Abstract
Despite the widespread availability of insulin pumps, continuous glucose sensors, and insulin analogs with rapid-acting pharmacokinetic profiles, most people with type 1 diabetes fail to meet recommended glycemic targets, rates of severe hypoglycemia remain unacceptably high, and the burden of care on patients and loved ones exacts an enormous psychosocial toll. The combination of continuous glucose monitoring with insulin delivery into an integrated automated system promises to improve diabetes control while at the same time reduce the burden of care. A wide variety of automated insulin delivery systems, ranging in scope from simple pump suspension to reduce hypoglycemia, to complex multiple hormone systems under separate regulation and delivery, have been studied in both controlled inpatient settings and more free-ranging outpatient environments. Preliminary findings have been positive, with most studies demonstrating reduction in overall glucose levels, increased time-in-target range, and reductions in exposure to hypoglycemia. As these systems move closer to commercialization, the focus of ongoing efforts will need to address the continuing challenges of sensor accuracy and reliability, connectivity issues, and human factors considerations.
1. Introduction
The combination of glucose monitoring and insulin delivery into a single device has the potential to improve glycemic control in patients with type 1 diabetes (T1D) while simultaneously enhancing quality of life. In a closed-loop (CL) artificial (or ‘bionic’) pancreas system, a continuous glucose monitor (CGM) communicates with an insulin pump that delivers insulin based on a control algorithm. Small feasibility studies have demonstrated the safety and preliminary efficacy of this approach to improve overall glucose control and reduce exposure to hypoglycemia. Historically, these systems have first been studied in closely monitored hospital inpatient settings, and as the algorithms used to determine insulin delivery have been validated and optimized, have then been transitioned to outpatient settings with variable degrees of in-person and remote monitoring capabilities.
2. Milestones in artificial pancreas
A spectrum of automated insulin delivery systems, ranging in scope from simple pump suspension to reduce hypoglycemia, to complex multiple hormone systems under separate regulation and delivery, are all under development (). The most basic modulation of insulin delivery based on CGM data is the suspension of basal insulin delivery for low sensor readings, the so-called threshold suspend system. Threshold suspend pumps have been shown to reduce exposure to hypoglycemia, without increasing exposure to hyperglycemia Citation[1]; these devices are now commercially available and in widespread use. The ability to track sensor glucose levels and suspend insulin delivery before hypoglycemia occurs, or ‘predictive’ suspend system, has also shown promise to reduce hypoglycemic exposure in the home environment Citation[2]; commercial versions of these devices are currently in large-scale evaluation. It should be noted that with these systems, there is no dynamic modulation of basal insulin; pre-defined basal rates are either continued as programmed or suspended, based on CGM levels and trends.
Figure 1. Schematic of approaches to an artificial pancreas. A broad spectrum of automated insulin delivery systems is under development, ranging from insulin suspension for reaching or approaching hypoglycemia, through night-time only closed-loop (CL) to full-day CL. Some systems retain the requirement for manual insulin dosing for meals (HCL), whereas others are fully automated. Finally, systems may include insulin only (Unihorm) or may incorporate other hormones (Bihorm). As these systems increase in automation and complexity, the need for user input decreases, but may be met by a compensatory increase in challenge to commercialization.
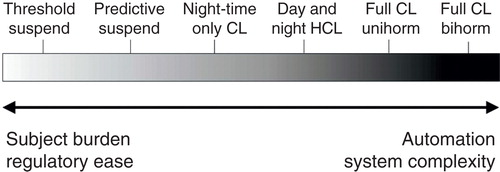
Whereas the previous examples of combination sensor-augmented pump (SAP) systems can be characterized by a very basic suspend/resume control algorithm, more sophisticated artificial pancreas systems with dynamic minute-to-minute regulation of insulin delivery have simultaneously also been under development and clinical evaluation. Early inpatient CL studies using relatively straightforward proportional-integral-derivative (PID) control algorithms demonstrated ability to maintain glucose within target range, particularly overnight, but fully automated approaches were limited by early postprandial hyperglycemia and late post-meal hypoglycemia Citation[3]. Through inpatient studies, it was discovered that small ‘priming’ insulin boluses improved glycemic control, but this required involvement of the patient to announce the meal and/or enter the amount of carbohydrate intake Citation[4]. The use of more complex model-predictive-control (MPC) algorithms, fuzzy-logic, and/or model-based modifications to the PID algorithms has mitigated the problem of late post-meal hypoglycemia, although the continued problem of large prandial glycemic excursions have led most investigators to rely on hybrid systems that incorporate manual boluses of some sort for meals Citation[5].
