Abstract
Objectives
Ribosomal protein S14 (RPS14) plays a key role in erythropoiesis and causes p53 activation in 5q- syndrome. However, the oncogenic potential of RPS14 is not understood in leukemia and high-risk myelodysplastic syndrome (MDS). Here, we investigated the changes of proliferation and apoptosis of SKM-1, an acute myeloid leukemia (MDS/AML) cell line transformed from MDS, and explored the role of RPS14 in them.
Methods
SKM-1 cells were transfected with recombined lentiviral vector shRPS14. Reverse-transcribed polymerase chain reaction and western blot assay were carried to detect the expression of RPS14 and p53. Cell proliferation was determined by MTT assay. Cell cycle and apoptosis were detected through flow cytometry.
Results
When compared with negative control, the proliferation rate of SKM-1 cells transfected with RPS14 hairpin siRNA dropped by 30%. Transfected SKM-1 cells presented with activation of p53. Transfection also arrested cells in G0/G1 phase and induced apoptosis, indicating that RPS14 is involved in the pathophysiology of MDS/AML.
Discussion
These findings indicate that partial silencing of RPS14 inhibits the proliferation of MDS/AML cells, and RPS14 may negatively regulate p53 activation in MDS/AML cells.
Introduction
RPS14, a ribosomal gene encoding the ribosomal subunit 40S, is required for the processing of 18S pre-rRNA. It is mapped to the commonly deleted region (CDR) in the 5q- syndrome, the most distinct subtype with good prognosis of myelodysplastic syndrome (MDS), which is characterized by 5q deletion as the sole karyotypic abnormality.Citation1 Haploinsufficiency of ribosomal protein S14 (RPS14) has recently been identified as a causal factor in the 5q- syndrome.Citation2 Recent research on RPS14 mainly focused on its role in the 5q- syndrome and erythropoiesis. Over-expression of RPS14 in cells from MDS patients with 5q deletion may rescue erythropoiesis.Citation2 Furthermore, haploinsufficiency of CDR causes macrocytic anemia and prominent erythroid dysplasia in the bone marrow of a mouse model of the 5q- syndrome.Citation3 However, the role of RPS14 in patients with 5q deletion and multiple cytogenetic abnormalities which are classified as high-risk MDS due to poor prognosis and high risk for transforming into acute myeloid leukemia (AML) remains unclear, and whether RPS14 plays the same role in the 5q- syndrome and high-risk MDS remains to be elucidated. Low expression of RPS14 had been found in MDS patients without concurrent cytogenetic aberrations on chromosome 5, who usually had prolonged survival.Citation4 It was indicated that low RPS14 expression might be a predictor of good prognosis in MDS or MDS transformed AML patients. Thus, it is imperative to clarify the role of RPS14 in progression of high-risk MDS to AML.
The tumor suppressor p53 is one of the most intensely studied proteins in oncology. Studies have shown that 50–70% of human tumors present with p53 gene mutations.Citation4,Citation5 Furthermore, most of the remaining tumors that have no p53 gene mutations display amplifications or deletions of other genes causing p53 functional inactivation.Citation6,Citation7 P53 network is the most important pathway involved in preventing the initiation of cancers. It has been shown that p53 pathway plays a key role in the hematopoietic effects of ribosomal gene haploinsufficiency.Citation8 Activated p53 initiates different transcriptional programs resulting in cell cycle arrest, cellular senescence, or apoptosis.Citation9,Citation10 In murine and zebrafish models, ribosomal gene dysfunction causes defective erythropoiesis, and red blood cell production is normalized in the absence of p53.Citation11 In the 5q- syndrome, RPS14 gene silencing causes macrocytic anemia, and the hematopoietic phenotype can be rescued in mice with homozygous knockout of p53 gene.Citation3 Thus, we hypothesize that the role of RPS14 in AML transformed from MDS is related with p53.
