Abstract
The late Holocene history of eolian activity in a parabolic dune complex in the northern Chugach Mountains, Alaska, is reconstructed using 80 tree-ring dates and 5 radiocarbon ages. A radiocarbon age on detrital organics shows mobilization of sands about cal yr A.D. 1260. General forest growth over the dune field area indicates that this active interval was followed by dune stabilization by A.D. 1500. The present interval of dune migration activity, reconstructed from calendar dates and radiocarbon ages on buried trees, began as early as the late A.D. 1600s, was well under way by the mid–A.D. 1700s, and continues today at a diminishing rate. Intervals of increased eolian activity correspond with pulses of the Little Ice Age as reconstructed from a well-established glacial history for the region over the last 1000 yr. Century-scale cooling in climate appears to have forced dune activity through general geomorphic instabilities of nearby glacial and fluvial-lacustrine systems. Geomorphic activity includes a combination of dynamic fluvial incision of cutbanks and release of dormant dune sands, increased wind intensity, as well as internal feedback mechanisms associated with parabolic and blowout dune dynamics. Over the past 200 yr, average rates of dune migration between 1 to 3 m yr−1 are estimated using tree-ring dating. Observations and tree ages along the dune margins suggest that vegetative cover may now be increasing and dune stabilization may be under way.
Background and Purpose
Much effort has focused on studying the glacial-interglacial response of eolian systems to climate variations on multimillennial timescales. However, the response of dune activity to climate changes of decades to centuries is not well documented (CitationKochel and Miller, 1997). Understanding the response of geomorphic systems to climate changes on these timescales is crucial for paleoenvironmental interpretation and for anticipating future response to changing environmental conditions such as contemporary warming.
Decadal and century-scale climate has a role in dune formation and remobilization. Eolian response to Little Ice Age (LIA) cooling is complicated by the role of associated moisture changes (CitationWolfe et al., 1995; CitationLancaster, 1997; CitationForman et al., 2001), fluctuations in the extent of vegetation (Filion, 1984; CitationKäyhkö et al., 1999) and the level of geomorphic activity (CitationEisner et al., 1995; CitationKocurek and Lancaster, 1999; CitationWilson et al., 2001). One of the challenges in identifying the response of geomorphic systems to past climate variations has been the lack of a good chronology of geomorphic activity and a reliable record of regional climate change. In this paper we present a radiocarbon and tree-ring dated history of eolian activity for the past 1000 yr from the Tana dune system located in the northern Chugach Mountains in Alaska . We then compare the reconstructed eolian history with a well-dated glacial chronology for the region (CitationWiles et al., 2002), which is primarily a record of temperature variability during the LIA. We consider the LIA to encompass the interval from about A.D. 1200 to the late A.D. 1800s. This comparison enables identification of factors influencing eolian activity over the past 1000 yr.
Study Area and Dune Description
The Tana dune complex is located along the northern flank of the Chugach Mountains within the Tana River valley . No meteorological data are available for the immediate area, but the climate is continental because the Chugach Mountains partially block the maritime influences from the north Pacific. The nearest meteorological data are from Kennicott and McCarthy, 50 km northwest of the dune, where weather observations have been taken discontinuously since 1922 and show a mean annual precipitation of about 20 cm yr−1 (Rickman and Rosenkrans, 1996). Gulkana, 100 km northwest of McCarthy and Kennicott, has a mean January temperature of −21°C and a mean July temperature of 14°C, and 27 cm of precipitation. The climate of these interior sites is in sharp contrast with the coastal Chugach Mountains, where Cordova, located 150 km southwest of the dunes on the seaward side of the Chugach Range, receives an order of magnitude more precipitation at 244 cm yr−1, with a milder January mean temperature of −4.8°C and a July mean of 12°C.
