Abstract
Changes in diatom assemblage composition since preindustrial times were analyzed in a landscape paleolimnological study of 50 lakes and also in a more detailed analysis of a dated sediment core from Slipper Lake in the Canadian central arctic treeline region. The most apparent taxonomic shift was toward a higher relative abundance of the planktonic Cyclotella stelligera complex and a lower relative abundance of benthic Fragilaria taxa (F. pinnata, F. construens var. venter, F. construens, and F. brevistriata) in the modern versus the older sediments. Diatom assemblage composition in Slipper Lake recorded a marked change in the top 5.0 cm (ca. mid-1800s) of the core with a clear shift to a more planktonic diatom assemblage characterized by higher percentages of the Cyclotella stelligera complex. Possible causative factors, such as recent anthropogenic acidification, nutrient enrichment, or atmospheric deposition of contaminants, do not appear sufficient to explain these species changes. Instead, these recent assemblage shifts are consistent with limnological changes occurring with climatic warming, such as a shorter duration of ice cover, a longer growing season, and/or stronger thermal stratification patterns.
Introduction
Arctic and subarctic regions are particularly sensitive to environmental changes, and especially those related to climatic warming (CitationSerreze et al., 2000). Average surface air temperature has increased by ∼0.3°C per decade in the 20th century (CitationKeyser et al., 2000), and increases are predicted to continue to about 4°C in subarctic Canadian regions over the next century (CitationHoughton et al., 1996). Circumpolar treeline regions are especially important for studies of environmental and climatic change, as vegetation growth is closely associated with climatic factors such as temperature (CitationTimoney et al., 1992). Recent increased concern over climatic warming emphasizes the importance of environmental studies in northern treeline regions.
The central Canadian arctic treeline region covers a large geographic area containing a multitude of lakes and ponds, which are further characterized by diverse catchment characteristics. The abundances and diversity of sites pose challenges for regional studies, as sufficiently large numbers of lakes must be studied in order to track changes on landscape scales. As climate change affects hydrological conditions (CitationMacDonald et al., 2000), as well as the physical (e.g., thermocline depth, temperature) and chemical (e.g., ionic concentrations) properties of lakes (CitationRouse et al., 1997), changes in these limnological properties will also influence the composition of aquatic biota (e.g., diatoms: CitationDouglas and Smol, 1999; CitationLotter et al., 1999). Moreover, lakes from different ecozones (e.g., boreal forest, arctic tundra) will likely respond differently to environmental changes.
In order to assess or characterize environmental changes across large regions, long-term water quality data or baseline conditions are required. Unfortunately, almost no long-term monitoring data exist in arctic and subarctic regions. Without an estimate of past environmental conditions, it is not possible to assess the magnitude and direction of recent environmental changes. Paleolimnological methods provide a means to reconstruct past environmental conditions (CitationSmol, 2002).
Diatoms (Bacillariophyceae) are the most extensively used biological indicators in paleolimnological studies (CitationStoermer and Smol, 1999). They are abundant, ecologically diverse, and, due to the siliceous nature of their cell walls, diatom valves are often well preserved in lake sediments. Furthermore, diatoms exhibit a variety of life strategies, have specific ecological preferences, and their short life spans enable them to respond rapidly to environmental changes. Collectively, these characteristics make diatoms effective paleo-indicators of environmental change, and are especially useful in arctic and subarctic regions (CitationDouglas and Smol, 1999; CitationLotter et al., 1999).
Paleolimnological techniques are rapidly being integrated into a variety of studies dealing with ecosystem change, and especially those related to lake management issues (CitationSmol, 2002). One of the major drawbacks of detailed paleolimnological studies, however, is that they are often labor-intensive, and so only a few sites can typically be studied in detail (e.g., at close sediment intervals). For some types of environmental assessments, such detailed analyses, although desirable, are not feasible, and so paleolimnologists have developed sampling methods that allow for relatively quick assessments of environmental change using the so-called top-bottom approaches. Indicators (e.g., diatoms) present in the surficial sediments of the lakes (e.g., 0–0.5 cm, the so-called top sample) are assumed to represent present-day or recent conditions, whereas the diatoms preserved in sediments deposited from preindustrial times (e.g., pre-1850 for most regions of Canada; the so-called bottom sample) represent “natural” or “baseline” conditions (CitationDixit et al., 1999). Sediment samples have the advantage that they represent an integrated sample of diatoms from all habitats within the lake, as well as an integration of several years of sediment accumulation. This “top-bottom” approach allows researchers to investigate large numbers of lakes over broad geographic areas, thereby making regional environmental assessments possible. However, this technique also has some limitations. As comparisons are only made between two discrete time slices (i.e., top versus bottom), it is not possible to precisely pinpoint the timing of environmental changes. Rather, the goal of this type of study is to determine, on a regional scale, whether the present-day environmental conditions are similar to those during preindustrial times, and if not, what changes have occurred.
Although the “top-bottom” approach has been used in a variety of lake management studies (e.g., acid rain: CitationCumming et al., 1992; CitationSmol et al., 1998; CitationKorsman, 1999; eutrophication: CitationHall and Smol, 1996; CitationDixit et al., 1999), it has rarely been applied to arctic or subarctic regions (e.g., CitationKorsman, 1999; CitationMichelutti et al., 2000), and has yet to be used for a paleoclimatic assessment. In this study, we apply this “top-bottom” approach to make a regional assessment of environmental changes by comparing preindustrial to modern diatom assemblages from 50 lakes spanning the northern boreal forest treeline and the tundra ecozones in the central Canadian Subarctic. Our data show that the most prominent regional shifts in diatom assemblages were related to changes in aquatic habitats, most likely as a result of late-19th- and 20th-century climatic warming.
Site Description
The 50 study lakes were selected to cover a large geographic area in the central Canadian arctic treeline region . The transect of lakes (all unnamed, and so referred to as TK-6 to TK-58 with the exception of Slipper Lake [SL]) spans a portion of Canada's circumpolar treeline from the northeastern side of Yellowknife (62°32.72′N, 114°07.13′W) to the northern proximity of the Thelon Game Sanctuary (66°41.32′N, 104°55.86′W) (, ). The region is characterized by gradually changing vegetation and climate, including the boreal forest ecozone to the south, the forest-tundra transitional ecotone, and the arctic tundra ecozone to the north . Vegetation is influenced by many factors, including climate, soils, the degree of permafrost development, the extent of snow accumulation, bedrock geology, and nutrient levels (CitationRitchie, 1993). The study area is underlain by Precambrian Shield bedrock consisting of gneiss, migmatite, granitoid intrusions, and metamorphosed volcanic and sedimentary rocks (CitationPadgham and Fyson, 1992). Soils are poorly developed due to late deglaciation of the region and the presence of underlying permafrost. Permafrost influences soil conditions and is loosely associated with climatic factors such as air temperature, precipitation, and vegetation (CitationBonan, 1992). Consequently, the southern limit of continuous permafrost generally follows the position of arctic treeline, as it has restricting effects on vegetation (CitationMacDonald and Gajewski, 1992).
Warmer seasonal temperatures and higher annual precipitation occur in the boreal forest sites to the south in comparison to the more northern sites in the arctic tundra. The boreal forest region near Yellowknife has an average annual precipitation of 267 mm (CitationCanada, 1990), and mean July daytime and January nighttime temperatures of 22.5°C and −30°C, respectively (CitationCanada, 1991), and lakes have an ice-free period of approximately 120 d (CitationWedel et al., 1990). In contrast, northern sites in the tundra have an ice-free period of only ∼90 d, an average annual precipitation of 200 mm, and mean July daytime and January nighttime temperatures of 12.5°C and −37.5°C, respectively (CitationCanada, 1990; CitationCanada, 1991).
