Abstract
Objective:
Tumor necrosis factor (TNF) is a highly pleiotropic cytokine with multiple activities other than its originally discovered role of tumor necrosis in rodents. TNF is now understood to play a contextual role in driving either tumor elimination or promotion. Using both animal and human data, this review examines the role of TNF in cancer development and the effect of TNF and TNF inhibitors (TNFis) on malignancy risk.
Research design:
A literature review was performed using relevant search terms for TNF and malignancy.
Results:
Although administration of TNF can cause tumor regression in specific rodent tumor models, human expression polymorphisms suggest that TNF can be a tumor-promoting cytokine, whereas blocking the TNF pathway in a variety of tumor models inhibits tumor growth. In addition to direct effects of TNF on tumors, TNF can variously affect immunity and the tumor microenvironment. Whereas TNF can promote immune surveillance designed to eliminate tumors, it can also drive chronic inflammation, autoimmunity, angiogenesis, and other processes that promote tumor initiation, growth, and spread. Key players in TNF signaling that shape this response include NF-κB and JNK, and malignant-inflammatory cell interactions, each of which may have different responses to TNF signaling. Focusing on rheumatoid arthritis (RA) patients, where clinical experience is most extensive, a review of the clinical literature shows no increased risk of overall malignancy or solid tumors such as breast and lung cancers with exposure to TNFis. Lymphoma rates are not increased with use of TNFis. Conflicting data exist regarding the risks of melanoma and nonmelanoma skin cancer. Data regarding the risk of recurrent malignancy are limited.
Conclusions:
Overall, the available data indicate that elevated TNF is a risk factor for cancer, whereas its inhibition in RA patients is not generally associated with an increased cancer risk. In particular, TNF inhibition is not associated with cancers linked to immune suppression. A better understanding of the tumor microenvironment, molecular events underlying specific tumors, and epidemiologic studies of malignancies within specific disease indications should enable more focused pharmacovigilance studies and a better understanding of the potential risks of TNFis.
Introduction
Various lines of evidence demonstrate a relationship between the immune system and cancer risk in humans and animal modelsCitation1,Citation2. In humans, an increased risk of various cancers is observed in patients with chronic inflammatory conditionsCitation3–5, primary (congenital) immune deficienciesCitation6,Citation7, acquired deficiencies (e.g., HIV/AIDS)Citation8–10, or with therapeutics used to suppress inflammation in patients with various inflammatory disorders or to support organ engraftmentCitation11–14. Additionally, emerging data demonstrate the potential for immunotherapy in the treatment of specific cancersCitation15–17 and the strong prognostic value of characterizing the immune composition in the tumor microenvironment in predicting patient survivalCitation18,Citation19.
Collectively, several juxtaposing models have been proposed to describe the relationship between specific arms of immunity and cancerCitation1. In the tumor immunosurveillance/immunoediting model, elements of the innate and adaptive immune system protect the host by eliminating transformed cells, with a mutual evolution of the immune response and the cancer as disease progressesCitation20,Citation21. Host immunity can also protect the host by eliminating, or at least suppressing, infection with oncogenic virusesCitation22,Citation23. However, chronic inflammation can contribute to cancer initiation and tumor progression by creating a permissive microenvironment for tumor invasion and metastasisCitation24–27. Finally, chronic antigenic stimulation, as seen with autoimmunity or unresolved pathogenic infection, can drive lymphomagenesisCitation1. Contextual relationships between specific immune alterations and specific cancers challenge the development of generalizations regarding cancer risk with immune alterations. This challenge is becoming more acute as therapeutic strategies evolve from broad-spectrum immune suppression, used in the treatment of grievous conditions such as organ transplantation, to limited immunomodulation targeting specific cytokines or immune subsets.
Tumor necrosis factor (TNF, cachectin, TNF-α) is a central proinflammatory cytokine involved in various inflammatory conditions, including autoimmunity; inhibitors of TNF (TNFis) can markedly reduce the inflammation and manifestations of disease in patients with these conditionsCitation28. TNF is a trimeric protein, expressed from a wide variety of cells, and exists in both soluble and membrane-associated forms. TNF was originally identified as a glycoprotein found in the serum of Bacillus Calmette-Guérin–infected infected mice given endotoxin (also known as lipopolysaccharide). When this serum was administered intravenously, necrosis of sarcomas that had been transplanted subcutaneously into mice was observedCitation29,Citation30. Cloning of human TNF was reported 10 years later by several groupsCitation31,Citation32. TNF is now known to have potent cytotoxic and proinflammatory effects. The observations that TNF could induce inflammatory responses and was elevated at sites of inflammation led researchers to explore the potential therapeutic benefit of inhibiting TNF activity in vivoCitation33,Citation34. A secreted cytotoxic protein expressed by lymphocytes was also being characterized, purified, and subsequently clonedCitation35–37. This factor, called lymphotoxin (LT), also demonstrated tumor necrosis activity and had noticeable sequence identity to TNFCitation38,Citation39. Both LT and TNF have been shown to bind and activate TNFR1 and TNFR2, inducing similar in vitro responses such as cytotoxicity in L929 cellsCitation38.
Both lymphotoxin (LT or LT-α, TNF-β) and TNF bind to the same two receptors: TNF receptor (TNFR) superfamily 1a (TNFRSF1a; p55 or p60, type I, TNFR1, CD120a, ubiquitous expression) and TNFRSF1b (p75 or p80, type II, TNFR2, CD120b, expression is restricted to immune cells)Citation28,Citation40, inducing similar in vitro responses such as cytotoxicity in L929 cellsCitation38. Soluble TNFRs are shed forms of the extracellular portion of the receptorCitation40,Citation41 that bind TNF and inhibit its activity, thus regulating the bioavailability of soluble TNFCitation40.
There are five TNFis that are currently licensed for use in the United States: three (adalimumab, golimumab, and infliximab) are monoclonal antibodiesCitation42–44, one (certolizumab) is a PEGylated Fab' fragmentCitation45, and one (etanercept) is a soluble receptor Fc fusion proteinCitation46. In addition to blocking TNF, only etanercept also inhibits LT; the properties of these agents are outlined in . Since the approval of the first marketed TNFi in 1998, globally there have been over 4 million patient-years of exposure to etanercept aloneCitation47. The broad clinical administration of these therapies, which are used chronically in a variety of inflammatory conditions (), combined with the identified association of systemic immune suppression (i.e., with congenital immunodeficiencies or acquired/induced profound immunosuppression) and increased cancer risk, has promoted interest in the potential long-term risks to patients treated with TNFis.
Table 1. Summary of currently approved TNFi therapies in the United States.
In this review, we analyze the current understanding of cancer risk with TNFis based on (a) information relating to TNF and tumorigenesis in experimental models, (b) understanding of the relationship between TNF, immunity, and cancer (TNF and tumor immune surveillance, TNF and antiviral immunity, TNF promoter polymorphisms and cancer risk), and (c) the accumulated clinical experience of TNFis over the last 16 years, notably in the treatment of rheumatoid arthritis (RA). A topic search was completed in MEDLINE and MEDLINE In-Process & Other Non-Indexed Citations on the Ovid database system. The search strategy consisted of using MeSH descriptors for tumor necrosis factor-alpha, lymphotoxin-alpha, cancer, cancer proliferation, malignancy, and immunosurveillance. Relevant clinical literature was searched through Ovid using the following search string: ((etanercept or Enbrel or Remicade or infliximab or adalimumab or Humira or golimumab or Simponi or certolizumab or Cimzia) AND (malignancy or malignancies or neoplasm malignant or neoplasm cancer or malignant tumor or malignant neoplasm or cancer or tumor malignant or neoplasm or unclassified tumor) and recurrence)).
Tumor necrosis factor and tumorigenesis in experimental studies
Antitumor activities of tumor necrosis factor
As mentioned above, TNF was originally identified as a glycoprotein found in the serum of Bacillus Calmette-Guérin–infected mice given endotoxin (also known as lipopolysaccharide). This serum could induce necrosis of sarcomas that had been transplanted subcutaneously into mice when administered intravenouslyCitation29,Citation30. The structurally related protein LT has also demonstrated tumor necrosis activityCitation39.
Experiments using purified TNF or cell lines expressing TNF demonstrate that in the models of established tumors, TNF can induce tumor necrosis. High concentrations of TNF are capable of killing tumor cells in in vitro systems and in animal modelsCitation48–50. Based on this potential for TNF to induce tumor cell death, the utilization of TNF as a cancer therapy has been explored in the clinical setting. Local administration by isolated limb perfusion of TNF in combination with the alkylating agent melphalan or with doxorubicin was effective treatment for patients with metastatic melanoma and unresectable soft-tissue sarcomasCitation51–54. Similar results have been observed for liver metastases of colorectal tumors in which isolated hepatic perfusion of TNF and melphalan was introduced via laparotomyCitation55. The predominant effects of TNF delivered directly into tumors by isolated perfusion involve the tumor vasculatureCitation51,Citation56. Specifically, the procoagulant effects of TNFlCitation50 may cause tumor necrosis through thrombus formation within the vasculatureCitation57. Overall, the available experimental data indicate that TNF is at most weakly cytotoxic or cytostatic to malignant cells, and the presence of metabolic inhibitors, which suppress downstream TNF signaling factors, is required for TNF-induced apoptosis to proceedCitation58.
TNFR1 and TNFR2 are connected to multiple intracellular signaling pathways. TNFR1 can induce expression of genes involved in inflammation, survival, and proliferation through activation of kinase signaling cascades that ultimately activate nuclear factor kappa B (NF-κB)Citation59. Alternatively, through the formation of the death-inducing signaling complex, TNFR1 can trigger cell death through apoptosis or necroptosis (programmed necrotic cell death; )Citation59. On balance, TNF is a poor inducer of apoptosis unless accompanied by inhibition of NF-κB, RNA synthesis, or protein synthesisCitation60–62. TNFR2, expressed on a more restricted subset of cells, activates pathways implicated in cell survival proliferation and migration and modulates regulatory T-cell functionCitation63. Multimerization of transmembrane TNF (caused by association of surface-expressed receptors or through binding to bivalent antibodies) may also induce intracellular signaling of TNF-expressing cells (i.e., reverse signaling), leading to apoptosis, cell activation, or cytokine suppressionCitation28.
Figure 1. TNFRSF1a signal transduction. Activation of TNFRSF1a results in NF-κB activation and ultimately inflammation, angiogenesis, and cell survival. The apoptotic pathway is suppressed by FLIP. A decrease in NF-κB activation results in reduction of FLIP, which then favors signaling through the dissociated intracellular DD complex, resulting in caspase-dependent apoptosis. DD = death domain; FADD = Fas-associated death domain; FLIP = FADD-like IL-1β–converting enzyme; JNK = Jun kinase; NF-κB = nuclear factor kappa B; TNF = tumor necrosis factor; TNFRSF1a = TNF receptor superfamily 1a; TRADD = TNF receptor–associated death domain; TRAF = TNF receptor–associated factor. Reprinted (with modification) from: Micheau O, Tschopp J. Induction of TNF receptor I-mediated apoptosis via two sequential signaling complexes. Cell 2003;114:188 © 2003 with permission from Elsevier.