Over the last 2 years, artificial pancreas studies have begun to take place in the outpatient setting, more closely simulating real-life conditions. As with the initial inpatient studies, early outpatient trials have shown most benefit in the nighttime period. A study of 56 adolescents in a camp setting showed a 10% reduction in mean glucose and 68% reduction in episodes of hypoglycemia during a single-night of CL control as compared to one night in an open-loop SAP therapy mode Citation[6]. Subsequent studies using this system for periods of up to 6 weeks in the home setting have yielded similar beneficial results on reduction of mean glucose and hypoglycemia Citation[7]. Hovorka et al. demonstrated improvements in overall nocturnal glucose and reduction of hyperglycemia without an increase in hypoglycemia in an MPC-based system in periods of up to 4 weeks of night-time only control in the home setting Citation[8].
Outpatient studies incorporating both daytime and nighttime periods of evaluation present the greatest challenge to CL systems, which must now deal with both meal and exercise disturbances. The first randomized study utilizing an MPC-based system in the outpatient setting featured 18 adult patients with T1D during 40 h of CL vs open-loop control in a supervised home environment. CL control was associated with less time spent in hypoglycemia at the expense of a slightly higher overall average glucose level Citation[9]. The longest outpatient CL day and night study to date, consisting of 17 adults over 1 week in the actual home environment, showed that the percentage of time in glucose target range was significantly higher in CL patients as compared to SAP for both day and night time periods. Overall average glucose levels were lower in CL condition, with similar time spent below target, indicating similar risk for hypoglycemia between the groups Citation[10]. It should be noted that these studies both utilize hybrid systems, in that manual boluses were required for meals.
All of the systems and studies discussed so far can be characterized as single-hormone systems, utilizing insulin alone in the artificial pancreas. The Damiano group in Boston has pioneered a two-hormone system including both insulin and glucagon, under separate algorithmic control and delivered via separate pumps. In a crossover study of 20 adults and 32 adolescents during 5 days of bihormonal CL control vs 5 days of conventional insulin-only SAP treatment, the bihormonal bionic pancreas lowered mean blood glucose in both adult and adolescent groups, and the adult cohort spent less time in hypoglycemia. In adolescents, there was a greater than 50% reduction in amount of carbohydrates given to treat hypoglycemia with the bionic pancreas Citation[11]. The use of incretin mimetics have recently shown promise as an adjunct to insulin in a CL system, presumably through the blunting of inappropriate prandial glucagon release Citation[12].
3. Expert opinion
Although significant progress has been made in the combination of glucose monitoring and insulin delivery into an artificial pancreas, there are remaining challenges to overcome before this technology can be safely and effectively applied to large segments of the population, either due to limitations to the devices themselves or to characteristics of the subjects who will be using them. Most obviously, the accuracy and reliability of continuous glucose sensors, while continuing to improve, remains an important factor. Commercial sensors at the present time generally achieve overall accuracy levels of 10 – 12% median absolute relative deviation Citation[13], but very large errors of 50% or more still occur in as much as 5% of readings, even under ideal research-environment calibration conditions. It is reasonable to expect that sensors calibrated by typical users under real-use conditions may be even less accurate and reliable. Further, transient losses in the sensor signal and accuracy due to positional pressure-induced attenuation (typically occurring during sleep) raise the concern of inappropriate suspension of insulin delivery during periods of sensor signal loss, interpreted erroneously as falling glucose, and conversely, inappropriate insulin delivery with resumption of sensor signal that mimics a rise in plasma glucose. Novel control algorithms have been developed to address this phenomenon Citation[14].
Limitations in artificial pancreas effectiveness and safety due to hormone delivery factors were recognized in the early inpatient feasibility studies of CL systems. The relatively slow rates of insulin absorption from the subcutaneous compartment, described earlier, have been primarily addressed using model-based algorithms and manual meal announcement strategies. However, these approaches may not be robust enough to account for the wide inter- and even intra-subject variations in insulin absorption rates due to local lipohypertrophy or fibrosis/atrophy. Even more importantly, variations in insulin sensitivity and action must be considered. On a daily basis, changes in insulin sensitivity related to exercise may require additional inputs to the system in the form of manual ‘exercise announcement’ or input from heart rate monitor or accelerometer. Additionally, accommodations to the systems for changes to insulin sensitivity related to stress, illness, puberty, and menses, have yet to be fully explored. Finally, issues with glucagon delivery must be considered: current bihormonal systems utilizing glucagon rely on daily reconstitution of a glucagon solution for pump delivery, which limits its practical use. As newer liquid-stable formulations of glucagon become available, these systems will need to be re-evaluated for safety and effectiveness.