The steep climate gradient across the northern Chugach–southern Wrangell Mountain region, coupled with the location of the 0°C annual isotherm (Péwé, 1975) creates a climate-sensitive environment for geomorphic systems. The area contains a continuum from relatively debris-free glaciers to almost completely debris-covered glaciers and a set of spectacular rock glaciers as well as the Tana dunes. CitationWiles et al. (2002) present a tree-ring, radiocarbon, and lichenometric glacial history for the region during the late Holocene. This history is a multidecadal to century-scale record of temperature variations for the region, and it is consistent with similar records for southern coastal Alaska that include many glaciers from the Chugach Range (CitationCalkin et al., 2001). Tree-ring temperature reconstructions include data from the northern Wrangell Mountains and show the decadal-to-centennial variations in temperature in the study area that are typical of the interior and Arctic Alaska, which include LIA cooling prior to the strong warming since the mid–A.D. 1800s (CitationJacoby et al., 1999; CitationDavi et al., in revision).
The Tana dune complex is a 1.2-km-long, parabolic form with two parallel ridges of 1- to 2-m-high transverse dunes superimposed on the back of the dune ( and ). The overall parabolic morphology reflects the strong prevailing winds toward the north, northwest along the major axis of the Tana River valley. The strong unidirectional wind flows from the head of the valley at the snout of the Tana Glacier 15 km to the southeast and from within tributary valleys in the surrounding snow and icefields. CitationYehle (2001) mapped the surficial geology of the quadrangles northwest of the dune area, and reported that sparsely vegetated floodplains, like that along the Tana River, often have eolian landforms associated with eroding, unconsolidated, forested bluffs. The Tana dunes fit this description with much of the sediment derived from an eroding bluff and the barren braided floodplain, which then becomes trapped in the forest above. The braided river plain immediately to the southeast of the dunefield has a set of conspicuous beach ridges along its northwestern shore. These beach ridges reveal that the level of the river occupying the braid plain was a lake in the past. Blowouts occur along the southern portion of the dune complex . From comparisons of vertical air photographs taken in 1952 and 1975, and field observations in 1998, the southern extent and areas outside of the dune horns, along with blowout depressions are becoming more vegetated.
The overall stratigraphy of the dune is exposed in the southern blowout area between sites 16 and 17 (, ), and consists of a basal gravel and boulder lag beneath an eolian complex. Dune sediments are subangular, fine to very fine sand, reflecting the proximal, glacially worked, crystalline rocks of the northern Chugach Range. Directly overlying the gravels are the sands of an older eolian dune with an incipient soil in which a ghost forest is rooted (, ). This soil and forest are buried by the sand of the subsequent aggradation by the modern dune. Sand thicknesses for the modern dune area are estimated from the extent of tree burial, and range from a maximum of about 10 m near the leading edge at the crest of the slipface to less than 1 m in the trailing blowout areas (, ).
This paper focuses on the tree-ring and radiocarbon dating of the ghost forest. Trees are being buried or have been killed and are now exposed in the wake of the advancing dune as it migrates into the surrounding, living white spruce forest (Picea glauca [Moench] Voss).
Methods and Results
We sampled multiple cores from subfossil and living trees during the summers of 1998 and 1999 to reconstruct the dune history (CitationMcAllister, 2000). We used standard dendrochronologic techniques (Stokes and Smiley, 1962; Cook and Kariukstis, 1990) to cross-date the subfossil trees making up the ghost forest. A floating ring-width series was first developed from cores and calendar ages were then assigned by cross-dating this floating series with living trees from within and near the leading edge of the dune. The final ring-width chronology consists of 93 series from 80 trees, and compares well with other tree-ring chronologies from the region .
The kill dates (outer ring calendar dates) of these trees are the primary data on which our interpretations are based. Most trees were partially rotted or badly abraded and therefore these dates are considered to be maximum limiting ages since the outer rings are lost. Inner ring dates (pith dates), in most cases, are younger than the germination date of the tree because sampling was done at the present land surface, which may have been meters above the rooted level. Therefore, these dates are minimum limiting dates with germination taking place prior to the pith date. For calculating minimum age estimates for surfaces we add a 30-yr ecesis interval to the tree age. This ecesis estimate of seedling establishment time is taken from glacial studies in the nearby Wrangell Mountains (CitationViereck, 1967; CitationWiles et al., 2002).