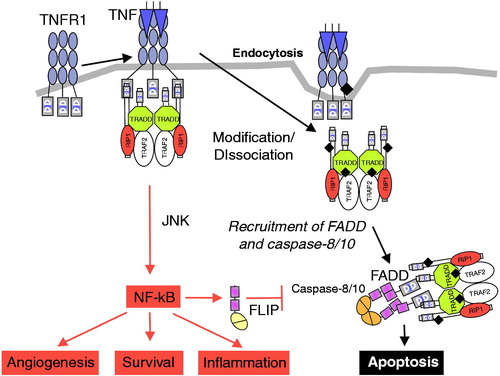
Although originally identified as a serum factor associated with hemorrhagic necrosis of tumors induced by endotoxinCitation29, TNF is now understood to play a contextual role in driving either tumor elimination or promotionCitation26,Citation58,Citation64–70.
Impact of blocking tumor necrosis factor on tumorigenesis
The impact of blocking TNF on tumorigenesis has been examined using a number of models corresponding to different stages of tumor growth and different types of tumors, as summarized in .
Table 2. Role of TNF in tumorigenesis in nonclinical tumor models.
While systemic or intratumoral injection of supraphysiological levels of TNF can cause inhibition of tumor growth, studies examining animal models of tumorigenesis demonstrate that eliminating TNF or its receptors through genetic manipulation (TNF−/− or TNFR−/− mice) is markedly inhibitory to tumor induction and growth.
Suganuma et al.Citation71 and Moore et al.Citation72 have reported that TNF knockout (TNF−/−) mice were more resistant to tumor formation than TNF+/+ mice when evaluated in skin carcinogenesis models with chemical induction/promotion. TNF−/− mice are protected from urethane−induced tumorigenesisCitation73, and blocking TNF bioactivity with the p75 TNF receptor Fc fusion protein etanercept blocked tumor initiation/promotion and tumor progression in urethane-dosed mice through reduction of tumor microvascular density and cell proliferationCitation75. The tumor-promoting activity of TNF was also demonstrated in a benzo[a]pyrene-7,8-diol-9,10-epoxide skin carcinogenesis modelCitation81. Endogenous TNF enhanced the malignancy of the lung carcinoma cell line 3LL cells introduced into TNF+/+ versus TNF−/− mice as the result of the induction of the promalignant gene secretory leukocyte protease inhibitor SLP1Citation77–79,Citation82,Citation83. Studies with TNFR-deficient mice show similar results. TNFR1/2−/− mice were found to have significantly fewer tumors in response to ultraviolet B irradiationCitation73. TNFR1−/− mice were also resistant to liver tumor formation in a model in which oval cell proliferation is induced by a choline-deficient, methionine-supplemented dietCitation76.
TNF was identified as a critical macrophage-derived factor for promoting an epithelial-mesenchymal transition in a model of invasive colon cancerCitation82. Inhibition of TNF by etanercept or the antibody infliximab exerted strong antitumoral effects in a pancreatic ductal adenocarcinoma (PDAC) model in severe combined immunodeficiency (SCID) mice, illustrating the important role of tumor-derived TNF in tumor growthCitation77. Administration of etanercept to wild-type (WT) mice after treatment with azoxymethane and dextran sulfate sodium (a model of ulcerative colitis leading to the development of colonic tumors) markedly reduced the number and size of tumors and reduced colonic infiltration by neutrophils and macrophagesCitation78. TNF also facilitates the clonal expansion and dominance of mutated Janus kinase 2 cells in myeloproliferative neoplasmsCitation83.
Taken together, these data indicate that in various models TNF can stimulate or promote tumor formation, whereas the lack or inhibition of TNF can reduce tumor formation.
Tumor necrosis factor and metastatic processes in experimental models
Several lines of evidence demonstrate that TNF may promote metastasis of certain tumor cell types. As mentioned earlier, TNF treatment dramatically enhanced PDAC growth and metastasis in a mouse model, whereas TNFi treatment with infliximab or etanercept showed strong antitumoral effects, decreasing liver metastases and volumes of recurrent tumorsCitation77. Similarly, in a separate in vitro study, TNF treatment of cultured colon-26 cells enhanced metastatic properties involving production of metallomatrix protein-9, adhesion, migration, and invasionCitation84–87. Chinese hamster ovary cells overexpressing TNF had a greater ability to invade and metastasize in mice compared with cells not overexpressing TNFCitation86, and metastatic lymphoma cells have been shown to have an even greater metastatic potential when coadministered with recombinant mouse TNFCitation87. Inhibition of TNF using an anti-TNF fusion protein (human TNFR1 extracellular portion and human immunoglobulin G1 [IgG1] Fc) decreased metastasis to the lung in a B16-BL6 mouse melanoma model, demonstrating a direct relationship between TNF and the metastatic processCitation80,Citation88. Myoblasts restrict prostate cancer growth and limit metastasis formation through paracrine TNF secretionCitation80.
Taken together, the results of these studies indicate that TNF can promote metastasis and that TNFi treatment in rodent models decreases metastatic potential.
Different mechanisms connecting tumor necrosis factor, immunity, and cancer
Tumor necrosis factor and tumor immune surveillance
In the immunosurveillance model, the host immune system protects against tumor growth and developmentCitation21. Innate and adaptive immune cells, primarily natural killer (NK), cytotoxic T-lymphocyte (CTL), and natural killer T (NKT) cells, are the key mediators of host protection from cancer, working in concert with key cytokines and mediators (e.g., interferon [IFN]-γ, perforin, granzymes) to eliminate tumors. However, tumors can downregulate antigen presentation to evade the immune system or express cytokines (e.g., tumor growth factor [TGF]-β, interleukin [IL]-10) to induce immunosuppressive cells (e.g., myeloid-derived suppressor cells, tumor-associated macrophages, and regulatory T cells). This model is supported by mechanistic data evaluating tumor growth in various animal models with immunodeficiency (e.g., recombination activation gene [RAG]1−/−, RAG2−/−, signal transducer and activator of transcription [STAT]1−/−, SCID, nudeCitation21). In addition, inflammatory cell infiltration within primary tumor samples from patients with colorectal, breast, and other cancer types shows that the presence of CD8+ and Th1 T cells is associated with good prognosis, and while the presence of Th17 and regulatory T cells in predicting patient outcome can vary between tumor types, generally poor outcomes have been reportedCitation18,Citation19. Evidence supporting the importance of a strong adaptive antitumor response is emerging from clinical data on approved immunotherapies, which augment antitumor immune responses, particularly dendritic cell (DC) and CD8+ T-cell interactionsCitation15,Citation16. In addition, a variety of tumor-derived factors contribute to the emergence of immunosuppressive networks, including vascular endothelial growth factor, IL-10, TGF-β, prostaglandin E(2), soluble phosphatidylserine, soluble Fas, soluble Fas ligand, and soluble major histocompatibility complex (MHC) class I–related chain A proteins. These factors can suppress protective host immune responses and promote tumor growth, invasion, and metastasisCitation89,Citation90.
The specific role of TNF in regulating factors of immune surveillance of tumors has been studied in a variety of models (). Studies of TNF−/−, LT−/−, TNFR−/− mice provide information on the key roles of these genes in the immune system and immune surveillance, which help identify their potential roles in tumorigenesis. In several rodent tumor models, TNF was associated with a protective immunosurveillance. Tumor rejection in TNF−/− mice was reported to be inhibited with the highly antigenic fibrosarcoma MC57XCitation91. In this model, tumor rejection is dependent on animals mounting an immune response to tumor antigens (i.e., mice are immunized with irradiated tumor cells before implantation), and thus failure to reject may be the result of the blunted immune response in TNF−/− mice. The administration of a TNF antibody to WT mice also resulted in impaired tumor rejection in this model, and the administration of recombinant TNF (rTNF) to TNF−/− mice resulted in tumor rejectionCitation91. In addition, in a modified model of metastatic lung cancer using the 3LLA-9 Lewis lung carcinoma cell line, in which cells transfected with a lymphocytic choriomeningitis virus antigen can be rejected because of an immune response to the transfected antigen, tumor rejection failed to occur in TNF−/− miceCitation100. Using the methylcholanthrene (MCA)–induced sarcoma as a chemically induced cancer model sensitive to effects on innate and adaptive immune surveillance, TNF−/− mice showed increased susceptibility compared with TNF+/+ miceCitation101. However, TNFR1−/−, TNFR2−/−, and TNFR1/2−/− animals did not show increased development of MCA-induced sarcoma compared with WT littermatesCitation102. Tumor-draining lymph node effector T cells cultured with TNF mediate increased regression of established mouse tumorsCitation103. In a model of LT deficiency, impaired NK function was associated with increased tumor growth and metastasisCitation104.
Table 3. Impact of TNF on immune surveillance (nonclinical models).
TNF−/− and/or LT−/− mice have specific immune function changes, including impaired NK and lymphokine-activated killer (LAK) cytotoxicity against various tumor cell lines (L929, WEHI 164, P815 for LAK; YAC-1 for NK)Citation91,Citation93–95,Citation105, supporting a role for TNF in tumor killing. Similarly, CTL responses against DCs pulsed with cellular extracts from allogeneic splenocytes were also decreased compared with TNF+/+ littermates, while it was shown that TNF−/− effector lymphocytes still exhibit both perforin and Fas ligand-based cytotoxicityCitation91. Similar to LAK and NK activities, CTL cytotoxicity was restored upon incubation of TNF−/− lymphocytes in vitro with rTNF during a cytotoxicity assay or upon treatment of TNF−/− mice with rTNFCitation91.
NK cell development from bone marrow precursors is suppressed in LT−/− mice, and splenocytes from LT−/− mice exhibit lower NK cytotoxicity in vitro against YAC-1 cells, possibly as the result of a lower content of splenic NK cells, while perforin is detectable in NK cells from LT−/− miceCitation104. In vitro cytotoxicity function against target cells P815 (MHC class I+, class II−) and TA3 (MHC class I+, class II+) is intact in LT−/− miceCitation96.
Characterization of the role of TNFR in immunosurveillance, using TNFR1−/−, TNFR2−/−, or TNFR1/2−/− mice, has been limited and more complex to interpret; TNFR2−/− CD8+ T cells are resistant to apoptosis, leading to increased protection against tumor growthCitation97,Citation106.
Overall, the complete abrogation of TNF signaling may impair some components of immunosurveillance in rodents, although in some instances the disruption of TNF signaling may increase antitumor CTL activity (due to decreased apoptosis).