In the present study, the expression of RPS14 and p53 was detected in human MDS/AML cell line (SKM-1 cells), and RPS14 gene silencing was done to explore the role of RPS14 in MDS transformed AML. Proliferation and apoptosis of SKM-1 cells after RPS14 gene silencing were investigated in order to gain more insight of the role of RPS14 in AML transformed from MDS.
Materials and methods
Materials
SKM-1 cells were kindly provided by Prof. Jianfeng Zhou in Tongji Medical College of Huazhong University of Science and Technology, and maintained in RPMI-1640 (HyClone, Logan, UT, USA) supplemented with 10% heat-inactivated fetal bovine serum (Gibco, Camarillo, California, USA). Antibody against RPS14 was purchased from Abcam (Cambridge, UK), and antibody against P53 from Beyotine (Shanghai, China).
Lentiviral vectors and infection
Lentiviral short hairpin RNAs (shRNAs) in pGC-SIL-GFP vector were obtained from Genechem Ltd (Shanghai, China). Sequences targeted by each shRNA were listed in . Lentivirus was produced in 293T cells as described previously.Citation12 Human MDS/AML cell line, SKM-1 cells, were infected with lentivirus 1 day after being thawed in the presence of 8 µg/ml polybrene (Sigma-Aldrich, St-Louis, MO, USA) and screened 24 hours later with 2 µg/ml puromycin (Sigma-Aldrich).
Table 1. Targeted sequences of shRNA of RPS14
Reverse-transcribed polymerase chain reaction
RNA isolation and cDNA synthesis were carried out according to manufacturer's instructions (Takara, Mountain View, CA, USA). Primers for RPS14 and β-actin were as follows: RPS14 ATGTTGGCTGCCCAG (forward), GGTCTTGGTCCTATTTCC TC (reverse); β-actin TCC ATG ACA ACT TTG GTA TCG (forward), GTCGCTGTTGAAGT C AGAGGA (reverse). Polymerase chain reaction (PCR) conditions were as follows: 95°C for 5 minutes, followed by 30 cycles of 95°C for 30 seconds, 58°C for 60 seconds, and 72°C for 60 seconds. PCR products were subjected to gel electrophoresis. The relative expression of RPS14 was quantitatively measured using densitometry.
Western blot assay
Western blot assay was performed using 50 ng of total proteins and primary antibodies against p53 (Beyotine, China) at 1:1000, RPS14 (Abcam, UK) at 1:5000, and GAPDH (Biao, Beijing, China) at 1:1000. Proteins were separated on 12% sodium dodecyl sulfate–polyacrylamide gel electrophoresis gel and then transferred onto polyvinylidene difluoride (PVDF) membrane (Millipore, Billerica, MA, USA) at 110 mA for 2 hours. The PVDF membrane was incubated with rabbit anti-RPS14 polyclonal antibody (Abcam), and then with secondary Abs goat anti-rabbit IgG conjugated with HRP (BioWorld, Wuhan, China). Visualization was done with ECL kit (BioWorld, China).
Quantitative real-time reverse-transcribed polymerase chain reaction
RNA was purified, and cDNA was generated using 200 ng of total RNA and oligo dT primers with reverse transcription kit (Takara, USA). Quantitative reverse-transcribed PCR (RT-PCR) was performed using SYBR Green supermix (Invitrogen, Camarillo, California, USA) in an iQ5 Cycler. β-Actin served as an internal control. Relative changes in mRNA expression were calculated using 2△△Ct method.
MTT assay
Cell survival was determined by 3-(4, 5-dimethylthiazol-2-yl-2, 5-diphenyltetrazolium (MTT) (Sigma, Santa Clara, CA, USA) assay. Around 104 cells/200 µl was seeded into 96-well plate and incubated for 24, 48, and 72 hours. After addition of 20 µl of 5 mg/ml MTT solution, incubation was done for 4 hours. Medium was then replaced with 150 µl of dimethyl sulfoxide to dissolve crystals. Cell viability was determined by measuring absorbance (A) at 490 nm. The inhibition rate of cell growth was calculated by the formula as follows: inhibition rate (%) = (1 − Atreated group/Auntreated group) × 100%.