Radiocarbon dating was instrumental in determining the initial age range of dune activity. Five radiocarbon ages were calibrated using CALIB 4.3 (CitationStuiver at al., 1998; CitationStuiver et al., 2000), and ages are reported and interpreted in the text using the two sigma range. Ages have been rounded to the nearest decade. Three of the five ages obtained are superseded by the tree-ring dating because they yielded ambiguous calibrated results, including modern ages.
The oldest radiocarbon age is from the lower sand, in which the ghost forest is rooted (site 13; , ). This age was obtained from detrital organics incorporated in the crossbeds of the dune sand. The woody debris was reworked and yielded a calibrated age range of cal yr A.D. 1190 (1260) 1280 based on the measured age of 800 ± 30 yr BP (NSRL-11341).
Above the lower sand and rooted in it is the ghost forest . Calendar pith dates calculated by tree-ring cross-dating and corrected with the ecesis interval of 30 yr show that trees were established on much of the dune area during the early decades of the A.D. 1500s . Inner-ring calendar dates cluster around the early part of the A.D. 1700s from sites toward the leading edge of the dune (sites 1, 2, 3; , ). Tree death dates from the ghost forest show that dune mobilization may have occurred as early as the A.D. 1600s, and was well under way by the mid–A.D.1700s . A radiocarbon age on the outer rings of a log of 210 ± 50 yr BP and calibrated to cal yr A.D. 1530 (1670, 1780, 1790) 1950 (Beta-133800) was obtained from site 17 on wood too poorly preserved for tree-ring dating. Here, in the wake of the migrating dune, is the most southerly occurrence of the ghost forest. Based on the nearby tree-ring dates, the calibrated age of cal yr A.D. 1670 is the most plausible age for this wood.
Rates of dune migration, estimated from outer-ring dates along transects, are considered to represent the migration of the crest. Tree kills show that the dune migrated about 3 m yr−1 from A.D. 1790 through 1960 along a near-dune axis transect at site 8 . An estimate taken from a more restricted transect at site 3 along the slip face shows migration over a 15-m distance between 1970 and 1995. This yields an advance rate of about 1 m yr−1.
In addition to dating trees buried in dune sands, living trees provide estimates of the age of beach ridges and dune stabilization. Trees were sampled from blowout areas along the margins of the dune complex to estimate the timing of stabilization. Trees near site 6 had 35 growth rings and together with an ecesis estimate of 30 yr indicate stabilization here in the A.D. 1930s. At site 7 110 growth rings plus the ecesis estimate show stabilization during the late 1800s. These observations suggest that some areas of the dune complex have stabilized over the past 125 yr.
The beach ridge along the shore of the Tana River is forested . Trees growing on beach ridges were about 20 yr old, suggesting a high lake stand before about 1950. The low gradient of the floodplain can easily cause water to pile up and therefore raise lake levels south of the spillway. The level may also fluctuate seasonally as ice dams form and intervals of high flows become backed up due to the restriction at the spillway . The Tana River may also change its level as periodic floodwaters from ice-dammed lakes impounded by the Tana Glacier ice have backed up at the outlet.
Summary and Comparison with Paleoclimate Records
The recent history of the Tana dune includes two periods of dune activity. The first is centered around A.D. 1260 followed by stabilization and general forest growth by the mid–A.D. 1500s near the back of the dune, to the early A.D. 1700s at the present dune front as reconstructed from calendar pith dates . These pith dates are considered minimum-limiting ages as the true pith dates are probably older depending on the height of the stem that the core was taken.
The second remobilization of dune sands that continues today was initiated as early as the late A.D. 1600s and was well under way by the mid–A.D. 1700s according to calendar dates on outer rings of the ghost forest. These dates are maximum–limiting ages as most trees have experienced a loss of their outer rings. Dune activity persists today as the primary sediment sources from the erosion of the lower sand in the blowout areas and the periodically exposed river bed continue to supply sediment. Rates of dune migration range from 3 m yr−1 for the interval from A.D. 1790 to 1960 to about 1 m yr−1 for recent decades. These values suggest that dune activity has declined over the past 150 yr.