The three ecozones (i.e., boreal forest, forest-tundra, arctic tundra; ) were delineated using aerial photographs, topographical maps, field observations, and maps developed by satellite imagery (CitationCanada, 1996). The zonations closely adhered to descriptions given by CitationRitchie (1993) and CitationTimoney et al. (1993).
The boreal forest is primarily composed of a closed-crown, continuous forest with a lichen floor. Conifers, including Picea mariana (black spruce), P. glauca (white spruce), Abies balsamea (balsam fir), and Pinus banksiana (jackpine), represent the majority of the trees (CitationRitchie, 1993). Conifers also dominate the transition zone (e.g., Picea mariana, P. glauca, and Larix laricina [larch] [CitationSirois, 1992]), but several deciduous tree species (e.g., Populus balsamifera [balsam poplar], P. tremuloides [trembling aspen], and Betula papyrifera [paper birch]) are also present. The arctic tundra ecozone is characterized mainly by sedges, lichen-heath, and dwarf shrub vegetation (CitationRitchie, 1993). A more detailed site description is given in CitationRühland and Smol (2002).
Materials and Methods
COLLECTION OF SEDIMENT CORES
TK-6 to TK-36 were sampled within a short time window between 10 August and 19 August 1996, and TK-37 to TK-58 were sampled between 14 August and 23 August 1998. These 49 sites represent a subset of 77 calibration lakes that have been used to develop diatom-based transfer functions for limnological variables (CitationRühland and Smol, 2002). Slipper Lake (SL), a tundra site, was sampled through ∼1.8 m of ice on 26 March 1997, and was added to this lake set for a total of 50 sites (15 tundra, 11 transition, and 24 forest lakes).
Sediment cores were obtained from the deepest part of each of the 50 lakes using a mini-CitationGlew (1991) gravity corer equipped with a 4.0-cm-diameter core tube. The surficial sediments (top 0.5 cm) and bottom sediments (bottom 1.0 cm) of each core were sectioned using a CitationGlew (1988) vertical extruder. The mean core length for these 50 lakes was 19.4 cm .
The Slipper Lake core was retrieved from the deepest part of the lake (∼14.0 m) using a modified KB gravity corer (10 cm diameter). The 45.5-cm core was extruded on site at 0.5-cm intervals for the top 6.5 cm and at 1.0-cm intervals for the remaining intervals, resulting in 48 sediment intervals.
PREPARATION AND ANALYSES OF SEDIMENT SAMPLES
Sediment samples were prepared for diatom taxonomic identification using standard procedures, as described in CitationRühland and Smol (2002). For the 50-lake study, a minimum of 350 diatom valves along transects of the coverslip were enumerated and identified to the lowest taxonomic level possible. Diatoms in the bottom sediment samples were enumerated using a Nikon Optiphot microscope (100× oil immersion, numerical aperture [N.A.] = 1.25) or a Leica DMRB microscope (100× oil immersion; N.A = 1.3). Diatom taxa in the surficial sediment samples were identified and enumerated using a Leitz Dialux 20 microscope (100× oil immersion; N.A = 0.90). Photomicrographs obtained using the Leica DMRB light microscope equipped with a Wild Photoautomat MPS45 camera system aided in maintaining taxonomic consistency between researchers and in taxonomic identification. Taxonomic consistency between researchers was further evaluated by exchanges of slides and frequent taxonomic checks between researchers during the enumeration process. For the Slipper Lake core, a minimum of 400 diatom valves were identified and enumerated for each sample using a Leitz Dialux 20 microscope (100× oil immersion lens; N.A. 0.90). Diatom enumerations were expressed as percent relative abundances of the total number of diatom valves counted in each sample. Taxonomic references included mainly CitationHustedt (1927–1966), CitationPatrick and Reimer (1966, Citation1975), CitationFoged (1981), CitationKrammer and Lange-Bertalot (1986–1991), CitationCamburn and Charles (2000), CitationHein (1990), CitationCumming et al. (1995) and CitationLange-Bertalot and Metzeltin (1996).
STATISTICAL ANALYSES USED TO INFER PAST ENVIRONMENTAL CONDITIONS
Diatom taxa that occurred with a relative abundance of at least 1% in one lake and were present in at least three lakes formed the data set (i.e., a total of 124 taxa) used for statistical analyses for the “top-bottom” study. Following CitationLaird et al. (1998), Bray-Curtis dissimilarity coefficients (DCs) were used to determine a critical value for comparing the fossil (bottom) diatom assemblages. This was done by comparing each top sample with all other top samples to determine the sample to which it had a minimum DC (i.e., most similar). The 95 percentile for the 50 modern (top) samples was calculated based on the mean minimum DC distance from the modern samples. Any fossil (bottom) sample that had a minimum DC above the 95 percentile (> 0.49) was considered to have no analogs in the modern assemblage (i.e., greater difference).
To identify which diatom taxa were important in determining the differences between groups, we used the PRIMER program SIMPER (CitationClarke and Warwick, 1994). This method computes the average dissimilarity between groups and then assesses the separate contributions of each species to this dissimilarity (CitationClarke and Warwick, 1994).
SLIPPER LAKE SEDIMENT CORE CHRONOLOGY
For 210Pb analysis, dried sediment for selected intervals of the Slipper Lake core were submitted to MyCore Scientific Inc., Deep River, Ontario, for analyses. 210Pb activity for each sample was estimated by alpha spectroscopy. Unsupported 210Pb was calculated by subtracting supported 210Pb from total activity at each measured level, following the guidelines in CitationBinford (1990). Dates were determined from unsupported isotopes using the constant rate of supply (C.R.S.) model (CitationAppleby and Oldfield, 1978).
Accelerator Mass Spectrometry (AMS) dating techniques were used to radiocarbon date bulk sediments (no terrestrial macrofossils were found) from two intervals by IsoTrace Radiocarbon Laboratory (University of Toronto). The radiocarbon dates were calibrated to calendar years before present (cal yr BP) at IsoTrace using the standard data set INTCAL98 (CitationStuiver et al., 1998).
Results
RATIONALE FOR THE “TOP-BOTTOM” APPROACH
We are confident that the downcore diatom assemblages (i.e., bottom samples) represent preindustrial conditions (i.e., conditions prior to ∼1850 A.D.), as full sediment cores from several of our study lakes have been dated using 210Pb chronology. For example, 210Pb dating techniques using alpha spectroscopy show that the entire inventory of unsupported 210Pb was contained in the top 4–5 cm of the Slipper Lake (SL) core, the top 5 cm of the TK-20 core, and the top 6 cm of the TK-54 core (CitationRühland, 2001). Sedimentation rates in these arctic regions are typically very low. Bottom samples in this study ranged in depth from 8.0 cm to 34.0 cm . These data suggest that most, if not all, of our bottom sample assemblages represented preindustrial conditions.