In different experimental settings, TNF was associated with a negative effect on immunosurveillance. TNF expression was significantly associated with positive lymph node stage and recurrence of colorectal cancer, possibly by induction of apoptosis of tumor-infiltrating CD8+ T cellsCitation107. TNF was demonstrated to favor proliferation of myelodysplastic syndrome blasts through induction of the immunoinhibitory molecule B7-H1, responsible for T-cell suppression via programmed cell death (PD-1) moleculesCitation98. In a murine model of melanoma, treatment with DCs matured by TNF led to an increase in the number of lung metastases, an effect associated with increased lung IL-10+ T cells and not observed in IL-10−/− miceCitation108. LT treatment was shown to decrease MHC I expression in HPV16-positive CaSki cells and to reduce CTL-specific lysis of CaSki cellsCitation109.
These seemingly contradictory results, which demonstrate both positive and negative roles of TNF in immunosurveillance, suggest that the role of TNF in immune surveillance is complex, contextual and reflects the pleiotropic nature of TNF signaling.
Specific effects of TNFis on some components of immune surveillance have been evaluated through animal studies. Cynomolgus monkeys administered 3 mg/kg etanercept twice weekly showed no changes in total lymphocyte/lymphocyte-subtype populations, nor were there any changes in markers for ‘activation’ or ‘memory’ phenotypes when using the following markers: CD3 (T lymphocytes), CD3/CD4 (helper T cells), CD3/CD8 (cytotoxic T cells), CD4/CD44 (memory CD4 cells), CD8/CD44 (memory CD8 cells), CD4/CD69 (predominantly activated helper T cells), CD8/CD69 (activated cytotoxic T cells), CD14 (monocytes), CD16 (NK cells), CD16/CD69 (activated NK cells), and CD20 (B lymphocytes)Citation47. To assess the possible influence of etanercept on immune function, animals were immunized with an antigenic preparation for delayed-type hypersensitivity (DTH) response assessment, and with keyhole-limpet hemocyanin and tetanus toxoid to assess capacity for production and maintenance of a primary or secondary T cell–dependent antibody responseCitation47. The administration of etanercept had no apparent effect on the ability of cynomolgus monkeys to mount a DTH response to an antigenic mixture of candida/trichophyton/diphtheria, an antibody response to a novel antigen, or to a recall antigen.
Studies in mice have shown that etanercept can modulate the immune response to specific challenges. For example, in murine models of collagen-induced arthritis, suppression of the immune-based destruction of joint tissue is the basis for therapeutic activity. However, in these models, normal levels of T-cell responses to mitogens and type II collagen are foundCitation110. Together, these data show that pharmacologic inhibition of TNF is not associated with broad immunosuppression.
Tumor necrosis factor and antiviral immunity
The host response to viral infection is mediated by both innate (NK, NKT, and granulocyte) and adaptive (CTL) antiviral responses, which contribute to viral clearance. The production of proinflammatory cytokines such as TNF, IL-12, IL-18, and IL-6 and the cellular production of IFN-α both directly interfere with viral replication and promote antiviral immunityCitation22,Citation23.
Administration of etanercept in an acute model of murine cytomegalovirus infection resulted in increased viral load in the liver in only the very early phase of infection (day 3), and the effects did not extend to later times; no infected cells were seen at day 7 in either treated or control groupsCitation47. However, the effects on a latent infection have not been examined, and at present, there are no established rodent viral models for potential human oncogenic viruses in which the human protein etanercept was examined.
In a clinical study, patients who received infliximab, adalimumab, or etanercept showed no difference in Epstein–Barr virus (EBV) viral load and no difference in numbers of EBV-specific IFN-γ–producing T cells (for both latent-cycle and lytic-cycle peptides) between baseline and treatment week 12Citation111. Other clinical studies showed that TNFi therapy did not increase the EBV burden in patients with RA or ankylosing spondylitisCitation112,Citation113.
Tumor necrosis factor promoter polymorphisms and cancer risk
Inflammation contributes to tumor development and growth through the expression of cytokines such as TNF and other inflammatory mediators, which establish a permissive environment for tumor growth and metastases, angiogenesis, increased cell survival, reduced cell death, altered differentiation, and altered response to hormones and chemotherapeutic agentsCitation24,Citation26,Citation27,Citation58,Citation114–117. Clinical data indicate that inflammation associated with increased TNF is a negative prognostic indicator of patient outcomes across a variety of tumor types. The TNF-308 A>G promoter region polymorphism, associated with two- to three-fold increased transcription and up to two-fold increased circulating TNF levels (for G/A vs G/G) in healthy humansCitation118,Citation119, has been positively associated with various malignancies. Meta-analyses covering eight cancer classifications (cervical, breast, lung, hepatocellular, gastric, aerodigestive, non-Hodgkin lymphoma, and colorectal) and involving over 50,000 cases and 66,000 controls have established a statistically significant increased cancer risk (expressed as an odds ratio) for A allele polymorphisms ranging from 1.1 to >4, with some variability across allelic forms (i.e., A/A, G/A, or either) and subgroup factors (e.g., menopausal status, race)Citation120–129. These data indicate a broad and complex role for TNF-308 G/A polymorphisms that regulate TNF transcription and production, although the relationship of these polymorphisms with genetic and other factors, such as ethnicity, that affect cancer risk remain poorly understoodCitation130.
Other TNF polymorphisms have been linked with an increased cancer risk (i.e., 238 G/A, 857 C/T, 863 A/C, 1031 C/T, 1210 C/T), although meta-analyses have not identified clear associations of these polymorphisms with cancer riskCitation120,Citation123,Citation124,Citation127,Citation131,Citation132. In addition to TNF polymorphisms, the LT-α NcoI A/A genotype, and its associated high production of LT-α, are associated with an increased risk of developing esophageal adenocarcinomaCitation133.
Whereas TNF polymorphisms have been associated with increased TNF production and cancer risk, increased systemic TNF from other causes also increases cancer risk. In B-cell chronic lymphocytic leukemia and hairy cell leukemia, overproduction of TNF by the malignant cells has been reported to result in more severe disease progression and decreased survivalCitation134,Citation135. Freedman et al. found that cells from patients with juvenile chronic myelogenous leukemia endogenously produced TNF, which played an important role in the pathogenesis of the disease by acting as an autocrine growth factorCitation136. These effects were inhibited following treatment with an anti-TNF antibody. TNF also maintains TNFR1-dependent IL-17 production in CD4+ T cells, which leads to myeloid cell recruitment into the tumor microenvironment and enhances tumor growth in ovarian cancer in mice and humansCitation137. Although Helicobacter pylori is considered to be a major factor contributing to gastric cancer, it was demonstrated that TNF mRNA and protein expressions were significantly increased in chronic gastritis, intestinal metaplasia, dysplasia, and gastric adenocarcinoma patients and that the degree of overexpression of TNF showed significant association with various stages of gastric carcinogenesisCitation138. In the context of H pylori, the TNF-inducing protein (Tip-α) was identified as a carcinogenic factor that acts through strong induction of TNFCitation139.
Overall, these data indicate that TNF in the inflammatory microenvironment may favor tumor growth in several models, and TNF expression is a poor prognostic factor for several tumor types in humans.
Taken as a whole, the studies summarized above indicate that TNF can activate pathways leading to three contextually different cellular responses: cell survival and activation/proliferation, transcription of proinflammatory genes, and cell death. These various effects are critical for controlling effectors of immune surveillance of tumors, tumor growth, and nonvirally-mediated lymphomagenesis as summarized below and illustrated in .
Although some models indicate that abrogation of the TNF pathway can reduce NK- and CTL-mediated cytotoxicity against tumor cells, other models indicate that CTL activity is intact when the TNF pathway is inhibited and, furthermore, that TNF can inhibit antitumor immunity through expansion of suppressor cells and induction of T-cell inhibitory molecules. Pharmacologic modulation of the TNF pathway (e.g., with etanercept) does not affect the number of circulating leukocytes (including NK cells and CTLs) in nonhuman primates and does not interfere with T-cell responses and antiviral immunity.
TNF shows antitumor activity in vivo in vascularized tumors through disruption of endothelial cell adhesiveness and procoagulation effects. It is weakly cytotoxic against many tumor cell lines unless combined with antimetabolic agents.
TNF (and inflammation in general) favors tumor growth and metastasis in several models, and available clinical data indicate that inflammation associated with TNF is a negative prognostic indicator of patient outcomes across a variety of tumor types. In particular, the TNF-308 G/A promoter region polymorphism, associated with a two- to three-fold increased transcription and up to two-fold increased circulating TNF levels in healthy humans, has been positively associated with various malignancies.
Figure 2. TNF and tumorigenesis. (A) TNF can impact multiple effectors of immune surveillance and exhibits either pro- (increased NK and CTL function), or anti- (increased suppressor cells, induction of PD-L1/B7-H1) surveillance activities. (B) TNF directly affects tumors through a complex contextual integration of signals driving a cellular response toward apoptosis/necrosis or survival, inflammation, and growth promotion. (C) TNF can initiate and progress autoimmunity, which can drive lymphoma development. AICD = activation-induced cell death; CTL = cytotoxic T-lymphocyte; DC = dendritic cell; IL = interleukin; MDSC = myeloid-derived suppressor cell; NK = natural killer; Th = T-helper lymphocyte; TNF = tumor necrosis factor. Adapted from: Aringer M, Smolen J. Complex cytokine effects in a complex autoimmune disease: tumor necrosis factor in systemic lupus erythematosus. Arthritis Res Ther 2003;5:174 © 2003 with permission from BioMed Central Ltd.
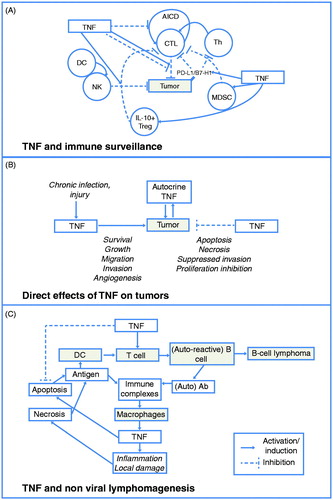
Tumor necrosis factor inhibitors: clinical trials and epidemiology data
While nonclinical models contribute to the understanding of the biological consequences of TNF inhibition, many of these models rely on rodent systems and/or are contrived, therefore their translatability to human disease is uncertain. The following section focuses on human data and reviews the overall risk of malignancy associated with TNFis, as well as the risk for specific tumor types.
Overall risk of malignancy in rheumatoid arthritis patients
Randomized clinical trials are underpowered and too short to determine the risk of rare events such as malignancy. Meta-analyses, large registries, and administrative database analyses have provided substantial evidence that malignancy rates are not increased overall with TNFis. Here, we focus on data from RA because this population is well defined regarding background rates of malignancy and has the largest amount of data examining the risk of malignancy associated with TNFis relative to other indications.
The attribution of malignancy risk to TNFis is complicated by the increased risk of malignancy in RA patients relative to the general population, as observed in the Danish Biologics (DANBIO) register (standardized incidence ratio [SIR] = 1.27; 95% CI, 1.08–1.49)Citation140 and the British Society for Rheumatology Biologics Register (BSRBR; SIR = 1.28; 95% CI, 1.10–1.48)Citation141. This is further confounded by the fact that these patients are also exposed to other cytotoxic or immunosuppressive drugs.