Century-scale changes in climate occurring in the region have been well documented through the study of nearby glacier fluctuations (CitationWiles et al., 2002). Analyses of the tree-ring data also show that the low frequency changes in temperature as reconstructed using tree rings (CitationJacoby et al., 1999; CitationDavi et al., in revision) are similar to those in the dune series. The A.D. 1260 radiocarbon age suggests the lower sand was active during the early LIA, which is dated in the Wrangells and from the Chugach Mountains along the Gulf of Alaska about A.D. 1250. The strongest cooling during the LIA advance is most likely centered on A.D. 1650 (mid-LIA advance) corresponding to reactivation of the dune complex. The final pulse of the LIA centered on A.D. 1850 appears to have sustained the march of the dunes that continues today , although at a slower pace.
Determining the mechanism that forced dune mobilization is complex. Expansion of the Tana Glacier can result from increasing wind intensity with a more extensive snow and ice cover. Cooling can also increase sediment to the system as the Tana Glacier upstream from the dunes becomes more active and the river increases discharge and capacity. The higher flows can lead to increased dune activity when instabilities, such as bank cutting, destabilize the unconsolidated banks of the river releasing the dormant dune sands. Once exposed, a general degradation of the land surface begins, which is self-sustaining as the blowout area provides sand for the active migration of the dune.
In a review, CitationKocurek and Lancaster (1999) emphasize the importance of sediment supply as crucial in determining the presence of dunes. They point out that deflated alluvial and lacustrine systems are often an external source of sediment, whereas internal sources and the role of cannibalism of sediments in blowout areas are crucial in sustaining dune migration. These sources of sand are identified in our discussion of the Tana dune field. The observation by CitationYehle (2001) that links the occurrence of eolian landforms with eroding, unconsolidated bluffs is also consistent with the geomorphic setting of the Tana dunes.
Other studies support some of these suggestions put forth to explain the Tana dune activity. Natural and anthropogenic variations in ecosystems have been implicated in the reactivation and sustained dune migration in a series of classic studies in Finland (CitationAlestalo, 1971, Citation1979; CitationHeikkenen and Tikkanen, 1987; CitationKäyhkö et al., 1999). Recently, CitationKäyhkö et al. (1999) noted that the LIA was a time of active dune migration in subarctic Fennoscandia. The increase in dune activity may have been forced by LIA cooling and changes in vegetation; however, reindeer trampling and forest fires may have also played a role in reactivation. CitationWilson et al. (2001) in a study in northern coastal England noted that dunes were active during times corresponding with the LIA. The connection here between climate and eolian activity was an increase in sediment supply and more vigorous winds forced by the cooler intervals. In contrast to these findings, work in Greenland has suggested that increased eolian activity is linked with intervals of retreating ice and therefore warming, when more sediment is exposed to the wind (CitationEisner et al., 1995).
In North America, Filion (1984) has linked greater dune activity to increased forest fire frequency in the Canadian Arctic resulting in the removal of protective and stabilizing vegetative cover. Although paleoenvironmental data are scant from the Tana dune region, the roles of fire, changes in land use, or ecosystem shifts are considered to be minor.
Dune stability in environments where moisture changes control vegetative cover are important during the LIA. Vance et al. (1992) noted that dunes in the Canadian Prairie were stable during the LIA probably due to associated increases in moisture. In contrast to stabilization during the LIA on the Canadian Prairie, CitationWolfe et al. (1995) examined the dune activity in the Great Sand Hills region of Saskatchewan and suggested that intervals of drought during the LIA and accompanying decreased vegetation led to increased eolian activity. Increased precipitation with warming was associated with dune stabilization here.
In northwest Alaska, CitationHeiser et al. (1999) have implicated increased aridity associated with cooling in remobilization of ancient dune sands of the Great Kobuk Dunefield. Since A.D. 1920 the Kobuk dunes have retracted as precipitation in the region has increased. Precipitation trends clearly will have an effect on the dunes by controlling vegetative cover. Increased precipitation with warm intervals over the past 1000 yr may well have had a role in stabilizing dune activity at the Tana dunes.