CHANGES IN DIATOM ASSEMBLAGE COMPOSITION SINCE PREINDUSTRIAL TIMES
A total of 410 diatom taxa were identified from the modern and downcore sediment samples. The relative percent abundances of the most common diatom taxa are shown in . Approximately 35% of sites exhibited Bray-Curtis DCs outside the extreme 5% of the modern samples (DC > 0.49) based on diatom assemblage composition. However, no substantial differences were observed in these DCs among the three ecozones (mean DC tundra = 0.46; mean DC forest-tundra = 0.48, mean DC boreal forest = 0.44).
Based on SIMPER results , diatom assemblages in the arctic tundra lakes had higher abundances of the Cyclotella stelligera complex (C. stelligera and C. pseudostelligera) in the modern samples and higher abundances of Fragilaria virescens var. exigua and Cymbella gaeumannii in the bottom samples. Forest-tundra lakes had higher abundances of the Cyclotella stelligera complex in the modern sediments and a higher abundance of Fragilaria virescens var. exigua in the preindustrial sediments. Boreal forest lakes had higher abundances of the Cyclotella stelligera complex in the modern sediments and higher abundances of Fragilaria pinnata and Nitzschia fonticola in the preindustrial sediments. A comparison of all 50 top samples with all 50 bottom samples (i.e., no zonations) revealed that the Cyclotella stelligera complex had higher abundances in the top samples, and that Fragilaria pinnata and F. virescens var. exigua had higher abundances in the bottom samples .
Clear assemblage changes were recorded among the more common diatom taxa since preindustrial times . In agreement with our SIMPER results, the most noted change was an increase in the relative abundances of the Cyclotella stelligera complex and a decrease in the relative abundances of small, benthic Fragilaria taxa (F. pinnata, F. brevistriata, F. construens, and F. construens var. venter) ( and ). Also noteworthy were changes in the relative abundances of Fragilaria virescens var. exigua, Nitzschia fonticola, and Brachysira taxa (B. vitrea, B. brebissonii, B. procera, B. styriaca, and B. zellensis) between top and bottom samples (, and ). These diatoms (particularly the Cyclotella stelligera complex and the small Fragilaria taxa) were commonly abundant in the 50 study lakes and, because they exhibited the most substantial changes, we examined their trends further.
Both the Cyclotella stelligera complex and the small Fragilaria taxa had a higher percent change in sites south of treeline, with Fragilaria species showing an overall decrease in relative abundance in the modern assemblages and Cyclotella species showing an overall increase in abundance . A comparison of the amount of change in these taxa along a gradient of increasing dissolved inorganic carbon (DIC) revealed that lakes higher in alkalinity had greater changes in the percent abundances of Fragilaria and, to a lesser degree, Cyclotella taxa . Deeper lakes recorded greater increases in the amount of change for the Cyclotella species, with the largest changes occurring in lakes with a maximum depth greater than 6.0 m . Lake water depth had no apparent affect on shifts in the relative percentage of small, benthic Fragilaria taxa .
Acidophilic Fragilaria virescens var. exigua valves were common in many of the lakes and, similar to small, alkaliphilic Fragilaria taxa, showed an overall decrease in relative abundance from preindustrial to present time . F. virescens var. exigua was clearly distinct from the remaining benthic Fragilaria taxa and occurred more commonly and in higher relative abundances in lakes low in DIC, but was scarce in lakes with DIC concentrations above 3.5 mg L−1 (CitationRühland et al., in press). Interestingly, F. virescens var. exigua attained highest abundances in lakes that displayed the most change (i.e., highest minimum DC) and, unlike small alkaliphilic Fragilaria taxa, were common in both the arctic tundra and the forest-tundra zones and were scarce in the boreal forest lakes . Nitzschia fonticola and Brachysira vitrea occurred almost exclusively in the boreal forest zone . However, B. vitrea occurred more frequently and in higher abundances in the modern sediments , whereas N. fonticola was more common in preindustrial sediments . Achnanthes taxa were amongst the most commonly occurring diatoms and were present in all 50 lakes in both the top and bottom sediments , with relatively little change between top and bottom samples in most lakes ( and ).
SLIPPER LAKE SEDIMENT CORE
The Slipper Lake 210Pb profile (CitationRühland, 2001) exhibited a characteristic exponential decline in 210Pb concentration from the surface to the bottom sediments. The profile exhibited no major departures from an exponential decay curve, suggesting that sediment mixing was not a problem. The 210Pb activity profile indicated that the entire unsupported 210Pb inventory was contained in the upper 4.0 to 4.5 cm (ca A.D. 1850). Radiocarbon dates indicated that the Slipper Lake core extends back to approximately 3660–3365 calibrated years B.C. .
The diatom flora of Slipper Lake was dominated by small benthic Fragilaria (F. pinnata, F. construens, F. construens var. venter, F. brevistriata) species, as well as by centric, planktonic taxa including the Cyclotella stelligera complex and the heavily silicified Aulacoseira taxa (A. lirata, A. lirata var. biseriata, A. perglabra, A. perglabra var. floriniae) at various intervals throughout the core . Diatom assemblage composition remained relatively stable throughout the bottom core intervals. The benthic Fragilaria complex, previously dominant in this lake, decreases dramatically at ∼9.0 cm (estimated A.D. 1687). Concurrent with decreases in the Aulacoseira taxa, the planktonic Cyclotella stelligera complex first appears ∼8.0 cm and then increases at 5.0 cm (∼A.D. 1837), reaching its maximum abundance (∼20% relative abundance) at the top interval of the core .
Discussion
Our 50 study lakes, which span a diverse array of environments from the treeline ecozone in the central Canadian Arctic, have clearly undergone major changes in diatom communities since preindustrial times, with a general shift toward a higher relative abundance of the planktonic C. stelligera complex and a decrease in the relative abundance of benthic Fragilaria species (, , ). These floristic changes likely occurred during a period of potential anthropogenic disturbance, beginning in the 19th century. Therefore, we explore several hypotheses that could potentially explain these changes, including (1) acidification, (2) nutrient increases, (3) chemical contaminants, and (4) climatic change.
One possible explanation for the recent diatom changes is acidification due to acid precipitation. It is widely accepted that acidification is an important stressor in some poorly buffered systems and that diatoms are excellent indicators of lakewater pH (CitationBattarbee et al., 1999). However, in relatively isolated regions, such as in our study area, atmospheric transport and deposition of sulphuric and nitric acids are very low, even though their concentrations have increased since the mid-1900s (CitationYalcin and Wake, 2001). Furthermore, our study lakes are presently slightly alkaline, well buffered (mean pH of 7.2; ). Moreover, as has been shown in many paleo-acidification studies, a characteristic feature of many acidified lakes is the loss of Cyclotella species (CitationBattarbee et al., 1999), contrasting with the trends that we record in this study. The overall decrease in the abundance of acidophilic Fragilaria virescens var. exigua in the modern sediments also conflicts with the hypothesis that acidification was responsible for these diatom changes. These conclusions are supported by our environmental reconstruction (CitationRühland, 2001), based on modern diatom distributions along a DIC gradient, that showed no consistent changes between modern and preindustrial DIC estimates.
Lakes in remote areas could possibly be affected by atmospheric deposits of nutrients from anthropogenic sources (CitationWolfe et al., 2001). However, based on our modern environmental data, together with our temporal diatom trends, it was clear that there was no relationship between abundances of our most common taxa (Fragilaria and Cyclotella species) and nutrients (total phosphorus [TP] and total nitrogen [TN]) in both the modern assemblages and in the amount of change between the modern and fossil assemblages (r 2 between 0.003 and 0.07). In fact, the modern distribution of Cyclotella species along the TP and TN gradients revealed that higher species abundances were recorded in lakes with low nutrients (i.e., oligotrophic). Furthermore, the majority of our lakes were ultraoligotrophic to oligotrophic (TP <10 µg L−1). Collectively, these findings strongly suggest that the diatom changes we observed over the past two hundred years were not related to human-induced nutrient increases.