Cell cycle analysis
Cells were infected with RPS14 siRNA lentivirus and negative control lentivirus in complete medium for 10 hours. Cells were harvested and stained with BrdU and 7-aminoactinomycin D (7-AAD) according to the manufacturers' instructions (BrdU flow kits; BD Biosciences PharMingen, San Jose, California, USA). Cells in G0/G1 phase, S phase, and G2/M phase were determined by flow cytometry.
Cell apoptosis analysis
Apoptosis was evaluated using an antibody against annexin V (BD Biosciences PharMingen) by flow cytometry.
Statistical analysis
Data were expressed as means ± standard deviation. Comparisons were done with one-way analysis of variance. A value of P < 0.05 was considered statistically significant. Statistical analysis was carried out with SPSS 17.0 for Windows.
Results
Construction of lentiviral vector encoding shRNA against RPS14 and its expression in SKM-1 cells
SKM-1 cells were infected with RPS14-shRNA lentivirus. Proportion of infected SKM-1 cells was detected by fluorescence-activated cell sorting (FACS). Results showed that the transfection efficiency was 68.41% (A–C). Then, RPS14 protein expression was detected by western blot assay in SKM-1 cells. RNAi targeting RPS14 significantly inhibited the protein expression of RPS14 in SKM-1 cells as compared with cells transfected with control siRNA and untransfected cells (D). Expression of green fluorescent protein (GFP) after transfection was detected by fluorescence microscopy.
Figure 1. Expression of GFP in SKM-1 cells. (A) Untransfected SKM-1 cells. M1 showed untransfected SKM-1 cells. (B) RPS14-shRNA transfected SKM-1 cells. Transfection efficiency was 68.41% (M2). (C) SKM-1 cells transfected with negative control vector. Transfection efficiency was 58.04% (M2). (D) Protein expression of RPS14 in SKM-1 cells transfected with RPS14-shRNA. Lane 1, untransfected SKM-1 cells; lane 2, SKM-1 cells transfected with negative control vector; lane 3, SKM-1 cells transfected with RPS14-shRNA. (E) Untransfected SKM-1 cells under fluorescence microscope. (F) SKM-1 cells transfected with RPS14-shRNA under fluorescence microscope.
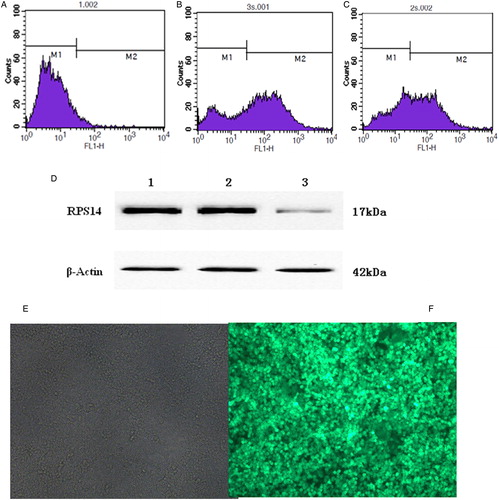
Detection of cell proliferation
To determine whether RPS14 gene silencing by RNAi exerted an inhibitory effect on SKM-1 cells, cell proliferation was detected by MTT assay. In SKM-1 cells transfected with RPS14-shRNA, the proliferation was suppressed significantly when compared with untransfected cells and those transfected with negative control (A). However, after treatment with RPS14 (ATGen, Gyeonggi-do, South Korea) at 10 µg/ml, suppression of proliferation of SKM-1 cells transfected with RPS14-shRNA was released, the cell proliferation remained unchanged in different groups (B). After treatment with Ara-C at 5 mg/ml, the proliferation of SKM-1 cells transfected with RPS14-shRNA was suppressed significantly when compared with untransfected cells and those transfected with negative control (C). Further, when treatment with Ara-C at 5 mg/ml and RPS14 at 10 µg/ml, the proliferation of SKM-1 cells transfected with RPS14-shRNA was suppressed when compared with untransfected cells and those transfected with negative control (D).