Our observations that vegetative cover has increased over portions of the complex parabolic dune and may be limiting sand supply have also been shown to control dune morphology. CitationAnthonsen et al. (1996) analyzed the Råbjerg Mile parabolic dune in Denmark, a dune complex of similar size and morphology to the Tana dune. Over a 100-yr period the dune evolved from a crescentic to parabolic form. This change in form was attributed to a reduction in wind energy and increased vegetative cover. Although the LIA morphology of the Tana dune is unknown, its present parabolic form is consistent with probable trends in wind vigor and vegetative cover.
Conclusions
Identifying the controls on activity at the Tana dunes depends on the climatic response of a series of complex geomorphic systems including glacial, fluvial, and lacustrine activity. These systems have been influenced by a series of century-scale climate variations. The following conclusions are derived for the past 1000 yr of dune activity and its comparison with the proxy climate record from the regional glacier and tree-ring record.
1. The Tana dune history is consistent with the LIA history for the region with cool intervals associated with increased dune activity. The early and mid-LIA activity corresponds well with dune activity and the late LIA sustains the dune migration that was begun during the early A.D. 1700s. Between the early and mid-LIA dune stabilization is evident based on the growth of the ghost forest beginning in the A.D. 1500s. Presently, the dune continues to migrate despite the widely recognized warming during the late 19th through 21st centuries. Some stabilization may be currently under way and the overall parabolic form of the dune is consistent with decreasing wind speed and increased vegetative cover.
2. The controls on dune mobilization appear to be a combination of increased sediment supply and possibly increased winds associated with the LIA. Sediment increases during LIA cooling may be associated with general instabilities in the glacier-fluvial-lacustrine system that supplies sediment to the dunes. The underlying, dormant sands are also a source of sediment as the landscape is reworked in the blowout areas, and this material is mobilized.
FIGURE 1. (a) Air photograph of the Tana dune and location map. Here the parabolic morphology of the dune is evident (USGS 1:80,000; 1981). (b) Map of the dune and sample sites. Screened lines indicate sampling transects.
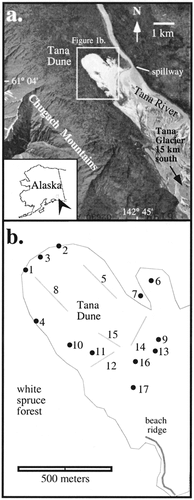
FIGURE 2. Morphology of the complex Tana dune. (a) The overall morphology of the dune advancing into spruce forest. Northwest is to the right. (b) Looking northeast at a ridge of superimposed transverse forms migrating along the larger parabolic feature. (c) The overrun ghost forest is evident in the southern blowout region of the dune field. (d) Close-up of the leading edge of sand migrating into recently buried trees.
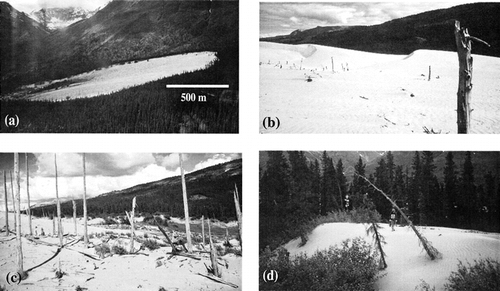
FIGURE 3. Composite stratigraphy of the dune complex. The lower sands are exposed in the southeastern locations and the overall sediment thickness reaches a maximum at the northwest apex of the dune.