Recently, there has been concern over the impact that long-range transport of heavy metals and of persistent organic pollutants (POPs), such as organochlorines and polycyclic aromatic hydrocarbons, have on arctic environments (CitationMuir et al., 1996; CitationDoubleday, 1997; CitationBetts-Piper, 2001; CitationRawn et al., 2001). Production of many of these compounds commenced in the early to mid-1900s and therefore may be a possible explanation for our observed diatom changes. Although paleolimnological studies examining the relationships between arctic diatoms and chrysophytes and contaminants are rare (e.g., CitationBetts-Piper, 2001; CitationMichelutti et al., in press; CitationPaterson et al., in press), these studies have shown that POPs such as polychlorinated biphenyls (PCBs), even in high concentrations (CitationPaterson et al., in press), have had no noticeable impact on arctic algal assemblages over the past ∼200 yr.
Our data make a strong case for dismissing acidification, nutrient enrichment and contaminants as possible causes for the diatom changes observed in this study. The remaining hypothesis that we examined to explain these floristic changes is climatic change. Climatic warming may affect diatom assemblage composition through a longer growing season and extended ice-free periods that affect lakewater characteristics, habitat availability, and, ultimately, aquatic biota (e.g., CitationSmol, 1983, Citation1988; CitationPsenner and Schmidt, 1992; CitationDouglas and Smol, 1999, Citation2000; CitationOhlendorf et al., 2000; CitationCatalan et al. 2002a, Citationb; CitationKoinig et al., 2002; CitationKorhola et al., 2002; CitationLotter et al., 2002). For example, increased duration and stability of thermal stratification with climatic warming alters various lakewater properties, such as nutrient and light availability and pH (e.g., CitationSorvari and Korhola, 1998; CitationLotter and Bigler, 2000; CitationOhlendorf et al., 2000; CitationSorvari et al., 2002). With warming, these limnological changes would generally favor the growth of planktonic diatom species, such as the C. stelligera complex, in arctic environments (CitationSmol, 1988; CitationBattarbee et al., 2002; CitationSorvari et al., 2002).
The changes observed in our 50-lake top-bottom study are similar to the marked diatom changes reported in the High Arctic (CitationDouglas et al., 1994), in Finnish Lapland (CitationSorvari and Korhola, 1998; CitationRautio et al., 2000; CitationSorvari et al., 2002), and in recent studies of several remote European alpine lakes (CitationLotter and Bigler, 2000; CitationCatalan et al., 2002a,Citationb; CitationKoinig et al., 2002; CitationKorhola et al., 2002; CitationLotter et al., 2002; see CitationBattarbee et al., 2002, for overview). These studies have also suggested that the present-day distribution of planktonic Cyclotella stelligera diatoms appear to be induced by warmer conditions during the later part of the thermal stratification period (before fall turnover). In general, planktonic diatoms and air temperature were found to be related to the duration of ice cover, as first considered by CitationSmol (1983, Citation1988). In agreement with our findings, these new studies have consistently found increases in planktonic Cyclotella taxa in the recent sediments of lakes that have been associated with a warming climate, and related limnological changes (e.g., extended and/or more stable thermal stratification, nutrient and light availability, etc.).
CitationSorvari and Korhola (1998) and CitationSorvari et al. (2002) recorded strikingly similar diatom assemblage changes in several Finnish Lapland lakes. They found consistent and synchronous increases in small planktonic Cyclotella species and decreases in benthic diatom taxa in the upper sediments of thermally stratified lakes. Again, these floristic changes were attributed to longer ice-free periods and increased stability of thermal stratification. Similarly, in our set of 50 lakes, deeper sites recorded stronger thermal stratification and also recorded the largest increases in Cyclotella species . CitationCremer et al. (2001) have also found a diatom assemblage change from planktonic Cyclotella pseudostelligera to benthic Fragilaria taxa in an East Greenland lake. They too suggested that this floristic change was likely due to a change in the degree of ice cover related to climatic warming. In our study, the overall changes in benthic (Fragilaria spp.) to planktonic (Cyclotella spp.) diatoms agree with these other paleolimnological studies in that the potential mechanism for this floristic change may be attributed to climatically induced changes over the past ∼150 yr.
In sites dominated by benthic diatom taxa, CitationCameron et al. (2002) and CitationSorvari et al. (2002) recorded much smaller diatom changes over the last few hundred years. CitationSorvari et al. (2002) suggested that the absence of planktonic diatoms in their shallow, isothermal lakes elicited a more muted response to climate change, whereas CitationCameron et al. (2002) suggested the lack of change recorded in their lake may be due to its higher acidity. Similarly, in our study, shallow lakes generally did not record marked changes in species distributions.
A higher relative abundance of Fragilaria species in our bottom sediments can be interpreted as generally colder conditions, and related limnological changes (e.g., more extensive ice and snow cover). Several paleolimnological studies from High Arctic regions have recorded marked declines in these taxa in post-1850 sediments, as warming was believed to have occurred (CitationDouglas et al., 1994; CitationWolfe, 2000; CitationSmith, 2002). For example, CitationSmol (1988) and CitationSmith (2002) observed that small Fragilaria species were dominant in the Arctic during colder temperatures where environmental conditions, such as longer seasonal ice cover, would provide a more favorable environment for the survival of these benthic aquatic diatoms. These observations are consistent with our interpretations of temporal and regional trends in Fragilaria species observed in our study.
Further support for climate-related mechanisms influencing our species distributions were provided by CitationLotter and Bigler's (2000) detailed study of diatoms from a Swiss alpine lake, where benthic Fragilaria taxa were more abundant under ice cover and in sediment deposited during the Little Ice Age cooling interval. Conversely, planktonic diatom communities quickly sank to the lake bottom following ice cover as conditions became less favorable for their growth, confirming CitationSmol's (1988) hypothesis linking diatom life strategies and lake ice cover in the High Arctic.
Detailed diatom analyses of a sediment core from Slipper Lake (SL) corroborate our landscape temporal trends recorded in the top-bottom study. Diatom assemblage composition had been relatively stable during the earlier history of the Slipper Lake core, with the clearest changes occurring during the 19th century , approximately coinciding with post–“Little Ice Age warming” ca. A.D. 1850 (CitationOverpeck et al., 1997; CitationBradley, 2000; CitationGrove, 2001). The C. stelligera complex became a prominent component of the assemblage by the mid-1800s; this expansion predates the period of known nutrient and contaminant increases by about a century (ca. post-1950s). Another notable change occurred at approximately 13.5 cm (estimated early A.D. 1500s) when small, benthic Fragilaria taxa (dominant in the previous sediment levels) diminish to trace levels (i.e., <1% relative abundance) . This clear decrease in alkaliphilic taxa (ca. A.D. 1500) again predates the impact of anthropogenic disturbances. These diatom changes are consistent with a change in climate and consequent changes in the aquatic habitat, supporting the changes observed in our 50-lake top-bottom study.