Figure 2. Proliferation of SKM-1 cells evaluated by MTT assay. Cell growth curves were delineated and inhibition rate of cell growth was calculated by measuring absorbance at 490 nm. Data represent means of three replicates. (A) The proliferation of SKM-1 cells transfected with RPS14-shRNA was suppressed significantly when compared with untransfected cells and those transfected with negative control. (B) The proliferation of SKM-1 cells in different groups remained unchanged after treatment with RPS14 at 10 µg/ml. (C) The proliferation of SKM-1 cells transfected with RPS14-shRNA was suppressed significantly when compared with untransfected cells and those transfected with negative control after treatment with Ara-C at 5 mg/ml. (D) The proliferation of SKM-1 cells transfected with RPS14-shRNA was less suppressed when compared with untransfected cells and those transfected with negative control after treatment with Ara-C at 5 mg/ml and RPS14 at 10 µg/ml.
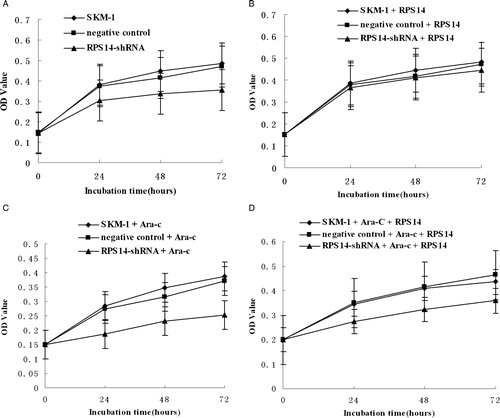
Decreased expression of RPS14 caused p53 activation in SKM-1 cells
Given the evidence that p53 was activated in animal models of ribosomal dysfunction, the effect of decreased expression of RPS14 on p53 expression was investigated in SKM-1 cells. Transfection with RPS14 siRNA caused p53 activation as compared with cells transfected with negative control and untransfected cells. Both semi-quantitative RT-PCR and quantitative real-time PCR (data not shown) demonstrated that RPS14 mRNA expression in SKM-1 cells transfected with RPS14 siRNA decreased significantly as compared with those transfected with negative control and untransfected cells ().
Figure 3. Expression of RPS14 and p53 in SKM-1 cells. (A) Total RNA was isolated from SKM-1 cells (1) and SKM-1 cells transfected with negative control (2), SKM-1 cells transfected with RPS14-shRNA (3). Semi-quantitative PCR was performed to measure the mRNA expressions of RPS14 and p53. RPS14 mRNA expression decreased significantly, but p53 mRNA expression increased significantly in SKM-1 cells after transfection with RPS14-shRNA. (B) Protein expressions of RPS14 and p53 were determined by western blot assay. (1) SKM-1 cells, (2) SKM-1 cells transfected with negative control, (3) SKM-1 cells transfected with RPS14-shRNA. Cell lysates were subjected to electrophoresis and processed for western blot assay with RPS14, p53, and GAPDH antibodies. RPS14-shRNA transfection significantly inhibited RPS14 expression in SKM-1 cells, and markedly increased p53 expression in SKM-1 cells. (C) The analyzed results of densitometry of (A). RPS14 mRNA expression in SKM-1 cells transfected with RPS14-shRNA decreased significantly when compared with untransfected SKM-1 cells (P < 0.05). P53 mRNA expression in SKM-1 cells transfected with RPS14-shRNA decreased significantly when compared with untransfected SKM-1 cells (P < 0.05). (D) The analyzed results of densitometry of (B). RPS14 protein expression in SKM-1 cells transfected with RPS14-shRNA decreased significantly when compared with untransfected SKM-1 cells; and p53 expression increased dramatically when compared with SKM-1 cells (P < 0.05).