Hematologic malignancy
Lymphoma risk is increased in RA patients at least two-fold overall, and increased disease severity is correlated with increased risk of lymphomaCitation142. The RA population also has a higher rate of leukemia relative to the general population in the United States (SIR = 1.7; 95% CI, 1.2–2.4) and Sweden (SIR = 2.1 [95% CI, 1.7–2.5] and 2.2 [95% CI, 0.6–5.7] for prevalent and incident RA, respectively)Citation143,Citation144. In particular, patients with RA have a higher risk of acute myeloid leukemia, with an SIR of 2.4 (95% CI, 1.79–3.15)Citation145,Citation146. Taken together, these data show that patients with RA have an increased risk of leukemia and lymphoma relative to the general population.
Solid tumors
A decreased risk of colon and breast cancers in RA patients relative to the general population was reported in both the Spanish Registry for Adverse Events of Biological Therapies in Rheumatic Diseases (BIOBADASER) and the US National Data Bank (NDB) for Rheumatic DiseasesCitation143,Citation147. The observed decrease in colon cancer may be attributed to nonsteroidal anti-inflammatory drug use in this populationCitation148,Citation149. Similarly, decreased rates of prostate and female genital cancers were observed in RA patients in the BSRBR registryCitation141. Lung cancer may be increased in patients with RA compared with the general population, although conflicting results have been observedCitation147. Increased rates of smoking may explain increased lung cancer rates, because smoking is a risk factor for RACitation150. An increased risk of nonmelanoma skin cancer (NMSC) in RA (irrespective of treatment) has been reportedCitation151. Further, compared to patients with osteoarthritis, RA patients have an increased risk of developing NMSC (hazard ratio [HR] = 1.19; 95% CI, 1.01–1.41)Citation152. Disease duration is also associated with NMSC (HR = 1.01; 95% CI, 1.00–1.02; p = 0.004)Citation152.
Cancer risk associated with tumor necrosis factor inhibitors
Meta-analyses of pooled clinical trial data across combined approved indications do not indicate an overall increased risk of malignancy with TNFisCitation140,Citation144,Citation147,Citation153–165 (). Moreover, increased duration of TNFi exposure does not appear to increase malignancy risk. Results from the DANBIO registry showed no overall increased risk of malignancy based on the cumulative duration of TNFi exposureCitation140, a finding similar to that of an analysis of a large US observational studyCitation143. Additionally, individual TNFis do not show differences in risk; no significantly increased risk of malignancy was observed for the individual TNFis adalimumab, etanercept, or infliximab in the BIOBADASER 2.0 studyCitation166. In a study conducted using the Taiwan National Health Insurance Research Database, the risk of newly diagnosed cancer was compared between RA patients taking biologics (predominantly TNFis alone; 13.1% received rituximab) and matched subjects taking nonbiologic disease-modifying antirheumatic drug (nbDMARDs) only. The overall risk of cancer was significantly reduced in subjects receiving TNFis (HR = 0.63; 95% CI, 0.49–0.80)Citation154.
Table 4. Relative risk of malignancies in patients with RA and other inflammatory conditions (key studies).
Hematologic malignancies – lymphoma
Across clinical trials, observational studies, meta-analyses, and postmarketing analyses, the risk of lymphoma does not appear increased with TNFis relative to background therapy. Analyses that compared patients with RA treated with TNFis to the general population reported SIRs ranging from 3.5 to 5.8Citation15Citation6,Citation167. However, patients with more severe disease are more likely to receive TNFis, thus complicating calculations of true risk of malignancy. Data do exist that compare the risk associated with TNFis to other RA medications. In a meta-analysis of 10 randomized controlled RA trials, the odds ratio (OR) for lymphoma for all TNFis combined was not statistically significant (2.14; 95% CI, 0.55–8.38) compared with patients with RA who received an nbDMARD or placeboCitation168,Citation169. Similarly, Leombruno et al. found no significantly increased risk for lymphoma; the exposure-adjusted relative risk (RR) was 1.26 (95% CI, 0.53–3.01) for all TNFis combinedCitation168. There were also no major differences found for the individual TNFi agents, nor did high doses of TNFis confer a greater risk of lymphoma relative to patients who received nbDMARDsCitation168. A pooled analysis of three registries reported no increased risk of lymphoma associated with TNFis in patients with RA compared with nbDMARDs (RR = 1.11; 95% CI, 0.70–1.51)Citation158. In the Safety Assessment of Biological Therapeutics (SABER) study, the risk associated with TNFis versus nbDMARDs was also nonsignificant (HR = 1.25; 95% CI, 0.71–2.20)Citation157. In an analysis of a Swedish registry of 757 patients with RA treated with TNFis compared with 800 treated with nbDMARDs, a trend for an increased risk was found; the adjusted HR for lymphoma was 5.0 (95% CI, 0.9–27.9; p = 0.06) with an SIR of 11.5 (95% CI, 3.7–26.9); however, the population examined in this study was smaller than in other studiesCitation162. In the Taiwan National Health Insurance Research Database Study, the SIR for lymphoma was higher in subjects receiving TNFis (6.13; 95% CI, 3.26–10.49) than in subjects receiving nbDMARDs (2.52; 95% CI, 1.56–3.85), which was significantCitation154. Thus, there is no consistent evidence that TNFis increase the risk of lymphoma above the generally high background rate in the RA population.
Although hepatosplenic T-cell lymphoma was initially thought to be associated with TNFis in patients with inflammatory bowel disease, more recent evidence indicates an increased risk with TNFis when used in combination with a thiopurine (or with the thiopurine alone) but not with TNFi monotherapyCitation170.
Hematologic malignancy – leukemia
The risk of leukemia was not found to be increased with biologic TNFis in an analysis of the NDB for Rheumatic Diseases (OR = 1.2; 95% CI, 0.5–3.1)Citation143 and in a North American study (versus the general population; SIR = 1.3; 95% CI, 0.85–1.97)Citation165.
Solid tumors
There does not appear to be an overall increased risk of solid tumors associated with TNFis compared with nbDMARDs. An analysis of the BSRBR for RA demonstrated no significant difference in proportion or RR for any of the common site-specific solid cancers in RA patients treated with TNFis compared with nbDMARDsCitation148. Similarly, SABER reported no significant increase for any solid cancer associated with TNFis compared with nbDMARDsCitation157, while the most recent analysis of the Corrona RA Registry found a decreased risk of solid cancer associated with TNFis compared with methotrexate (HR = 0.21; 95% CI, 0.07–0.64)Citation160. Conversely, in the DANBIO registry, colon cancer was increased in patients treated with TNFis compared with nbDMARDs; however, the number of overall cases was low among the TNFi and nbDMARD groupsCitation140. Registry data have not indicated an increased incidence of lung cancer related to TNFi therapy above the already increased risk in the RA populationCitation143,Citation148; the risks of breast and lung cancers were reported to be decreased in patients treated with TNFis compared with methotrexate in the Corrona registryCitation160.
Nonmelanoma skin cancer
Overall, there appears to be a potential increased risk of NMSC associated with TNFis compared with nbDMARDs in RA patients. Two meta-analyses of randomized clinical trials reported no increased risk of NMSCCitation168,Citation169, and another reported a significant two-fold increase in the risk of NMSC associated with TNFis relative to comparator arms (HR = 2.02; 95% CI, 1.11–3.95)Citation155. Registry data from the BSRBR and administrative data from the SABER study showed statistically nonsignificantly increased risks of NMSC with TNFis compared with nbDMARDsCitation151,Citation157, and the Corrona registry showed a decreased risk of NMSC compared with methotrexateCitation160. Conversely, a study from the NDB reported a statistically significantly increased risk of NMSC associated with TNFis, although this was relative to the general US populationCitation143. A pooled analysis of four registries reported a statistically significantly increased risk of NMSC (pooled estimate = 1.33; 95% CI, 1.06–1.60). This analysis included data from the three registries (Corrona registry, BSRBR, and Antirheumatic Therapies in Sweden study [ARTIS]) with results that were nonsignificant compared with RA patients treated with nbDMARDs and a previous result from the NDB, which was the only study with a significant result included in the analysisCitation158,Citation171. A separate analysis from the NDB reported a trend toward an increased risk of NMSC associated with TNFis without concomitant methotrexate (HR = 1.24; 95% CI, 0.97–1.58) or when used in combination with methotrexate (HR = 1.97; 95% CI, 1.51–2.58)Citation152. The potential association between TNFis and NMSC requires further observation.
Melanoma
There have been mixed findings with respect to the association of TNFis and melanoma, and the overall risk is difficult to determine. An analysis of 29,423 patients with RA in 63 randomized controlled trials found an OR for melanoma associated with TNFi agents of 1.08 (95% CI, 0.11–10.21)Citation169. A separate meta-analysis reported a trend toward an increased risk of melanoma with TNFis based on the inclusion of two studies (pooled estimate = 1.79; 95% CI, 0.92–2.67)Citation158. Infliximab and etanercept were both associated with a statistically nonsignificantly increased risk of melanoma in the NDB, with ORs of 2.6 (95% CI, 1.0–6.7) and 2.4 (95% CI, 1.0–5.8), respectivelyCitation143. An analysis of the ARTIS registry found an increased risk of invasive melanoma compared with RA patients not treated with biologic drugs (HR = 1.5; 95% CI, 1.0–2.2) but no significantly increased risk of melanoma in situ (HR = 1.1; 95% CI, 0.5–2.1) associated with TNFis compared with other RA therapiesCitation172. Taken together, current evidence suggests a potential increased risk of melanoma overall, but the evidence is conflicting. However, although there does not appear to be an increased risk of in situ melanoma associated with TNFis, there may be increased risk of invasive melanoma.
Malignancy associated with viral infection
Patients treated with TNFis are at increased risk for certain viral infections such as herpes zosterCitation173 and reactivation of hepatitis BCitation174, raising the question of susceptibility to oncogenic viruses. Infection with certain viruses may lead to malignancy: EBV and lymphoma, HPV and cervical and anogenital cancers, polyomavirus and Merkel cell cancer, HHV-8 and Kaposi’s sarcoma. Certain types of HPV cause all cervical cancers; most anal cancers; and some vaginal, vulvar, penile, and oropharyngeal cancersCitation175. However, there is no evidence for an increased risk of these viral infection–associated malignancies with TNFi. In one study, patients who received infliximab, adalimumab, or etanercept showed no difference in EBV viral load and no difference in numbers of EBV-specific IFN-γ–producing T cells (for both latent-cycle and lytic-cycle peptides) between baseline and treatment week 12Citation111. Other clinical studies showed that TNFi therapy did not increase the EBV burden in patients with RA or ankylosing spondylitisCitation112,Citation113. Lower rates of female genital cancer (including cervical) were reported in the BSRBRCitation141, and there was no increase in female genital cancers in women with prior cervical carcinoma in situ treated with TNFis compared with those treated with nbDMARDs, although the number of patients was smallCitation176. Merkel cell carcinomas are rare, aggressive, cutaneous neuroendocrine tumors. Although rates of occurrence are not available, postmarketing case reports of Merkel cell carcinoma have occurred with TNFis, leading to mention of this development in the prescribing information for the majority of TNFisCitation42,Citation43,Citation46.