Summary and Conclusions
Increases in planktonic Cyclotella taxa since preindustrial (ca. A.D. 1850) times in lakes from the Canadian central arctic region are likely due to climatically induced limnological changes associated with a longer ice-free period and/or enhanced thermal stratification. These results have important implications for future climate scenarios, as continued warming will result in more dramatic changes in these sensitive arctic environments. These data suggest that other anthropogenically induced stressors (e.g., acid rain, nutrient enrichment, persistent organic pollutants) are likely not the primary mechanisms influencing these diatom species changes. However, the potential for synergistic effects may be heightened, as these lakes are already undergoing marked environmental changes. Our results show that the application of a paleolimnological lake-management tool (i.e., the “top-bottom” approach) can be effectively used in an arctic treeline environment to test hypotheses and to track environmental changes on a regional scale. In the absence of long-term lake monitoring data, this paleolimnological approach should be considered for other arctic regions.
FIGURE 1. Location of the 50 study lakes and their geographical distribution with respect to the position of arctic treeline.
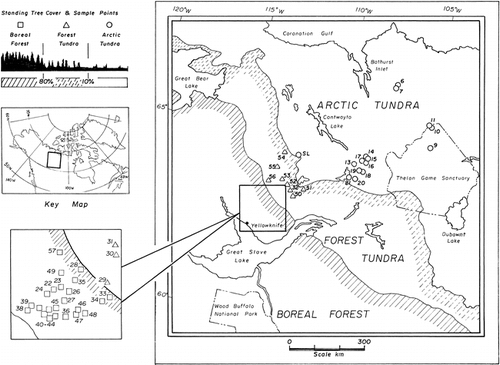
FIGURE 2. Comparison between the relative percent abundances of the most common diatom taxa in modern and preindustrial sediments for the 50 study lakes. Modern diatom abundances are represented by solid bars and fossil abundances by open bars. The lakes are separated into the three ecozones (arctic tundra, forest-tundra, boreal forest). Within each ecozone the lakes are organized in order of decreasing dissimilarity between surface and bottom diatom assemblages, as measured by the minimum Bray-Curtis Dissimilarity Coefficients (Min. DC). SL = Slipper Lake.
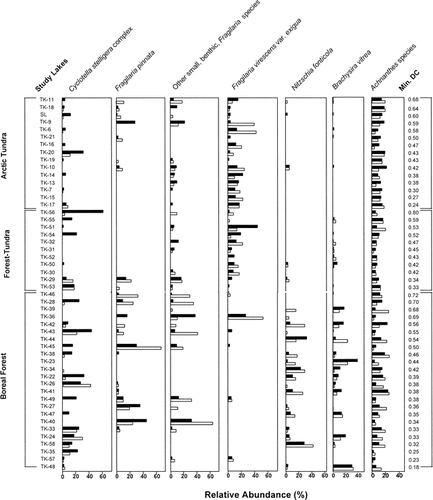
FIGURE 3. The relationship between the percent relative abundances of (a) the Cyclotella stelligera complex (C. stelligera and C. pseudostelligera, (b) small, benthic Fragilaria taxa (F. brevistriata, F. construens, F. construens var. venter, and F. pinnata), (c) Fragilaria virescens var. exigua, (d) Nitzschia fonticola, (e) Brachysira vitrea, and (f) Achnanthes taxa (A. acares, A. altaica, A. carrissima, A. chlidanos, A. conspicua, A. curtissima, A. didyma, A. exigua, A. helvetica, A. impexiformis, A. lacus-vulcani, A. laevissima, A. laterostrata, A. marginulata, A. minutissima, A. pusilla, A. rosenstockii, A. rossii, A. saccula, A. scotica, A. subatomoides, A. suchlandtii, A. thermalis, A. ventralis) found in preindustrial and modern sediments for the 50 study lakes. The majority of lakes lie above the 1:1 line indicating a higher relative abundance of the C. stelligera complex in surface sediments (a). The majority of lakes lie below the 1:1 line indicating a higher relative abundance of small, benthic Fragilaria species in the preindustrial diatom assemblages (b).
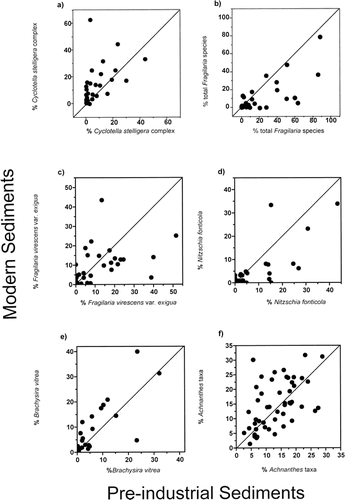
FIGURE 4. The percentage of change between the modern and preindustrial diatom taxa in relation to the distance of each lake from current treeline for (a) the Cyclotella stelligera complex (C. stelligera and C. pseudostelligera) and (b) small, benthic Fragilaria species (F. brevistriata, F. construens, F. construens var. venter, and F. pinnata). The categorization of zones is based largely on vegetational distributions and closely follow schemes by CitationTimoney et al. (1993) and CitationRitchie (1993). These ecozones were delineated according to “percentage tree cover” derived from field observations, topographic maps, aerial photographs, and maps derived from satellite imagery (CitationCanada, 1996). Negative distances along the y-axis indicate lakes south of treeline and positive distances indicate lakes north of treeline. Sites north of treeline showed little change in the abundances of these two important diatom groups whereas sites south of treeline recorded marked changes. Negative results indicate a decrease in the relative percent abundance in the modern sediments and a positive result indicates an increase in the relative percent abundance in the modern sediments. Most lakes south of treeline recorded an increase in the Cyclotella stelligera complex and a decrease in benthic Fragilaria species.
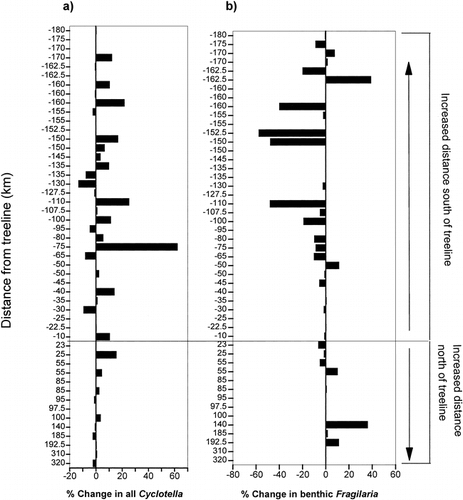
FIGURE 5. The percentage of change between modern and preindustrial diatom taxa in relation to increasing measured dissolved inorganic carbon (DIC) for (a) the Cyclotella stelligera complex (C. stelligera and C. pseudostelligera) and (b) small, benthic Fragilaria species (F. brevistriata, F. construens, F. construens var. venter, and F. pinnata). Negative results indicate a decrease in the relative percent abundance of these diatoms in the modern sediments.
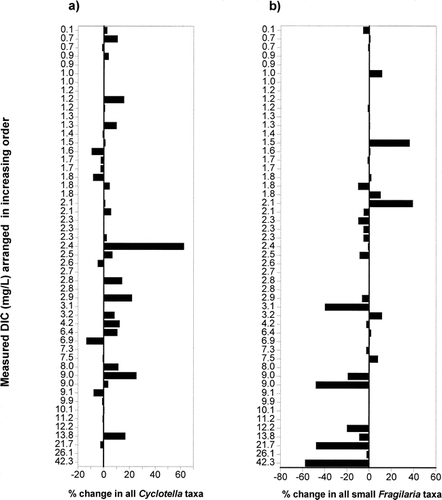
FIGURE 6. The percentage of change between modern and preindustrial diatom taxa in relation to maximum lake depth for (a) the Cyclotella stelligera complex (C. stelligera and C. pseudostelligera) and (b) small, benthic Fragilaria species (F. brevistriata, F. construens, F. construens var. venter, and F. pinnata). Negative results indicate a decrease in the relative percent abundance of these diatoms in the modern sediments.