Finally, challenges with an artificial pancreas system relate to its use as a commercial medical device. Device factors are critical to the adoption of these systems for clinical use, including issues such as device size and weight, visual display, battery life, alarm fatigue, body footprint, and potential connectivity to the ‘cloud’ for remote-assisted safety monitoring or intervention. Systems that incorporate devices of multiple manufacturers will require collaborative efforts during the regulatory approval process and sophisticated licensing agreements for commercialization. There will undoubtedly be great variability in how people with type 1 diabetes will interact with these systems, and these human factors are just beginning to be explored: patient selection, device training, use in pediatric, elderly, and pregnant populations, and psychosocial and quality of life factors must all be considered as these devices near regulatory approval and commercial availability. Finally, third-party payers will likely require demonstrable proof that the additional costs for these devices will translate into overall cost savings through better long-term health of persons with diabetes: reduced hospitalizations for severe hypoglycemia, for example, or reduced costs related to care for long-term complications.
Declaration of interest
The authors were supported by National Institutes of Health/National Institute of Diabetes and Digestive and Kidney Diseases (NIH/NIDDK) grant R01 DK085618 and the Michael D Ryan & Rosemary McNicolas Ryan Pediatric Diabetes Research Fund. SA Weinzimer serves as a consultant for the following commercial entities involved in the production and sale of insulin pumps and/or continuous glucose sensors: Animas, Insulet, Medtronic and Tandem, and WV Tamborlane is on the advisory board for Medtronic. The authors have no other relevant affiliations or financial involvement with any organization or entity with a financial interest in or financial conflict with the subject matter or materials discussed in the manuscript apart from those disclosed.
Notes
Bibliography
- Bergenstal RM, Klonoff DC, Garg SK, et al. Threshold-based insulin pump interruption for reduction of hypoglycemia. N Engl J Med 2013;369:224-32
- Maahs DM, Calhoun P, Buckingham BA, et al. A randomized trial of a home system to reduce nocturnal hypoglycemia in type 1 diabetes. Diabetes Care 2014;37:1885-91
- Steil GM, Rebrin K, Darwin C, et al. Feasibility of automating insulin delivery for the treatment of type 1 diabetes. Diabetes 2006;55:3344-50
- Weinzimer SA, Steil GM, Swan KL, et al. Fully automated closed-loop insulin delivery vs. semi-automated hybrid control in pediatric patients with type 1 diabetes using an artificial pancreas. Diabetes Care 2008;31:934-9
- Doyle FJ, Huyett LM, Lee JB, et al. Closed-loop artificial pancreas systems: engineering the algorithms. Diabetes Care 2014;37:1191-7
- Phillip M, Battelino T, Atlas E, et al. Nocturnal glucose control with an artificial pancreas at a diabetes camp. N Engl J Med 2013;368:824-33
- Nimri R, Muller I, Atlas E, et al. MD-logic overnight control for 6 weeks of home use in patients with type 1 diabetes: randomized crossover trial. Diabetes Care 2014;37:3025-32
- Thabit H, Lubina-Solomon A, Stadler M, et al. Home use of closed-loop insulin delivery for overnight glucose control in adults with type 1 diabetes: a 4-week, multi-centre, randomised crossover study. Lancet Diabetes Endocrinol 2014;2:701-9
- Kovatchev BP, Renard E, Cobelli C, et al. Safety of outpatient closed-loop control: first randomized crossover trials of a wearable artificial pancreas. Diabetes Care 2014;37:1789-96
- Leelarathna L, Dellweg S, Mader JK, et al. Day and night home closed-loop insulin delivery in adults with type 1 diabetes: three-center randomized crossover study. Diabetes Care 2014;37:1931-7
- Russell SJ, El-Khatib F, Sinha M, et al. Outpatient glycemic control with a bionic pancreas in type 1 diabetes. N Engl J Med 2014;371:313-25
- Weinzimer SA, Sherr JL, Cengiz E, et al. Effect of pramlintide on prandial glycemic excursions during closed-loop control in adolescents and young adults with type 1 diabetes. Diabetes Care 2012;35:1994-9
- Damiano ER, McKeon K, El-Khatib F, et al. A comparative effectiveness analysis of three continuous glucose monitors: the Navigator, G4 Platinum, and Enlite. J Diabetes Sci Technol 2014;8:699-708
- Baysal N, Cameron F, Buckingham BA, et al. A novel method to detect pressure-induced sensor attentuations (PISA) in an artificial pancreas. J Diabetes Sci Technol 2014;8:1091-6