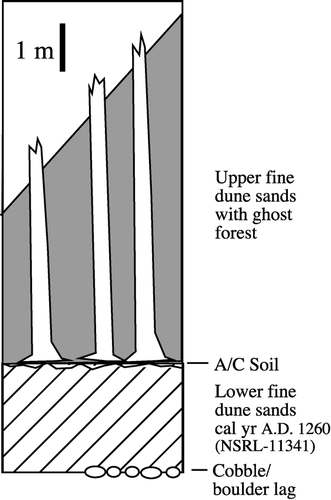
FIGURE 4. (a) The cross-dated positions of 80 trees. Series are arranged from south (bottom) to north (top). See for site locations. Inner ring dates are maxima as the stems of trees were sampled meters above the original ground surface. Outer-ring kill dates are minimal as an undetermined number of rings have been abraded or rotted. The black bars are intervals of growth for trees from the ghost forest; the gray bars are living trees. (b) The composite ring-width series based on samples from the Tana dunes. The broken line is a temperature reconstruction based on tree rings (CitationJacoby et al., 1999). Note the similarity between the temperature reconstruction and the growth patterns of the Tana dune trees.
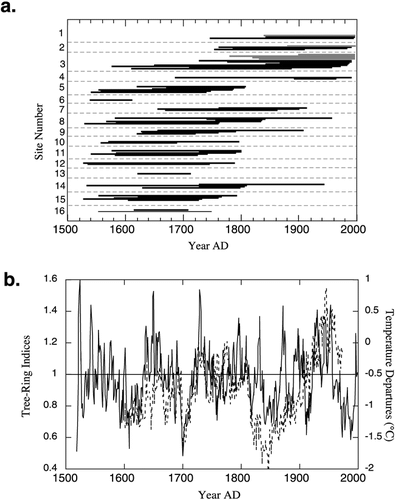
FIGURE 5. Comparison of glacial history from coastal Alaska and the Wrangell Mountains (CitationWiles et al., 1999, Citation2002) with dune activity. The glacier record is considered a proxy for summer temperature. The three-part LIA is evident from this record showing ice advances centered on A.D. 1250, 1650, and 1850 (shaded). The gray bars represent the ranges of radiocarbon-dated ice expansions. Black bars for all three regions are tree-ring-dated forests overrun by ice. Each bar represents an individual tree. The histograms are based on moraine dates. The two sigma range for radiocarbon age of NSRL-11341 from the lower sand at Tana Dunes is also shown.
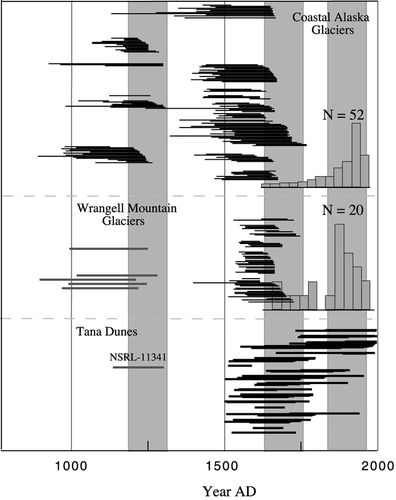
Acknowledgments
Gary Green of McCarthy Air provided logistical support and introduced us to the dune area. Leslie McCluskey helped in tree-ring dating and Sarah Skelly assisted in field sampling. This work was supported by the National Science Foundation Grant ATM97-09095 and ATM99-10805. A Research Experience for Undergraduates NSF supplement grant to R. P. McAllister is also gratefully acknowledged.
References Cited
- Alestalo, J. 1971. Dendrochronological interpretation of geomorphic processes. Fennia 105:140.
- Alestalo, J. 1979. Land uplift and development of littoral and aeolian morphology on Hailuotot, Finland. Acta Universitatis Ouluensis, A 82, Geologica 3:109–120.
- Anthonsen, K. L. , L. B. Clemmensen , and J. H. Jensen . 1996. Evolution of a dune from crescentic to parabolic form in response to short-term climatic changes: Råbjerg Mile, Skegen Odde, Denmark. Geomorphology 17:63–77.
- Calkin, P. E. , G. C. Wiles , and D. J. Barclay . 2001. Holocene coastal glaciation of Alaska. Quaternary Science Reviews 20:449–461.
- Davi, N. , G. C. Jacoby , and G. C. Wiles . in revision. Boreal temperature variability inferred from maximum latewood density and tree-ring width data, Wrangell Mountain Region, Alaska. Climatic Change .