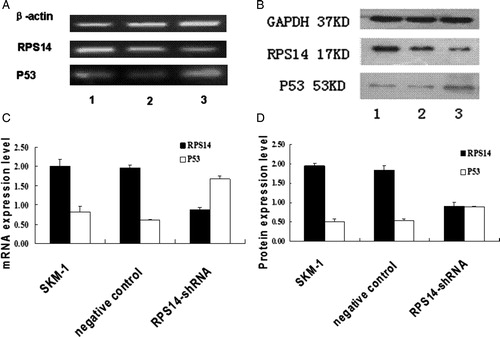
Detection of cell cycle and apoptosis
FACS was employed to assess apoptosis and cell cycle. The apoptosis rate was 17.63 ± 0.874% at 6 days after transfection with RPS14-shRNA (A and C). Significant difference in the apoptosis rate was observed in RPS14-shRNA transfected cells and untransfected SKM-1 cells (P < 0.05). 7-AAD and BrdU were used to evaluate the cell cycle. Results showed the percentage of RPS14-shRNA transfected cells in S phase decreased significantly (B and D), but that in G0/G1 phase increased. The proportion of cells in M phase remained unchanged.
Figure 4. Effects of RPS14-shRNA on apoptosis and cell cycle of SKM-1 cells in flow cytometry. (A) Apoptosis rate was evaluated by FACS after transfection with RPS14-shRNA. (B) Cell cycle was assessed by FACS after transfection with RPS14-shRNA. (C) The analyzed results of apoptosis rate in (A). Apoptosis rate of SKM-1 cells transfected with RPS14-shRNA significantly increased, when compared with SKM-1 cells transfected with negative control (P < 0.05). (D) The analyzed results of cell cycle in (B). The percentage of RPS14-shRNA transfected cells in S phase decreased markedly when compared with cells transfected with negative control (P < 0.05). The percentage of RPS14-shRNA transfected cells in G0/G1 phase increased as compared with those transfected with negative control (P < 0.05). RPS14-shRNA transfection results in cell cycle arrest.
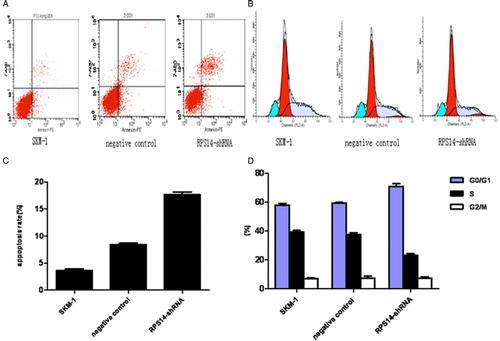
Discussion
RPS14, a ribosomal gene encoding for a component of ribosomal subunit 40S, is required for the processing of 18S pre-rRNA. Haploinsufficiency of RPS14 is closely related to the 5q- syndrome and may cause p53 activation.Citation13 Although there is a possibility that other genes in CDR may be also involved in this process,Citation14 it has been widely accepted that RPS14 may serve as a causal gene in the 5q- syndrome.Citation3,Citation13,Citation15 Knockdown of RPS14 was proved to result in p53 activation in HCT116 cells and A549 cells.Citation16 Zhou et al.Citation16 found that RPS14 could bind to the central acidic domain of MDM2 and inhibit its E3 ubiquitin ligase activity towards p53. However, whether RPS14 plays a direct role in carcinogenesis of MDS transforming into AML remains still unclear. Although studies showed that haploinsufficiency of RPS14 indeed caused p53 activation in the 5q- syndrome,Citation2,Citation17 there was no report regarding the role of RPS14 and the relationship between p53 and RPS14 in MDS/AML. To clarify the role of RPS14 in MDS/AML, shRPS14 was constructed to silence RPS14 expression in MDS/AML cells without 5q deletion. Results showed that RPS14 silencing by RNAi exerted an inhibitory effect on the proliferation of SKM-1 cells. Moreover, RPS14 was highly associated with negative regulation of p53 in MDS/AML cells. RPS14-shRNA transfection caused the p53 activation in SKM-1 cells, arrested these cells in G0/G1 phase, and induced apoptosis, suggesting that RPS14 is involved in the pathophysiology of MDS/AML.