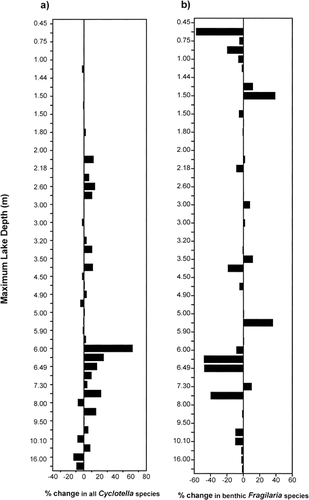
FIGURE 7. Simplified diatom stratigraphy of the three most common and abundant diatom groups from Slipper Lake showing the increase in the relative abundance of the Cyclotella stelligera complex in the recent sediments (modified from CitationRühland, 2001). The radiocarbon dates were calibrated to calendar years using the standard data set INTCAL98 (CitationStuiver et al. 1998). The 2σ range (95% confidence interval) for each age is reported.
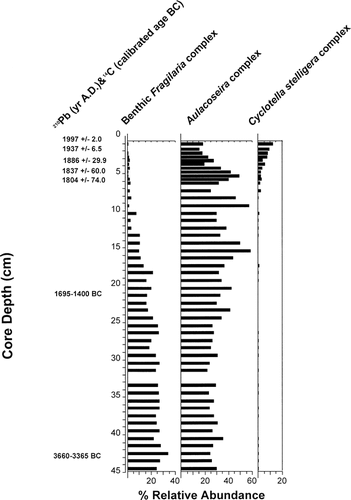
TABLE 1 Geographical coordinates and selected limnological measurements for the 50 study lakesa
TABLE 2 Results of SIMPER test identifying diatom taxa that were important in determining the differences between modern (top) and preindustrial (bottom) sediments in the three ecozones based on their average abundances. The average dissimilarity between the tops and bottoms for each zone is based on percentages (the higher the percentage, the higher the dissimilarity).
Acknowledgments
This research has been supported by an NSERC grant to J. Smol, a Northern Studies Training Program (NSTP) grant, as well as an NSERC special collaborative grant to Paleoecological Analysis of Circumpolar Treeline (PACT). Logistical support from the Polar Continental Shelf Project (PCSP) is also gratefully acknowledged (PCSP contribution # PCSP 01202.). We thank the Department of Indian Affairs and Northern Development (DIAND) in Yellowknife, who invited us to undertake the Slipper Lake research and who retrieved the sediment core and provided the water chemistry (particularly Brian Latham, Glen Stephens, and Francis Jackson). We thank Tammy Karst, Tamsin Laing, Brent Wolfe, and Dave Porinchu for assistance in sediment sampling. Water chemistry analyses for the 50-lake study were facilitated by Derek Muir, National Water Research Institute. We thank John Glew for drafting our map and members of PEARL for additional discussions. We also thank A. P. Wolfe and an anonymous reviewer for greatly improving the clarity and quality of the manuscript.
References Cited
- Appleby, P. G. and F. Oldfield . 1978. The calculation of lead-210 dates assuming a constant rate of supply of unsupported 210Pb to the sediment. Catena 5:1–8.
- Battarbee, R. W. , D. F. Charles , S. S. Dixit , and I. Renberg . 1999. Diatoms as indicators of surface water acidity. In Stoermer, E.F., and Smol, J.P. (eds.), The Diatoms: Applications for the Environmental and Earth Sciences. Cambridge: Cambridge University Press. 85–127.
- Battarbee, R. W. , J-A. Grytnes , R. Thompson , P. G. Appleby , J. Catalan , A. Korhola , H. J. B. Birks , E. Heegaard , and A. Lami . 2002. Comparing palaeolimnological and instrumental evidence of climate change for remote mountain lakes over the last 200 years. Journal of Paleolimnology 28:161–179.
- Betts-Piper, A. A. 2001. Chrysophyte stomatocyst-based paleolimnological investigations of environmental changes in Arctic and alpine environments. M.Sc. thesis, Queen's University, Kingston, Ontario, Canada. 207. pp.
- Binford, M. W. 1990. Calculation and uncertainty analysis of 210Pb dates for PIRLA project lakes sediment cores. Journal of Paleolimnology 3:253–267.
- Bonan, G. B. 1992. Soil temperature as an ecological factor in boreal forests. In Shugart, H.H., Leemans, R., and Bonan, G.B. (eds.), A Systems Analysis of the Global Boreal Forest. Cambridge: Cambridge University Press. 126–307.
- Bradley, R. S. 2000. Past global changes and their significance for the future. Quaternary Science Reviews 19:391–402.
- Camburn, K. E. and D. F. Charles . 2000. Diatoms of Low Alkalinity Lakes in the Northeastern United States. The Academy of Natural Sciences of Philadelphia. Philadelphia: Scientific Publications. 152. pp.
- Cameron, N. G. , ØA. Schnell , M. L. Rautio , A. Lami , D. M. Livingstone , P. G. Appleby , J. A. Dearing , and N. L. Rose . 2002. High-resolution analyses of sediments from a Norwegian mountain lake and comparison with instrumental records of climate. Journal of Paleolimnology 28:79–93.
- Canada (Environment Canada) 1990. The Climates of Canada. Ottawa: Canadian Government Publishing Centre. 176. pp.
- Canada (Department of Energy, Mines and Resources) 1991. National Atlas of Canada, 5th ed. Ottawa: Department of Energy, Mines and Resources.
- Canada (Natural Resources Canada, Canadian Forest Service, and Geomatics Canada) 1996. National Atlas of Canada: The Boreal Forest.
- Barbieri Bitušik Kopáček 2002a. Seasonal ecosystem variability in remote mountain lakes: implications for detecting climatic signals in sediment records. Journal of Paleolimnology 28:25–46.
- Catalan, J. , S. Pla , M. Rieradevall , M. Felip , M. Ventura , T. Buchaca , L. Camarero , A. Brancelj , P. G. Appleby , A. Lami , J. A. Grytnes , A. Angusti-Panareda , and R. Thompson . 2002b. Lake Redó ecosystem response to an increasing warming in the Pyrenees during the twentieth century. Journal of Paleolimnology 28:129–145.
- Clarke, K. R. and R. M. Warwick . 1994. Change in Marine Communities: an approach to statistical analysis and interpretation. Bournemouth, UK: Natural Environment Research Council, Bourne Press Limited.
- Cremer, H. , B. Wagner , M. Melles , and H-W. Hubberten . 2001. The postglacial development of Raffles Sø, East Greenland: inferences from a 10,000 year diatom record. Journal of Paleolimnology 26:68–87.
- Cumming, B. F. , J. P. Smol , J. C. Kingston , D. F. Charles , H. J. B. Birks , K. E. Camburn , S. S. Dixit , A. J. Uutala , and A. R. Selle . 1992. How much acidification has occurred in Adirondack region lakes (New York, USA) since preindustrial times?. Canadian Journal of Fisheries and Aquatic Sciences 49:128–141.