Recurrent malignancy
The data are limited, and it is unknown whether there is an overall increased risk of malignancy recurrence with TNFis across all indications; current guidelines do not provide clear guidance regarding the use of TNFis in patients with recent malignancies. Current European League Against Rheumatism guidelines have no specific recommendations regarding the treatment of patients with prior malignancies, although rituximab (a non-TNFi biologic) may be preferred in this scenarioCitation177. The British Society of Rheumatology guidelines recommend caution in the use of TNFis in patients with previous malignanciesCitation178. The American College of Rheumatology recommends the use of any biologic agent in patients with a history of a treated solid malignancy or NMSC >5 years agoCitation179; rituximab is recommended for patients with a more recent history (≤5 years) of a treated malignancy based on lack of evidence associating the use of this biologic DMARD with cancerCitation179.
Safety registries provide insight regarding the risk of malignancy recurrence with TNFis. In the BSRBR, Dixon et al. reported an incidence rate ratio (IRR) of incident cancer in TNFi-treated patients with RA with prior malignancies of 0.45 (95% CI, 0.09–2.17)Citation180. This study reported only incident malignancy occurring after the start of TNFi treatment (nbDMARD as control) and was not designed specifically to examine recurrence; in fact, many of the malignancies in those who reported prior malignancies could be classified as second primary tumorsCitation180. In the Rheumatoid Arthritis Observation of Biologic Therapy (RABBIT) study, malignancy recurrence rates were similar between patients with RA who received TNFis (45.5/1000 patient-years) and those who received nbDMARD therapy (31.4/1000 patient-years), with an IRR = 1.4 (95% CI, 0.5–5.5)Citation164. Most (14 of 15) of the second tumors were true recurrences of the primary tumor; nine patients received TNFis and five received nbDMARDsCitation164. The recurrent malignancies in patients treated with TNFis were breast (n = 4) and lung, bladder, liposarcoma, melanoma, and testicular cancers (n = 1 each)Citation164. A pooled analysis of the RABBIT and BSRBR studies reported an estimated IRR of 0.62 (95% CI, 0.04–1.20)Citation158. Data regarding cancer recurrence are also available from BIOBADASER 2.0, which compared patients with rheumatic diseases (including RA, juvenile idiopathic arthritis, ankylosing spondylitis, psoriatic arthritis) and psoriasis treated with TNFis to a cohort of patients not exposed to TNFis. In the TNFi-treated patients, 24 of 4694 were found to have a malignancy before TNFi exposure. The IRR for recurrent cancer in patients with prior malignancies was 5.22 (95% CI, 0.79–34.34), a nonsignificant trend that was complicated by small numbersCitation147.
A recent analysis of the ARTIS registry evaluated cancer recurrence in patients with RA and at least one diagnosis of breast cancer before initiating TNFi treatment. Comparing TNFi-treated patients with biologics-naive patients and adjusting for nodal status, type of surgery, and chemotherapy at index cancer, the HR was 1.1 (95% CI, 0.4–2.8). The authors of this report indicated that these results support current clinical guidelines, which state that TNFis may be initiated in patients with a >5-year history of treated breast cancerCitation181. Analyses of the BSRBR and ARTIS registries also specifically examined melanoma recurrence. In a small data set of patients with prior melanoma in the BSRBR, there were two definite (and one probable) melanoma recurrences in 17 patients exposed to TNFis and none in 10 patients not exposed to TNFisCitation180. An analysis of the ARTIS registry reported a nonsignificant three-fold increase in the risk of a second primary melanoma associated with TNFis relative to nbDMARDs in patients with RA, although only a total of 13 cases were reported (3 TNFi and 10 nbDMARD)Citation172.
Owing to the small number of cases reported, further analyses are needed to determine the recurrence risk, if any, of TNFi therapies in patients with prior malignancies.
Discussion and conclusions
Clinical and experimental animal data demonstrate that TNF can both promote and prevent tumor formation and that a complex, contextual integration of signals drives a cellular response toward apoptosis/necrosis or survival, inflammation, and growth promotion (). Mechanistic data indicate that key players in TNF signaling that shape this response include NF-κB and Jun kinase, as well as other factors intrinsic to normal or transformed cellsCitation64,Citation182. In addition to direct effects of TNF on tumors, TNF can variously affect immunity and the tumor microenvironment. Whereas TNF can promote immune surveillance designed to eliminate tumors, it can also drive chronic inflammation, autoimmunity, angiogenesis, and other processes that promote tumor initiation, growth, and spread (). The context-specific role of TNF in tumor regression or growth is evident in variable outcomes observed in rodent models ().
Human expression polymorphisms suggest that TNF is generally a tumor-promoting cytokine. Additionally, data from population studies, administrative databases, registries, meta-analyses, and observational studies demonstrate that overall rates of malignancy are not higher with TNFi therapy use among patients with RA, who have an elevated risk of certain malignancies compared with the general population. These data indicate that the risk of cancer associated with TNFi use is markedly different from the cancer risks experienced by individuals with primary (congenital) immune deficiencies, acquired deficiencies (e.g., HIV/AIDS), or those treated with therapeutics used to support organ engraftment, who have increased risks of both virally associated and nonviral cancersCitation5–14.
Taken as a whole, the available data indicate that elevated TNF is a risk factor for cancer, whereas its inhibition among patients with RA is not generally associated with an increased cancer risk, particularly for the range of cancers linked with immune suppression. More information is required to fully understand the risk of TNFi use regarding risk of skin malignancies and risk of cancer recurrence. Although this review focused on RA, which has the most robust data, there are reports in other disease indications showing no increased rate in malignancy with TNFis in juvenile idiopathic arthritisCitation183, psoriatic arthritisCitation157, and Crohn’s disease or inflammatory bowel diseaseCitation157,Citation184.
Because the immune system can both suppress and promote cancer, it is obligatory that an assessment of cancer risk among RA patients consider the risk of undertreating their inflammatory disease balanced against the use of immunosuppressive agents that impair immune surveillance. Such data, including outcomes with individual TNFis as presented here, are critical for informing patients and clinicians regarding the overall cancer risks they face given their disease and treatment options.
Developing an understanding of the cancer risk associated with TNFi use is confounded by a number of factors, including the nature of the underlying disease (and its severity), history of oncogenic viral infection, and the use of concomitant therapiesCitation1. Because more potent immunosuppressive agents are typically used in the treatment of more severe disease, dissociation of their effects as drivers of malignancy is especially challenging. As personalized medicine evolves and individual tumors are evaluated for markers, the role of TNF may be further understood.
Transparency
Declaration of funding
The preparation of the manuscript was funded by Amgen Inc.
Declaration of financial/other relationships
H.L., R.P., B.D.P., J.I., T.L.B., and M.H. have disclosed that they are employees of and own stock in Amgen Inc.
CMRO peer reviewers on this manuscript have received an honorarium from CMRO for their review work, but have no relevant financial or other relationships to disclose.
Acknowledgments
The authors acknowledge editorial assistance from Tim Peoples MA ELS CMPP of Amgen Inc., and Miranda Tradewell PhD of Complete Healthcare Communications, Inc., whose work was funded by Amgen Inc.
References
- Ponce RA, Gelzleichter T, Haggerty HG, et al. Immunomodulation and lymphoma in humans. J Immunotoxicol 2014;11:1-12
- Yaqub S, Aandahl EM. Inflammation versus adaptive immunity in cancer pathogenesis. Crit Rev Oncog 2009;15:43-63
- Balkwill F, Charles KA, Mantovani A. Smoldering and polarized inflammation in the initiation and promotion of malignant disease. Cancer Cell 2005;7:211-17
- Borrello MG, Degl'Innocenti D, Pierotti MA. Inflammation and cancer: the oncogene-driven connection. Cancer Lett 2008;267:262-70
- Kraus S, Arber N. Inflammation and colorectal cancer. Curr Opin Pharmacol 2009;9:405-10
- Mueller BU, Pizzo PA. Cancer in children with primary or secondary immunodeficiencies. J Pediatr 1995;126:1-10
- Filipovich AH, Mathur A, Kamat D, et al. Lymphoproliferative disorders and other tumors complicating immunodeficiencies. Immunodeficiency 1994;5:91-112
- Shiels MS, Pfeiffer RM, Gail MH, et al. Cancer burden in the HIV-infected population in the United States. J Natl Cancer Inst 2011;103:753-62
- Reed M, Cosgrove JM, Cindrich R, et al. Ten years later: a single hospital experience with malignancy in HIV/AIDS. J Surg Oncol 2010;102:282-6
- Engels EA, Biggar RJ, Hall HI, et al. Cancer risk in people infected with human immunodeficiency virus in the United States. Int J Cancer 2008;123:187-94
- Gallagher MP, Kelly PJ, Jardine M, et al. Long-term cancer risk of immunosuppressive regimens after kidney transplantation. J Am Soc Nephrol 2010;21:852-8
- Vajdic CM, van Leeuwen MT. Cancer incidence and risk factors after solid organ transplantation. Int J Cancer 2009;125:1747-54