P53 gene as a tumor suppressor gene is closely related to the proliferation, apoptosis, and metastasis of cancer cells.Citation9,Citation18 Knockdown of ribosomal gene can cause ribosomal stress leading to p53 activation through blocking MDM2-p53 feedback loop, which then promotes the transcription of its target genes resulting in p53-independet cell cycle arrest or apoptosis.Citation15,Citation19 Investigators also indicate that activation of p53 and up-regulation of the p53 pathway as a result of ribosomal stress seem to play an important role in the 5q- syndrome.Citation3,Citation8 RPS14 is down-regulated in MDS/AML cells, and associated with the negative regulation of p53. Thus, we hypothesize that RPS14 may also affect cell cycle and p53 activation resulting in occurrence of MDS. To test this hypothesis, the cell cycle and apoptosis of SKM-1 cells were determined. The proliferation of SKM-1 cells transfected with RPS14-shRNA was significantly suppressed by 30% when compared with untransfected cells and those transfected with negative control, suggesting that the cell viability is reduced due to apoptosis. This was consistent with the apoptotic phenotype of MDS in a previous study.Citation20 Moreover, RPS14 silencing by shRNA increased the apoptosis rate by five times and induced cell cycle arrest at G0/G1 phase. The increased proportion of cells in G0/G1 phase might be attributed to the dysfunction of p21, the activation of which is p53 dependent.Citation21 Since p21 exerts negative regulation on cells in G1 phase, lack of p53 in SKM-1 cells induces p21 activation leading to arrest in G1/G0 phase. Studies also revealed that p53 and p21 are necessary to maintain the arrest in G2 phase, which is not dependent on their interaction.Citation22,Citation23 Thus, the proportion of cells in M phase remained unchanged. These results confirm that knockdown of RPS14 causes ribosomal stress, and the p53 pathway is involved in the pathogenesis of MDS as shown in other studies.Citation11
In conclusion, our results suggest that partial silencing of RPS14 inhibits the proliferation of MDS/AML cells, and RPS14 negatively regulates p53 activation in MDS/AML cells. RPS14 may serve as a new therapeutic target for the treatment of MDS.
Authorship and disclosures
L.W. was the principal investigator and took primary responsibility for this paper; L.W. and L.L. wrote this paper; J.L. and Q.N. performed experiment in this study; Q.X. andZ.S.Y. took part in data processing and writing paper. This project was supported by the National Nature Science Foundation of China (Grant no: H0807-30971277; H0807-81250034) and Chongqing Natural Science Foundation (Grant no: CSTC 2009BB5070).
References
- Boultwood J, Pellagatti A, Wainscoat JS. 5q- syndrome. Curr Pharm Des. 2012;18(22):3180–3.
- Ebert BL, Pretz J, Bosco J, Chang CY, Tamayo P, Galili N, et al. Identification of RPS14 as a 5q- syndrome gene by RNA interference screen. Nature 2008;451(7176):335–9.
- Barlow JL, Drynan LF, Hewett DR, Holmes LR, Lorenzo-Abalde S, Lane AL, et al. A p53-dependent mechanism underlies macrocytic anemia in a mouse model of human 5q- syndrome. Nat Med. 2010;16(1):59–66.
- Czibere A, Bruns I, Junge B, Singh R, Kobbe G, Haas R, et al. Low RPS14 expression is common in myelodysplastic syndromes without 5q- aberration and defines a subgroup of patients with prolonged survival. Haematologica. 2009;94(10):1453–5.