- Cumming, B. F. , S. E. Wilson , R. I. Hall , and J. P. Smol . 1995. Diatoms from British Columbia (Canada) Lakes and Their Relationship to Salinity, Nutrients, and Other Limnological Variables. Bibliotheca Diatomologica, Vol. 31. Stuttgart: J. Cramer. 207. pp.
- Dixit, S. S. , J. P. Smol , D. F. Charles , R. M. Hughes , S. G. Paulsen , and G. B. Collins . 1999. Assessing water quality changes in the lakes of the northeastern United States using sediment diatoms. Canadian Journal of Fisheries and Aquatic Sciences 56:131–152.
- Doubleday, N. C. 1997. Arctic contaminants and the environment. In Fleming, T. (ed.), The Environment and Canadian Society. Toronto: International Thomson Publishing Company. 85–104.
- Douglas, M. S. V. , J. P. Smol , and W. Blake Jr . 1994. Marked post–18th century environmental change in High Arctic ecosystems. Science 266:416–419.
- Douglas, M. S. V. and J. P. Smol . 1999. Freshwater diatoms as indicators of environmental change in the High Arctic. In Stoermer, E., and Smol, J.P. (eds.), The Diatoms: Applications for the Environmental and Earth Sciences. Cambridge: Cambridge University Press. 227–244.
- Douglas, M. S. V. and J. P. Smol . 2000. Eutrophication and recovery in the High Arctic: Meretta Lake (Cornwallis Island, Nunavut, Canada) revisited. Hydrobiologia 431:193–204.
- Foged, N. 1981. Diatoms in Alaska. Bibliotheca Phycologica, Vol 53. Vaduz: J. Cramer, Verlag. 317. pp.
- Glew, J. R. 1988. A portable extruding device for close interval sectioning of unconsolidated core samples. Journal of Paleolimnology 1:235–239.
- Glew, J. R. 1991. Miniature gravity corer for recovering short sediments cores. Journal of Paleolimnology 5:285–287.
- Grove, J. M. 2001. The initiation of the ‘Little Ice Age’ in regions round the North Atlantic. Climatic Change 48:53–82.
- Hall, R. I. and J. P. Smol . 1996. Paleolimnological assessment of long-term water quality changes in south-central Ontario lakes affected by cottage development and acidification. Canadian Journal of Fisheries and Aquatic Sciences 53:1–17.
- Hein, M. K. 1990. Flora of Adak Island, Alaska: Bacillariophyceae (Diatoms). Bibliotheca Diatomologica. Berlin: J. Cramer. 133. pp.
- Houghton, J. T. , L. G. Meira Filho , B. A. Callander , N. Harris , A. Kattenberg , and K. Maskell . 1996. Climate Change 1995: The Science of Climate Change. Cambridge: Cambridge University Press.
- Hustedt, F. 1927–1966. Die Kieselagen Deutschlands, Österreichs und der Schweiz. In Dr. L. Rabenhorst's Kryptogamen–Flora von Deutschland, Osterreich und der Schweiz. Leipzig: Akademische Verlagsgesellschaft, 3 vols.
- Keyser, A. R. , J. S. Kimball , R. R. Nemani , and S. W. Running . 2000. Simulating the effects of climatic change on the carbon balance of North American high-latitude forests. Global Change Biology 6:Suppl. 1 185–195.
- Koinig, K. A. , C. Kamenik , R. Schmidt , A. Angusti-Panareda , P. Appleby , A. Lami , M. Prazakova , N. Rose , ØA. Schnell , R. Tessadri , R. Thompson , and R. Psenner . 2002. Environmental changes in an alpine lake (Gossenköllesee, Austria) over the last two centuries—the influence of air temperature on biological parameters. Journal of Paleolimnology 28:147–160.
- Korhola, A. , S. Sorvari , M. Rautio , P. G. Appleby , J. A. Dearing , N. Rose , A. Lami , and N. G. Cameron . 2002. A multi-proxy analysis of climate impacts on recent development of subarctic Lake Saanjärvi in Finnish Lapland. Journal of Paleolimnology 28:59–77.
- Korsman, T. 1999. Temporal and spatial trends of lake acidity in northern Sweden. Journal of Paleolimnology 22:1–15.
- Krammer, K. and H. Lange-Bertalot . 1986–1991. Bacillariophyceae. In Ettl, H., Gerloff, J., Heynig, H., and Mollenhauer D., (eds.), Süßwasserflora von Mitteleuropa, Volume 2 (1–4). Stuttgart/Jena: Gustav Fischer Verlag.
- Laird, K. R. , S. C. Fritz , B. F. Cumming , and E. C. Grimm . 1998. Early-Holocene limnological and climatic variability in the Northern Great Plains. The Holocene 8:275–285.
- Lange-Bertalot, H. and D. Metzeltin . 1996. Iconographia Diatomologica: Annotated Diatom Micrographs, Vol.2: Indicators of Oligotrophy. Lange-Bertalot, H. (ed.). Königstein, Germany: Koeltz Scientific Books. 390. pp.
- Lotter, A. F. , R. Pienitz , and R. Schmidt . 1999. Diatoms as indicators of environmental change near arctic and alpine treeline. In Stoermer, E.F., and Smol, J.P. (eds.), The Diatoms: Applications for the Environmental and Earth Sciences. Cambridge: Cambridge University Press. 205–226.
- Lotter, A. F. and C. Bigler . 2000. Do diatoms in the Swiss Alps reflect the length of ice-cover?. Aquatic Science 62:125–141.
- Lotter, A. F. , P. G. Appleby , R. Bindler , J. A. Dearing , J-A. Grytnes , W. Hofmann , C. Kamenik , A. Lami , D. M. Livingstone , C. Ohlendorf , N. Rose , and M. Sturm . 2002. The sediment record of the past 200 years in a Swiss high-alpine lake: Hagelseewi (2339 m.a.s.l.). Journal of Paleolimnology 28:111–127.
- MacDonald, G. M. and K. Gajewski . 1992. The northern treeline of Canada. In Janelle, D. G. (ed.), Geographical Snapshots of North America. New York: The Guilford Press. 34–37.
- MacDonald, G. M. , B. Felzer , B. P. Finney , and S. L. Forman . 2000. Holocene lake sediment records of Arctic hydrology. Journal of Paleolimnology 24:1–14.
- Michelutti, N. , T. E. Laing , and J. P. Smol . 2000. Diatom assessment of past environmental changes in lakes located near the Noril'sk (Siberia) smelters. Water, Air, and Soil Pollution 125:231–241.
- Michelutti, N. , M. S. V. Douglas , and J. P. Smol . in press. Diatom response to recent climatic change in a high arctic lake (Char Lake, Resolute Bay, Cornwallis Island, Nunavut). Global and Planetary Change .
- Muir, D. G. C. , A. Omelchenko , N. P. Grift , D. A. Savoie , W. L. Lockhart , P. Wilkinson , and G. J. Brunskill . 1996. Spatial trends and historical deposition of polychlorinated biphenyls in Canadian midlatitude and Arctic lake sediments. Environmental Science and Technology 30:3609–3617.
- Ohlendorf, C. , C. Bigler , G. Goudsmit , G. Lemecke , D. M. Livingstone , A. F. Lotter , B. Müller , and M. Sturm . 2000. Causes and effects of long periods of ice cover on a remote high Alpine lake. Journal of Limnology 59:Suppl. 1 65–80.