- Grulich AE, van Leeuwen MT, Falster MO, Vajdic CM. Incidence of cancers in people with HIV/AIDS compared with immunosuppressed transplant recipients: a meta-analysis. Lancet 2007;370:59-67
- Kasiske BL, Snyder JJ, Gilbertson DT, Wang C. Cancer after kidney transplantation in the United States. Am J Transplant 2004;4:905-13
- Mellman I, Coukos G, Dranoff G. Cancer immunotherapy comes of age. Nature 2011;480:480-9
- Pardoll DM. The blockade of immune checkpoints in cancer immunotherapy. Nat Rev Cancer 2012;12:252-64
- Guo Y, Xu F, Lu T, et al. Interleukin-6 signaling pathway in targeted therapy for cancer. Cancer Treat Rev 2012;38:904-10
- Fridman WH, Pages F, Sautes-Fridman C, Galon J. The immune contexture in human tumours: impact on clinical outcome. Nat Rev Cancer 2012;12:298-306
- Galon J, Costes A, Sanchez-Cabo F, et al. Type, density, and location of immune cells within human colorectal tumors predict clinical outcome. Science 2006;313:1960-4
- Dunn GP, Bruce AT, Ikeda H, et al. Cancer immunoediting: from immunosurveillance to tumor escape. Nat Immunol 2002;3:991-8
- Vesely MD, Kershaw MH, Schreiber RD, Smyth MJ. Natural innate and adaptive immunity to cancer. Annu Rev Immunol 2011;29:235-71
- Biron CA, Brossay L. NK cells and NKT cells in innate defense against viral infections. Curr Opin Immunol 2001;13:458-64
- Malmgaard L. Induction and regulation of IFNs during viral infections. J Interferon Cytokine Res 2004;24:439-54
- de Visser KE, Eichten A, Coussens LM. Paradoxical roles of the immune system during cancer development. Nat Rev Cancer 2006;6:24-37
- Tan TT, Coussens LM. Humoral immunity, inflammation and cancer. Curr Opin Immunol 2007;19:209-16
- Mantovani A, Allavena P, Sica A, Balkwill F. Cancer-related inflammation. Nature 2008;454:436-44
- Colotta F, Allavena P, Sica A, et al. Cancer-related inflammation, the seventh hallmark of cancer: links to genetic instability. Carcinogenesis 2009;30:1073-81
- Tracey D, Klareskog L, Sasso EH, et al. Tumor necrosis factor antagonist mechanisms of action: a comprehensive review. Pharmacol Ther 2008;117:244-79
- Carswell EA, Old LJ, Kassel RL, et al. An endotoxin-induced serum factor that causes necrosis of tumors. Proc Natl Acad Sci USA 1975;72:3666-70
- Green S, Dobrjansky A, Carswell EA, et al. Partial purification of a serum factor that causes necrosis of tumors. Proc Natl Acad Sci USA 1976;73:381-5
- Shirai T, Yamaguchi H, Ito H, et al. Cloning and expression in Escherichia coli of the gene for human tumour necrosis factor. Nature 1985;313:803-6
- Wang AM, Creasey AA, Ladner MB, et al. Molecular cloning of the complementary DNA for human tumor necrosis factor. Science 1985;228:149-54
- Brennan FM, Chantry D, Jackson A, et al. Inhibitory effect of TNF alpha antibodies on synovial cell interleukin-1 production in rheumatoid arthritis. Lancet 1989;2:244-7
- Fong Y, Tracey KJ, Moldawer LL, et al. Antibodies to cachectin/tumor necrosis factor reduce interleukin 1 beta and interleukin 6 appearance during lethal bacteremia. J Exp Med 1989;170:1627-33
- Aggarwal BB, Henzel WJ, Moffat B, et al. Primary structure of human lymphotoxin derived from 1788 lymphoblastoid cell line. J Biol Chem 1985;260:2334-44
- Aggarwal BB, Moffat B, Harkins RN. Human lymphotoxin. Production by a lymphoblastoid cell line, purification, and initial characterization. J Biol Chem 1984;259:686-91
- Gray PW, Aggarwal BB, Benton CV, et al. Cloning and expression of cDNA for human lymphotoxin, a lymphokine with tumour necrosis activity. Nature 1984;312:721-4
- Aggarwal BB, Kohr WJ, Hass PE, et al. Human tumor necrosis factor. Production, purification, and characterization. J Biol Chem 1985;260:2345-54
- Pennica D, Nedwin GE, Hayflick JS, et al. Human tumour necrosis factor: precursor structure, expression and homology to lymphotoxin. Nature 1984;312:724-9
- Vilcek J, Lee TH. Tumor necrosis factor. New insights into the molecular mechanisms of its multiple actions. J Biol Chem 1991;266:7313-16
- Engelmann H, Aderka D, Rubinstein M, et al. A tumor necrosis factor-binding protein purified to homogeneity from human urine protects cells from tumor necrosis factor toxicity. J Biol Chem 1989;264:11974-80
- Simponi (golimumab). Full Prescribing Information. Horsham, PA, USA: Janssen Biotech Inc., 2014
- Remicade (infliximab). Full Prescribing Information. Horsham, PA, USA: Janssen Biotech, Inc., 2013
- Humira (adalimumab). Full Prescribing Information. North Chicago, IL, USA: Abbott Laboratories, 2013
- Cimzia (certolizumab pegol). Full Prescribing Information. Smyrna, GA, USA: UCB Inc., 2013
- Enbrel (etanercept). Full Prescribing Information. Thousand Oaks, CA, USA: Immunex Corporation, 2013
- Data on File. Thousand Oaks, CA, USA: Amgen Inc., 2014
- Brouckaert PG, Leroux-Roels GG, Guisez Y, et al. In vivo anti-tumour activity of recombinant human and murine TNF, alone and in combination with murine IFN-gamma, on a syngeneic murine melanoma. Int J Cancer 1986;38:763-9
- Balkwill FR, Lee A, Aldam G, et al. Human tumor xenografts treated with recombinant human tumor necrosis factor alone or in combination with interferons. Cancer Res 1986;46:3990-3
- Waters JP, Pober JS, Bradley JR. Tumour necrosis factor and cancer. J Pathol 2013;230:241-8
- Grunhagen DJ, de Wilt JH, van Geel AN, Eggermont AM. Isolated limb perfusion for melanoma patients – a review of its indications and the role of tumour necrosis factor-alpha. Eur J Surg Oncol 2006;32:371-80
- Grunhagen DJ, de Wilt JH, van Geel AN, et al. Isolated limb perfusion with TNF-alpha and melphalan in locally advanced soft tissue sarcomas of the extremities. Recent Results Cancer Res 2009;179:257-70
- Deroose JP, Eggermont AM, van Geel AN, et al. Long-term results of tumor necrosis factor alpha- and melphalan-based isolated limb perfusion in locally advanced extremity soft tissue sarcomas. J Clin Oncol 2011;29:4036-44
- Di Filippo F, Giacomini P, Rossi CR, et al. Hyperthermic isolated perfusion with tumor necrosis factor-alpha and doxorubicin for the treatment of limb-threatening soft tissue sarcoma: the experience of the Italian Society of Integrated Locoregional Treatment in Oncology (SITILO). In Vivo 2009;23:363-7
- Alexander HR Jr, Bartlett DL, Libutti SK, et al. Analysis of factors associated with outcome in patients undergoing isolated hepatic perfusion for unresectable liver metastases from colorectal center. Ann Surg Oncol 2009;16:1852-9
- Ruegg C, Yilmaz A, Bieler G, et al. Evidence for the involvement of endothelial cell integrin alphaVbeta3 in the disruption of the tumor vasculature induced by TNF and IFN-gamma. Nat Med 1998;4:408-14
- Zhang F, Decker K. Platelet-activating factor antagonists suppress the generation of tumor necrosis factor-alpha and superoxide induced by lipopolysaccharide or phorbol ester in rat liver macrophages. Eur Cytokine Netw 1994;5:311-17
- Balkwill F. Tumour necrosis factor and cancer. Nat Rev Cancer 2009;9:361-71
- Micheau O, Tschopp J. Induction of TNF receptor I-mediated apoptosis via two sequential signaling complexes. Cell 2003;114:181-90
- Beg AA, Baltimore D. An essential role for NF-kappaB in preventing TNF-alpha-induced cell death. Science 1996;274:782-4
- Luo JL, Maeda S, Hsu LC, et al. Inhibition of NF-kappaB in cancer cells converts inflammation-induced tumor growth mediated by TNFalpha to TRAIL-mediated tumor regression. Cancer Cell 2004;6:297-305
- Van Antwerp DJ, Martin SJ, Kafri T, et al. Suppression of TNF-alpha-induced apoptosis by NF-kappaB. Science 1996;274:787-9
- Pan S, An P, Zhang R, et al. Etk/Bmx as a tumor necrosis factor receptor type 2-specific kinase: role in endothelial cell migration and angiogenesis. Mol Cell Biol 2002;22:7512-23
- Wang X, Lin Y. Tumor necrosis factor and cancer, buddies or foes? 1. Acta Pharmacol Sin 2008;29:1275-88
- Balkwill F. TNF-α in promotion and progression of cancer. Cancer Metastasis Rev 2006;25:409-16
- Grivennikov SI, Greten FR, Karin M. Immunity, inflammation, and cancer. Cell 2010;140:883-99
- Ren G, Zhao X, Wang Y, et al. CCR2-dependent recruitment of macrophages by tumor-educated mesenchymal stromal cells promotes tumor development and is mimicked by TNFα. Cell Stem Cell 2012;11:812-24
- Perkins ND. The diverse and complex roles of NF-κB subunits in cancer. Nature Reviews Cancer 2012;12:121-32
- Volk A, Li J, Xin J, et al. Co-inhibition of NF-κB and JNK is synergistic in TNF-expressing human AML. J Exp Med 2014;211:1093-108
- DiDonato JA, Mercurio F, Karin M. NF-kappaB and the link between inflammation and cancer. Immunol Rev 2012;246:379-400
- Suganuma M, Okabe S, Marino MW, et al. Essential role of tumor necrosis factor alpha (TNF-alpha) in tumor promotion as revealed by TNF-alpha-deficient mice. Cancer Res 1999;59:4516-18
- Moore RJ, Owens DM, Stamp G, et al. Mice deficient in tumor necrosis factor-alpha are resistant to skin carcinogenesis. Nat Med 1999;5:828-31
- Bernert H, Sekikawa K, Radcliffe RA, et al. Trifa and I1-10 deficiencies have contrasting effects on lung tumor susceptibility: gender-dependent modulation of IL-10 haploinsufficiency. Mol Carcinog 2003;38:117-23
- Starcher B. Role for tumour necrosis factor-alpha receptors in ultraviolet-induced skin tumours. Br J Dermatol 2000;142:1140-7
- Karabela SP, Kairi CA, Magkouta S, et al. Neutralization of tumor necrosis factor bioactivity ameliorates urethane-induced pulmonary oncogenesis in mice. Neoplasia 2011;13:1143-51
- Knight B, Yeoh GC, Husk KL, et al. Impaired preneoplastic changes and liver tumor formation in tumor necrosis factor receptor type 1 knockout mice. J Exp Med 2000;192:1809-18
- Egberts JH, Cloosters V, Noack A, et al. Anti-tumor necrosis factor therapy inhibits pancreatic tumor growth and metastasis. Cancer Res 2008;68:1443-50
- Popivanova BK, Kitamura K, Wu Y, et al. Blocking TNF-alpha in mice reduces colorectal carcinogenesis associated with chronic colitis. J Clin Invest 2008;118:560-70
