269:23431–6.
- Park HS, Lee SM, Lee JH, Kim YS, Bae YS, Park JW. Phosphorylation of the leucocyte NADPH oxidase subunit p47phox by casein kinase 2: conformation-dependent phosphorylation and modulation of oxidase activity. Biochem J 2001;358:783–90.
- Clancy RM, Levartovsky D, Leszczynska-Piziak J, Yegudin J, Abramson SB. Nitric oxide reacts with intracellular glutathione and activates the hexose monophosphate shunt in human neutrophils: evidence for S-nitrosoglutathione as a bioactive intermediary. Proc Natl Acad Sci U S A 1994;91:3680–4.
- Selemidis S, Dusting GJ, Peshavariya H, Kemp-Harper BK, Drummond GR. Nitric oxide suppresses NADPH oxidase-dependent superoxide production by S-nitrosylation in human endothelial cells. Cardiovasc Res 2007;75:349–58.
- Park JW. Attenuation of p47phox and p67phox membrane translocation as the inhibitory mechanism of S-nitrosothiol on the respiratory burst oxidase in human neutrophils. Biochem Biophys Res Commun 1996;220:31–5.
- Beckman JS, Beckman TW, Chen J, Marshall PA, Freeman BA. Apparent hydroxyl radical production by peroxynitrite: implications for endothelial injury from nitric oxide and superoxide. Proc Natl Acad Sci U S A 1990;87:1620–4.
- Beckman JS, Carson M, Smith CD, Koppenol WH. ALS, SOD and peroxynitrite. Nature 1993;364:584.
- Pryor WA, Squadrito GL. The chemistry of peroxynitrite: a product from the reaction of nitric oxide with superoxide. Am J Physiol Lung Cell Mol Physiol 1995;268:L699–722.
- Lee JH, Yang ES, Park JW. Inactivation of NADP+-dependent isocitrate dehydrogenase by peroxynitrite. Implications for cytotoxicity and alcohol-induced liver injury. J Biol Chem 2003;278:51360–71.
- Inanami O, Johnson JL, Babior BM. The leukocyte NADPH oxidase subunit p47phox: the role of the cysteine residues. Arch Biochem Biophys 1998;350:36–40.
- Lee JH, Lee KS, Chung T, Park JW. C-terminal region of the cytosolic subunit p47phox is a primary target of conformational change during the activation of leukocyte NADPH oxidase. Biochimie 2000;82:727–32.
- Park JW, Babior BM. Activation of the leukocyte NADPH oxidase subunit p47phox by protein kinase C. A phosphorylation-dependent change in the conformation of the C-terminal end of p47phox. Biochemistry 1997;36:7474–80.
- Hess DT, Stamler JS. Regulation by S-nitrosylation of protein post-translational modification. J Biol Chem 2012;287:4411–8.
- Issinger OG. Casein kinases: pleiotropic mediators of cellular regulation. Pharmacol Ther 1993;59:1–30.
- Gietz RD, Graham C, Litchfield DW. Interactions between the subunits of casein kinase II. J Biol Chem 1995;270:13017–21.
- Park JW, Hoyal CR, El Benna J, Babior BM. Kinase-dependent activation of the leukocyte NADPH oxidase in a cell-free system. Phosphorylation of membranes and p47phox during oxidase activation. J Biol Chem 1997;272:11035–43.
- Yuzawa S, Ogura K, Horiuchi M, Suzuki N, Fujioka Y, Kataoka M, et al. Solution structure of the tandem Src homology 3 domains of p47phox in an autoinhibited form. J Biol Chem 2004;279:29752–60.
- Park HS, Park JW. Conformational changes of the leukocyte NADPH oxidase subunit p47phox during activation studied through its intrinsic fluorescence. Biochim Biophys Acta 1998;1387:406–14.