- Overpeck, J. , K. Hughen , D. Hardy , R. Bradley , R. Case , M. Douglas , B. Finney , K. Gajewski , G. Jacoby , A. Jennings , S. Lamoureux , A. Lasca , G. M. MacDonald , J. Moore , M. Retelle , S. Smith , A. Wolfe , and G. Zielinski . 1997. Arctic environmental change of the last four centuries. Science 278:1251–1256.
- Padgham, W. A. and W. K. Fyson . 1992. The Slave Province: a distinct Archean craton. Canadian Journal of Earth Sciences 29:2072–2086.
- Paterson, A. M. , A. A. Betts-Piper , J. P. Smol , B. A. Zeeb , and K. J. Reimer . in press. Diatom and chrysophyte algal response to long-term point-source PCB contamination in northern Labrador, Canada. Water, Air, and Soil Pollution .
- Patrick, R. and C. Reimer . 1966. The Diatoms of the United States. Vol. 1: Fragilariaceae , Eunotiaceae , Achnanthaceae , Naviculaceae . Philadelphia: The Academy of Natural Sciences of Philadelphia. 688. pp.
- Patrick, R. and C. Reimer . 1975. The Diatoms of the United States. Vol. 2: Entomoneidaceae , Cymbellaceae , Gomphonemaceae , Epithemiaceae . Philadelphia: The Academy of Natural Sciences of Philadelphia. 213. pp.
- Psenner, R. and R. Schmidt . 1992. Climate-driven pH control of remote alpine lakes and effects of acid deposition. Nature 356:781–783.
- Rautio, M. , S. Sorvari , and A. Korhola . 2000. Crustacean zooplankton and diatom communities, their seasonal variability, and representation in the sediments of subarctic Lake Saanajärvi. Journal of Limnology 59:Suppl. 1 81–96.
- Rawn, D. F. K. , W. L. Lockhart , P. Wilkinson , D. A. Savoie , G. B. Rosenberg , and D. C. G. Muir . 2001. Historical contamination of Yukon Lake sediments by PCBs and organochlorine pesticides: influence of local sources and watershed characteristics. The Science of the Total Environment 280:17–37.
- Ritchie, J. C. 1993. Northern vegetation. In French, H.M., and Slaymaker, O. (eds.), Canada's Cold Environments. Montreal and Kingston: McGill–Queen's University Press. 93–116.
- Rouse, W. R. , M. S. V. Douglas , R. E. Hecky , A. E. Hershey , G. E. Kling , L. Lesack , P. Marsh , M. McDonald , B. J. Nicholson , N. T. Roulet , and J. P. Smol . 1997. Effects of climate change on the freshwaters of arctic and subarctic North America. Hydrological Processes 11:873–902.
- Rühland, K. 2001. Diatom assemblage shifts relative to changes in environmental and climatic conditions in circumpolar treeline regions of the Canadian and Siberian Arctic. Ph.D. dissertation, Queen's University, Kingston, Ontario, Canada. 265. pp.
- Rühland, K. and J. P. Smol . 2002. Freshwater diatoms from the Canadian Arctic treeline and development of paleolimnological inference models. Journal of Phycology 38:249–264.
- Rühland, K. , J. P. Smol , and R. Pienitz . in press. Ecology and spatial distributions of surface-sediment diatoms from 77 lakes in the subarctic Canadian treeline region. Canadian Journal of Botany .
- Serreze, M. C. , J. E. Walsh , F. S. Chapin III , T. Osterkamp , M. Dyurgerov , V. Romanovsky , W. C. Oechel , J. Morison , T. Zhang , and R. G. Barry . 2000. Observational evidence of recent change in the northern high-latitude environment. Climatic Change 46:159–207.
- Sirois, L. 1992. The transition between boreal forest and tundra. In Shugart, H.H., Leemans, R., and Bonan, G.B. (eds.), A System Analysis of the Global Boreal Forest. Cambridge: Cambridge University Press. 196–215.
- Smith, I. R. 2002. Diatom-based Holocene paleoenvironmental records from continental sites on northeastern Ellesmere Island, high Arctic, Canada. Journal of Paleolimnology 27:9–28.
- Smol, J. P. 1983. Paleophycology of a high arctic lake near Cape Herschel, Ellesmere Island. Canadian Journal of Botany 61:2195–2204.
- Smol, J. P. 1988. Paleoclimate proxy data from freshwater arctic diatoms. Verhundlungen der Internationalen Vereinigung von Limnologen 23:837–844.
- Smol, J. P. , B. F. Cumming , A. S. Dixit , and S. S. Dixit . 1998. Tracking recovery patterns in acidified lakes: a paleolimnological perspective. Restoration Ecology 6:318–326.
- Smol, J. P. 2002. Pollution of Lakes and Rivers: A Paleoenvironmental Perspective. London: Arnold Publishing. 280. pp.
- Sorvari, S. and A. Korhola . 1998. Recent diatom assemblage change in subarctic Lake Saanajärvi, NW Finnish Lapland, and their paleoenvironmental implications. Journal of Paleolimnology 20:205–215.
- Sorvari, S. , A. Korhola , and R. Thompson . 2002. Lake diatom response to recent Arctic warming in Finnish Lapland. Global Change Biology 8:153–163.
- Stoermer, E. F. and J. P. Smol . 1999. Applications and uses of diatoms: prologue. In Stoermer, E.F., and Smol, J.P. (eds.), The Diatoms: Applications for the Environmental and Earth Sciences, Cambridge: Cambridge University Press. 3–8.
- Stuiver, M. , P. J. Reimer , E. Bard , J. W. Beck , G. S. Burr , K. A. Hughen , B. Kromer , F. G. McCormac , J. van der Plicht , and M. Spurk . 1998. INTCAL98 radiocarbon age calibration 24,000—0 cal BP. Radiocarbon 40:1041–1083.
- Timoney, K. P. , G. H. LaRoi , S. C. Zoltoi , and A. L. Robinson . 1992. The high subarctic forest-tundra of northwestern Canada: position, width, and vegetation gradients in relation to climate. Arctic 45:1–9.
- Timoney, K. P. , G. H. LaRoi , S. C. Zoltoi , and A. L. Robinson . 1993. Vegetation communities and plant distributions and their relationships with parent materials in the forest-tundra of northwestern Canada. Ecography 16:174–188.
- Wedel, J. H. , A. Smart , and P. Squires . 1990. An Overview Study of the Yellowknife River Basin, N.W.T. N.W.T. Programs. Inland Waters Directorate Conservation and Protection, Western and Northern Region, Environment Canada. 82. pp.
- Wolfe, A. P. 2000. A 6500-year diatom record from southwestern Fosheim Peninsula, Ellesmere Island, Canadian High Arctic. In Garneau, M., and Alt, B.T., (eds.). Environmental Response to Climate Change in the High Arctic, Geological Survey of Canada Bulletin 529:249–256.
- Wolfe, A. P. , J. S. Baron , and R. J. Cornett . 2001. Anthropogenic nitrogen deposition induces rapid ecological changes in alpine lakes of the Colorado Front Range (USA). Journal of Paleolimnology 25:1–7.
- Yalcin, K. and C. P. Wake . 2001. Anthropogenic signals recorded in an ice core from Eclipse Icefield, Yukon Territory, Canada. Geophysical Research Letters 28:4487–4490.