- Devoogdt N, Revets H, Kindt A, et al. The tumor-promoting effect of TNF-alpha involves the induction of secretory leukocyte protease inhibitor. J Immunol 2006;177:8046-52
- Stolting MN, Ferrari S, Handschin C, et al. Myoblasts inhibit prostate cancer growth by paracrine secretion of tumor necrosis factor-alpha. J Urol 2013;189:1952-9
- Ouyang W, Hu Y, Li J, et al. Direct evidence for the critical role of NFAT3 in benzo[a]pyrene diol-epoxide-induced cell transformation through mediation of inflammatory cytokine TNF induction in mouse epidermal Cl41 cells. Carcinogenesis 2007;28:2218-26
- Bates RC, Mercurio AM. Tumor necrosis factor-alpha stimulates the epithelial-to-mesenchymal transition of human colonic organoids. Mol Biol Cell 2003;14:1790-800
- Fleischman AG, Aichberger KJ, Luty SB, et al. TNFalpha facilitates clonal expansion of JAK2V617F positive cells in myeloproliferative neoplasms. Blood 2011;118:6392-8
- Choo MK, Sakurai H, Koizumi K, Saiki I. Stimulation of cultured colon 26 cells with TNF-alpha promotes lung metastasis through the extracellular signal-regulated kinase pathway. Cancer Lett 2005;230:47-56
- Shin SY, Kim JH, Baker A, et al. Transcription factor Egr-1 is essential for maximal matrix metalloproteinase-9 transcription by tumor necrosis factor alpha. Mol Cancer Res 2010;8:507-19
- Malik ST, Naylor MS, East N, et al. Cells secreting tumour necrosis factor show enhanced metastasis in nude mice. Eur J Cancer 1990;26:1031-4
- Orosz P, Kruger A, Hubbe M, et al. Promotion of experimental liver metastasis by tumor necrosis factor. Int J Cancer 1995;60:867-71
- Cubillos S, Scallon B, Feldmann M, Taylor P. Effect of blocking TNF on IL-6 levels and metastasis in a B16-BL6 melanoma/mouse model. Anticancer Res 1997;17:2207-11
- Kim R, Emi M, Tanabe K, Arihiro K. Tumor-driven evolution of immunosuppressive networks during malignant progression. Cancer Res 2006;66:5527-36
- Poschke I, Mougiakakos D, Kiessling R. Camouflage and sabotage: tumor escape from the immune system. Cancer Immunol Immunother 2011;60:1161-71
- Baxevanis CN, Voutsas IF, Tsitsilonis OE, et al. Compromised anti-tumor responses in tumor necrosis factor-alpha knockout mice. Eur J Immunol 2000;30:1957-66
- Iizuka K, Chaplin DD, Wang Y, et al. Requirement for membrane lymphotoxin in natural killer cell development. Proc Natl Acad Sci USA 1999;96:6336-40
- Xu J, Chakrabarti AK, Tan JL, et al. Essential role of the TNF-TNFR2 cognate interaction in mouse dendritic cell-natural killer cell crosstalk. Blood 2007;109:3333-41
- Eugster HP, Muller M, Karrer U, et al. Multiple immune abnormalities in tumor necrosis factor and lymphotoxin-alpha double-deficient mice. Int Immunol 1996;8:23-36
- Marino MW, Dunn A, Grail D, et al. Characterization of tumor necrosis factor-deficient mice. Proc Natl Acad Sci USA 1997;94:8093-8
- De Togni P, Goellner J, Ruddle NH, et al. Abnormal development of peripheral lymphoid organs in mice deficient in lymphotoxin. Science 1994;264:703-7
- Kim EY, Teh SJ, Yang J, et al. TNFR2-deficient memory CD8 T cells provide superior protection against tumor cell growth. J Immunol 2009;183:6051-7
- Kondo A, Yamashita T, Tamura H, et al. Interferon-gamma and tumor necrosis factor-alpha induce an immunoinhibitory molecule, B7-H1, via nuclear factor-kappaB activation in blasts in myelodysplastic syndromes. Blood 2010;116:1124-31
- Zhao X, Rong L, Zhao X, et al. TNF signaling drives myeloid-derived suppressor cell accumulation. J Clin Invest 2012;122:4094-104
- Prevost-Blondel A, Roth E, Rosenthal FM, Pircher H. Crucial role of TNF-alpha in CD8 T cell-mediated elimination of 3LL-A9 Lewis lung carcinoma cells in vivo. J Immunol 2000;164:3645-51
- Swann JB, Vesely MD, Silva A, et al. Demonstration of inflammation-induced cancer and cancer immunoediting during primary tumorigenesis. Proc Natl Acad Sci USA 2008;105:652-6
- Kammertoens T, Qin Z, Briesemeister D, et al. B-cells and IL-4 promote methylcholanthrene-induced carcinogenesis but there is no evidence for a role of T/NKT-cells and their effector molecules (Fas-ligand, TNF-alpha, perforin). Int J Cancer 2012;131:1499-508
- Tanigawa K, Craig RA, Stoolman LM, Chang AE. Effects of tumor necrosis factor-alpha on the in vitro maturation of tumor-reactive effector T cells. J Immunother 2000;23:528-35
- Ito D, Back TC, Shakhov AN, et al. Mice with a targeted mutation in lymphotoxin-alpha exhibit enhanced tumor growth and metastasis: impaired NK cell development and recruitment. J Immunol 1999;163:2809-15
- Korner H, Cook M, Riminton DS, et al. Distinct roles for lymphotoxin-alpha and tumor necrosis factor in organogenesis and spatial organization of lymphoid tissue. Eur J Immunol 1997;27:2600-9
- Peschon JJ, Torrance DS, Stocking KL, et al. TNF receptor-deficient mice reveal divergent roles for p55 and p75 in several models of inflammation. J Immunol 1998;160:943-52
- Grimm M, Lazariotou M, Kircher S, et al. Tumor necrosis factor-alpha is associated with positive lymph node status in patients with recurrence of colorectal cancer – indications for anti-TNF-alpha agents in cancer treatment. Anal Cell Pathol (Amst) 2010;33:151-63
- Vohra N, Verhaegen M, Martin L, et al. TNF-alpha-treated DC exacerbates disease in a murine tumor metastasis model. Cancer Immunol Immunother 2010;59:729-36
- Kim DH, Kim EM, Lee EH, et al. Human papillomavirus 16E6 suppresses major histocompatibility complex class I by upregulating lymphotoxin expression in human cervical cancer cells. Biochem Biophys Res Commun 2011;409:792-8
- Wooley PH, Dutcher J, Widmer MB, Gillis S. Influence of a recombinant human soluble tumor necrosis factor receptor FC fusion protein on type II collagen-induced arthritis in mice. J Immunol 1993;151:6602-7
- Miceli-Richard C, Gestermann N, Amiel C, et al. Effect of methotrexate and anti-TNF on Epstein-Barr virus T-cell response and viral load in patients with rheumatoid arthritis or spondylarthropathies. Arthritis Res Ther 2009;11:R77
- Balandraud N, Guis S, Meynard JB, et al. Long-term treatment with methotrexate or tumor necrosis factor alpha inhibitors does not increase Epstein–Barr virus load in patients with rheumatoid arthritis. Arthritis Rheum 2007;57:762-7
- Couderc M, Payet S, Henquell C, et al. TNFalpha antagonist therapy does not increase the Epstein-Barr virus burden in patients with rheumatoid arthritis or ankylosing spondylitis. Joint Bone Spine 2010;77:414-17
- Coussens LM, Werb Z. Inflammation and cancer. Nature 2002;420:860-7
- Maccio A, Madeddu C. Inflammation and ovarian cancer. Cytokine 2012;58:133-47
- Wu Y, Zhou BP. TNF-alpha/NF-kappaB/Snail pathway in cancer cell migration and invasion. Br J Cancer 2010;102:639-44
- Bauer J, Namineni S, Reisinger F, et al. Lymphotoxin, NF-kB, and cancer: the dark side of cytokines. Dig Dis 2012;30:453-68
- Kroeger KM, Carville KS, Abraham LJ. The -308 tumor necrosis factor-alpha promoter polymorphism effects transcription. Mol Immunol 1997;34:391-9
- Louis E, Franchimont D, Piron A, et al. Tumour necrosis factor (TNF) gene polymorphism influences TNF-alpha production in lipopolysaccharide (LPS)-stimulated whole blood cell culture in healthy humans. Clin Exp Immunol 1998;113:401-6
- Gorouhi F, Islami F, Bahrami H, Kamangar F. Tumour-necrosis factor-A polymorphisms and gastric cancer risk: a meta-analysis. Br J Cancer 2008;98:1443-51
- Jin G, Zhao Y, Sun S, Kang H. Association between the tumor necrosis factor alpha gene -308G> A polymorphism and the risk of breast cancer: a meta-analysis. Tumour Biol 2014;35:12091-8
- Min L, Chen D, Qu L, Shou C. Tumor necrosis factor-a polymorphisms and colorectal cancer risk: a meta-analysis. PLoS One 2014;9:e85187
- Rokkas T, Sechopoulos P, Pistiolas D, et al. Population differences concerning TNF-alpha gene polymorphisms in gastric carcinogenesis based on meta-analysis. Ann Gastroenterol 2014;27:139-48
- Shen C, Sun H, Sun D, et al. Polymorphisms of tumor necrosis factor-alpha and breast cancer risk: a meta-analysis. Breast Cancer Res Treat 2011;126:763-70
- Wang J, Jin X, Wang H, et al. The -308G/A polymorphism of the tumor necrosis factor-alpha gene is associated with the risk of upper aerodigestive tract cancer: a meta-analysis. Tohoku J Exp Med 2013;229:245-54
- Xie H, Yao H, Huo Y, et al. Association between TNF-alpha gene 308G>A polymorphism and lung cancer risk: a meta-analysis. Tumour Biol 2014;35:9693-9
- Yang Y, Luo C, Feng R, Bi S. The TNF-alpha, IL-1B and IL-10 polymorphisms and risk for hepatocellular carcinoma: a meta-analysis. J Cancer Res Clin Oncol 2011;137:947-52
- Zhai K, Ding J, Zhou Y. Different role of tumor necrosis factor-alpha polymorphism in non-Hodgkin lymphomas among Caucasian and Asian populations: a meta-analysis. Int J Mol Sci 2014;15:7684-98
- Zhang HL, Zhang YJ. A systemic assessment of the association between tumor necrosis factor alpha 308 G/A polymorphism and risk of cervical cancer. Tumour Biol 2013;34:1659-65
- Feng RN, Liu GY, Wang C, et al. TNF polymorphisms and cancer. Asian Pac J Cancer Prev 2012;13:3007
- Cen G, Wu W. Association between tumor necrosis factor-alpha 857C/T polymorphism and gastric cancer: a meta-analysis. Tumour Biol 2013;34:3383-8
- Tsukasaki K, Miller CW, Kubota T, et al. Tumor necrosis factor alpha polymorphism associated with increased susceptibility to development of adult T-cell leukemia/lymphoma in human T-lymphotropic virus type 1 carriers. Cancer Res 2001;61:3770-4
- Menke V, van Zoest KP, Moons LM, et al. NcoI TNF-beta gene polymorphism and TNF expression are associated with an increased risk of developing Barrett's esophagus and esophageal adenocarcinoma. Scand J Gastroenterol 2012;47:378-86
- Foa R, Massaia M, Cardona S, et al. Production of tumor necrosis factor-alpha by B-cell chronic lymphocytic leukemia cells: a possible regulatory role of TNF in the progression of the disease. Blood 1990;76:393-400
- Barak V, Nisman B, Polliack A. The tumor necrosis factor family and correlation with disease activity and response to treatment in hairy cell leukemia. Eur J Haematol 1999;62:71-5