- Lane DP. Exploiting the p53 pathway for the diagnosis and therapy of human cancer. Cold Spring Harb Symp Quant Biol. 2005;70:489–97.
- Oren M. Regulation of the p53 tumor suppressor protein. J Biol Chem. 1999;274(51):36031–34.
- Jin S, Levine AJ. The p53 functional circuit. J Cell Sci. 2001;114(Pt 23):4139–40.
- Dutt S, Narla A, Lin K, Mullally A, Abayasekara N, Megerdichian C, et al. Haploinsufficiency for ribosomal protein genes causes selective activation of p53 in human erythroid progenitor cells. Blood. 2011;117(9):2567–76.
- Vogelstein B, Lane D, Levine AJ. Surfing the p53 network. Nature. 2000;408(6810):307–10.
- Hofseth LJ, Hussain SP, Harris CC. p53: 25 years after its discovery. Trends Pharmacol Sci. 2004;25(4):177–81.
- Narla A, Ebert BL. Ribosomopathies: human disorders of ribosome dysfunction. Blood. 2010;115(16):3196–205.
- Scherr M, Eder M. Gene transfer into hematopoietic stem cells using lentiviral vectors. Curr Gene Ther. 2002;2:45–55.
- Pellagatti A, Hellstrom-Lindberg E, Giagounidis A, Perry J, Malcovati L, Della Porta MG, et al. Haploinsufficiency of RPS14 in 5q- syndrome is associated with deregulation of ribosomal- and translation-related genes. Br J Haematol. 2008;142(1):57–64.
- Wang L, Fidler C, Nadig N, Giagounidis A, Della Porta MG, Malcovati L, et al. Genome-wide analysis of copy number changes and loss of heterozygosity in myelodysplastic syndrome with del(5q) using high-density single nucleotide polymorphism arrays. Haematologica. 2008;93(7):994–1000.
- Boultwood J. The role of haploinsufficiency of RPS14 and p53 activation in the molecular pathogenesis of the 5q- syndrome. Pediatr Rep. 2011;3 (Suppl. 2):e10.
- Zhou X, Hao Q, Liao J, Zhang Q, Lu H. Ribosomal protein S14 unties the MDM2-p53 loop upon ribosomal stress. Oncogene 2013;32(3):388–96.
- Oliva EN, Cuzzola M, Nobile F, Ronco F, D'Errigo MG, Lagana C, et al. Changes in RPS14 expression levels during lenalidomide treatment in low- and intermediate-1-risk myelodysplastic syndromes with chromosome 5q deletion. Eur J Haematol. 2010;85(3):231–5.
- Sharpless NE, DePinho RA. p53: good cop/bad cop. Cell. 2002;110(1):9–12.
- Wu Q, Gou Y, Wang Q, Jin H, Cui L, Zhang Y, et al. Downregulation of RPL6 by siRNA inhibits proliferation and cell cycle progression of human gastric cancer cell lines. PLoS ONE. 2011;6(10):e26401.
- Raza A, Gezer S, Mundle S, Gao XZ, Alvi S, Borok R, et al. Apoptosis in bone marrow biopsy samples involving stromal and hematopoietic cells in 50 patients with myelodysplastic syndromes. Blood. 1995;86(1):268–76.
- Pietenpol JA, Stewart ZA. Cell cycle checkpoint signaling: cell cycle arrest versus apoptosis. Toxicology. 2002;181–182:475–81.
- Bunz F, Dutriaux A, Lengauer C, Waldman T, Zhou S, Brown JP, et al. Requirement for p53 and p21 to sustain G2 arrest after DNA damage. Science. 1998;282(5393):1497–501.
- Flatt PM, Tang LJ, Scatena CD, Szak ST, Pietenpol JA. p53 regulation of G(2) checkpoint is retinoblastoma protein dependent. Mol Cell Biol. 2000;20(12):4210–23.