- Eisner, W. R. , T. E. Törnqvist , E. A. Koster , O. Bennike , and J. F. N. van Leeuwen . 1995. Paleoecological studies of a Holocene lacustrine record from the Kangerlussuaq (Søndre Strømfjord) region of West Greenland. Quaternary Research 43:55–66.
- Forman, S. L. , R. Oglesby , and R. S. Webb . 2001. Temporal and spatial patterns of Holocene dune activity on the Great Plains of North America: megadroughts and climate links. Global and Planetary Change 29:1–29.
- Heikkenen, O. and M. Tikkanen . 1987. The Kalajoki dune field on the west coast of Finland. Fennia 165:241–267.
- Heiser, P. A. , B. P. Finney , and D. H. Mann . 1999. Holocene dune activity recorded in high-resolution lacustrine records from Great Kobuk Sand Dunes, Northwest Alaska. Geological Society of America Abstracts with Programs 31:7 53.
- Jacoby, G. C. , R. D. D'Arrigo , and G. Juday . 1999. Tree-ring indicators of climatic change at northern latitudes. World Resources Review 11:21–29.
- Käyhkö, J. A. , P. Worsley , K. Pye , and M. L. Clarke . 1999. A revised chronology for aeolian activity in subarctic Fennoscandia during the Holocene. The Holocene 9:195–205.
- Kochel, R. C. and J. R. Miller . 1997. Geomorphic responses to short-term climatic change: an introduction. Geomorphology 19:171–173.
- Kocurek, G. and N. Lancaster . 1999. Aeolian system sediment state: theory and Mojave Desert Kelso dune field example. Sedimentology 46:505–515.
- Lancaster, N. 1997. Response of eolian geomorphic systems to minor climate change: examples from the southern California deserts. Geomorphology 19:333–347.
- McAllister, R. P. 2000. Dendrogeomorphology and recent history of the Tana Dunes, south-central Alaska. Unpublished undergraduate thesis, The College of Wooster. 81. pp.
- McAllister, R. P. , G. C. Wiles , N. K. Davi , and G. C. Jacoby . 2000. Dendrogeomorphology of the Tana Dunes, Alaska. Geological Society of America Abstracts with Programs 32:4 51.
- Stokes, M. A. and T. L. Smiley . 1968. An Introduction to Tree-Ring Dating. Chicago: University of Chicago Press. 73. pp.
- Stuiver, M. , P. J. Reimer , and T. F. Braziunas . 1998. High-precision radiocarbon age calibration for terrestrial and marine samples. Radiocarbon 40:1127–1151.
- Stuiver, M. , P. J. Reimer , and R. Reimer . 2000. CALIB 4.3 Radiocarbon Calibration. University of Washington: http://depts.washington.edu/qil/calib/calib.htm .
- Viereck, L. 1967. Botanical dating of recent glacial activity in western North America. In Wright, H. E., Jr., and Osburn, W. H., eds., Arctic and Alpine Environments. Bloomington: Indiana University Press. 189–204.
- Wiles, G. C. , D. J. Barclay , and P. E. Calkin . 1999. Tree-ring dated Little Ice Age histories of maritime glaciers from western Prince William Sound, Alaska. The Holocene 9:163–173.
- Wiles, G. C. , G. C. Jacoby , N. K. Davi , and R. P. McAllister . 2002. Late Holocene glacial fluctuations in the Wrangell Mountains, Alaska. Geological Society of America Bulletin 114:896–908.
- Wilson, P. , J. D. Orford , J. Knight , S. M. Braley , and A. C. Wintle . 2001. Late Holocene (post–4000 years BP) coastal dune development in Northumberland, northeast England. The Holocene 11:215–229.
- Wolfe, S. A. , D. J. Huntley , and J. Ollerhead . 1995. Recent and late Holocene sand dune activity in southwestern Saskatchewan. Geological Survey of Canada, Current Research 1995-B:131–140.
- Yehle, L. A. 2001. Preliminary surficial geologic maps of the McCarthy B-6, 7, 8 and C-8 Quadrangles, Alaska. Administrative Report, U.S. Geological Survey, 4 plates.