- Freedman MH, Cohen A, Grunberger T, et al. Central role of tumour necrosis factor, GM-CSF, and interleukin 1 in the pathogenesis of juvenile chronic myelogenous leukaemia. Br J Haematol 1992;80:40-8
- Charles KA, Kulbe H, Soper R, et al. The tumor-promoting actions of TNF-alpha involve TNFR1 and IL-17 in ovarian cancer in mice and humans. J Clin Invest 2009;119:3011-23
- Senthilkumar C, Niranjali S, Jayanthi V, et al. Molecular and histological evaluation of tumor necrosis factor-alpha expression in Helicobacter pylori-mediated gastric carcinogenesis. J Cancer Res Clin Oncol 2011;137:577-83
- Suganuma M, Kuzuhara T, Yamaguchi K, Fujiki H. Carcinogenic role of tumor necrosis factor-alpha inducing protein of Helicobacter pylori in human stomach. J Biochem Mol Biol 2006;39:1-8
- Dreyer L, Mellemkjaer L, Andersen AR, et al. Incidences of overall and site specific cancers in TNFalpha inhibitor treated patients with rheumatoid arthritis and other arthritides – a follow-up study from the DANBIO Registry. Ann Rheum Dis 2013;72:79-82
- Mercer LK, Davies R, Galloway JB, et al. Risk of cancer in patients receiving non-biologic disease-modifying therapy for rheumatoid arthritis compared with the UK general population. Rheumatology 2013;52:91-8
- Baecklund E, Iliadou A, Askling J, et al. Association of chronic inflammation, not its treatment, with increased lymphoma risk in rheumatoid arthritis. Arthritis Rheum 2006;54:692-701
- Wolfe F, Michaud K. Biologic treatment of rheumatoid arthritis and the risk of malignancy: analyses from a large US observational study. Arthritis Rheum 2007;56:2886-95
- Askling J, Fored CM, Baecklund E, et al. Haematopoietic malignancies in rheumatoid arthritis: lymphoma risk and characteristics after exposure to tumour necrosis factor antagonists. Ann Rheum Dis 2005;64:1414-20
- Hemminki K, Li X, Sundquist K, Sundquist J. Cancer risk in hospitalized rheumatoid arthritis patients. Rheumatology 2008;47:698-701
- Ramadan SM, Fouad TM, Summa V, et al. Acute myeloid leukemia developing in patients with autoimmune diseases. Haematologica 2012;97:805-17
- Carmona L, Abasolo L, Descalzo MA, et al. Cancer in patients with rheumatic diseases exposed to TNF antagonists. Semin Arthritis Rheum 2011;41:71-80
- Mercer LK, Lunt M, Low AL, et al. Risk of solid cancer in patients exposed to anti-tumour necrosis factor therapy: results from the British Society for Rheumatology Biologics Register for Rheumatoid Arthritis. Ann Rheum Dis 2014; [Epub]. doi 10.1136/annrheumdis-2013-204851. March 31, 2014
- Kauppi M, Pukkala E, Isomaki H. Low incidence of colorectal cancer in patients with rheumatoid arthritis. Clin Exp Rheumatol 1996;14:551-3
- Symmons DP, Bankhead CR, Harrison BJ, et al. Blood transfusion, smoking, and obesity as risk factors for the development of rheumatoid arthritis. Results from a primary care-based incident case-control study in Norfolk, England. Arthritis Rheum 1997;40:1955-61
- Mercer LK, Green AC, Galloway JB, et al. The influence of anti-TNF therapy upon incidence of keratinocyte skin cancer in patients with rheumatoid arthritis: longitudinal results from the British Society for Rheumatology Biologics Register. Ann Rheum Dis 2012;71:869-74
- Chakravarty EF, Michaud K, Wolfe F. Skin cancer, rheumatoid arthritis, and tumor necrosis factor inhibitors. J Rheumatol 2005;32:2130-5
- Michaud TL, Rho YH, Shamliyan T, et al. The comparative safety of TNF inhibitors in rheumatoid arthritis – a meta-analysis update of 44 randomized controlled trials. Am J Med 2014;127:1208-32
- Wu C-Y, Chen D-Y, Shen J-L, et al. The risk of cancer in patients with rheumatoid arthritis taking tumor necrosis factor antagonists: a nationwide cohort study. Arthritis Res Ther 2014;16:449
- Askling J, Fahrbach K, Nordstrom B, et al. Cancer risk with tumor necrosis factor alpha (TNF) inhibitors: meta-analysis of randomized controlled trials of adalimumab, etanercept, and infliximab using patient level data. Pharmacoepidemiol Drug Saf 2011;20:119-30
- Gottlieb AB, Gordon K, Giannini EH, et al. Clinical trial safety and mortality analyses in patients receiving etanercept across approved indications. J Drugs Dermatol 2011;10:289-300
- Haynes K, Beukelman T, Curtis JR, et al. Tumor necrosis factor alpha inhibitor therapy and cancer risk in chronic immune-mediated diseases. Arthritis Rheum 2013;65:48-58
- Mariette X, Matucci-Cerinic M, Pavelka K, et al. Malignancies associated with tumour necrosis factor inhibitors in registries and prospective observational studies: a systematic review and meta-analysis. Ann Rheum Dis 2011;70:1895-904
- Burmester GR, Panaccione R, Gordon KB, et al. Adalimumab: long-term safety in 23 458 patients from global clinical trials in rheumatoid arthritis, juvenile idiopathic arthritis, ankylosing spondylitis, psoriatic arthritis, psoriasis and Crohn's disease. Ann Rheum Dis 2013;72:517-24
- Solomon DH, Kremer JM, Fisher M, et al. Comparative cancer risk associated with methotrexate, other non-biologic and biologic disease-modifying anti-rheumatic drugs. Semin Arthritis Rheum 2014;43:489-97
- Askling J, Fored CM, Brandt L, et al. Risks of solid cancers in patients with rheumatoid arthritis and after treatment with tumour necrosis factor antagonists. Ann Rheum Dis 2005;64:1421-6
- Geborek P, Bladstrom A, Turesson C, et al. Tumour necrosis factor blockers do not increase overall tumour risk in patients with rheumatoid arthritis, but may be associated with an increased risk of lymphomas. Ann Rheum Dis 2005;64:699-703
- Pallavicini FB, Caporali R, Sarzi-Puttini P, et al. Tumour necrosis factor antagonist therapy and cancer development: analysis of the LORHEN registry. Autoimmun Rev 2010;9:175-80
- Strangfeld A, Hierse F, Rau R, et al. Risk of incident or recurrent malignancies among patients with rheumatoid arthritis exposed to biologic therapy in the German biologics register RABBIT. Arthritis Res Ther 2010;12:R5
- Setoguchi S, Solomon DH, Weinblatt ME, et al. Tumor necrosis factor alpha antagonist use and cancer in patients with rheumatoid arthritis. Arthritis Rheum 2006;54:2757-64
- Wiens A, Venson R, Correr CJ, et al. Meta-analysis of the efficacy and safety of adalimumab, etanercept, and infliximab for the treatment of rheumatoid arthritis. Pharmacotherapy 2010;30:339-53
- Weinblatt ME, Bathon JM, Kremer JM, et al. Safety and efficacy of etanercept beyond 10 years of therapy in North American patients with early and longstanding rheumatoid arthritis. Arthritis Care Res (Hoboken) 2011;63:373-82
- Leombruno JP, Einarson TR, Keystone EC. The safety of anti-tumour necrosis factor treatments in rheumatoid arthritis: meta and exposure-adjusted pooled analyses of serious adverse events. Ann Rheum Dis 2009;68:1136-45
- Lopez-Olivo MA, Tayar JH, Martinez-Lopez JA, et al. Risk of malignancies in patients with rheumatoid arthritis treated with biologic therapy: a meta-analysis. JAMA 2012;308:898-908
- Deepak P, Sifuentes H, Sherid M, et al. T-cell non-Hodgkin's lymphomas reported to the FDA AERS with tumor necrosis factor-alpha (TNF-α) inhibitors: results of the REFURBISH study. Am J Gastroenterol 2013;108:99-105
- Mariette X, Reynolds AV, Emery P. Updated meta-analysis of non-melanoma skin cancer rates reported from prospective observational studies in patients treated with tumour necrosis factor inhibitors. Ann Rheum Dis 2012;71:e2
- Raaschou P, Simard JF, Holmqvist M, et al. Rheumatoid arthritis, anti-tumour necrosis factor therapy, and risk of malignant melanoma: nationwide population based prospective cohort study from Sweden. BMJ 2013;346:f1939
- Ramiro S, Gaujoux-Viala C, Nam JL, et al. Safety of synthetic and biological DMARDs: a systematic literature review informing the 2013 update of the EULAR recommendations for management of rheumatoid arthritis. Ann Rheum Dis 2014;73:529-35
- Ali T, Kaitha S, Mahmood S, et al. Clinical use of anti-TNF therapy and increased risk of infections. Drug Healthc Patient Saf 2013;5:79-99
- National Cancer Institute. HPV and cancer: fact sheet. 2014. Available at: http://www.cancer.gov/cancertopics/factsheet/Risk/HPV/print [Last accessed 6 June 2014]
- Mercer LK, Low ASL, Galloway JB, et al. Anti-TNF therapy in women with rheumatoid arthritis with a history of carcinoma in situ of the cervix. Ann Rheum Dis 2013;72:143-4
- Smolen JS, Landewé R, Breedveld FC, et al. EULAR recommendations for the management of rheumatoid arthritis with synthetic and biological disease-modifying antirheumatic drugs. Ann Rheum Dis 2010;69:964-75
- Ding T, Ledingham J, Luqmani R, et al. BSR and BHPR rheumatoid arthritis guidelines on safety of anti-TNF therapies. Rheumatology 2010;49:2217-19
- Singh JA, Furst DE, Bharat A, et al. 2012 Update of the 2008 American College of Rheumatology recommendations for the use of disease-modifying antirheumatic drugs and biologic agents in the treatment of rheumatoid arthritis. Arthritis Care Res 2012;64:625-39
- Dixon W, Watson K, Lunt M, et al. Influence of anti-tumor necrosis factor therapy on cancer incidence in patients with rheumatoid arthritis who have had a prior malignancy: results from the British Society for Rheumatology Biologics Register. Arthritis Care Res 2010;62:755-63
- Raaschou P, Frisell T, Askling J, et al. TNF inhibitor therapy and risk of breast cancer recurrence in patients with rheumatoid arthritis: a nationwide cohort study. Ann Rheum Dis 2014; [Epub]. doi:10.1136/annrheumdis-2014-205745. August 8, 2014
- Karin M. NF-kappaB as a critical link between inflammation and cancer. Cold Spring Harb Perspect Biol 2009;1:a000141
- Beukelman T, Haynes K, Curtis JR, et al. Rates of malignancy associated with juvenile idiopathic arthritis and its treatment. Arthritis Rheum 2012;64:1263-71
- Peyrin-Biroulet L, Deltenre P, De Suray N, et al. Efficacy and safety of tumor necrosis factor antagonists in Crohn's disease: meta-analysis of placebo-controlled trials. Clin Gastroenterol Hepatol 2008;6:644-53