Abstract
To evaluate edge effects in subalpine coniferous forest, I quantified stand structure characteristics in a plot that extended into the forest from a road that had been constructed approximately 30 yr previously on Mt. Fuji, in central Japan. The basal area of standing dead trees and the density of saplings in the plot were greater near the road. However, cluster analysis indicated that stand structure near the road edge was similar to that of gaps in the interior of the stand. Since edge effects may result from interactions among several variables, analyzing data sets that include several variables rather than a single variable is a promising approach for evaluating edge effects. In a multiple regression analysis, sapling densities of Tsuga diversifolia and Abies veitchii were strongly affected by the basal area of standing dead trees that had apparently died as a result of the construction. A. veitchii is more shade intolerant than the other two main species in the study plot, but it responds quickly to increased light availability. Although the plot is currently composed of T. diversifolia and A. mariesii, successful regeneration of A. veitchii may eventually change the species composition of this plot.
Introduction
Road construction changes the spatial patterns of a forested landscape. The process, defined as dissection (CitationForman, 1995), creates an edge and severely affects the biotic and abiotic environments of the surrounding areas (e.g., CitationSchonewald-Cox and Buechner, 1992; CitationNoss and Csuti, 1994; CitationYoung, 1994). These edge effects, which are caused not only by road construction but also by other human impacts that transform a landscape, such as clear-cutting, have been widely reported (e.g., CitationHarris, 1984; CitationBrothers and Spingarn, 1992; CitationFraver, 1994; CitationYoung and Mitchell, 1994). Evaluations of edge effects have varied, depending on the species and scale context, from their being considered a traditionally positive effect, especially for wildlife (see CitationYahner, 1988), to being considered a negative effect on biodiversity (e.g., CitationNoss, 1983; CitationHarris, 1988; CitationChen et al., 1992). Most research on edge effects has focused on measuring the extent of their influence (e.g., CitationWilliams-Linere, 1990; CitationMatlack, 1993).
It is important for management and restoration purposes to measure the “edge depth,” i.e., the distance from the edge to the forest interior, to maximize the amount of interior habitat (CitationLaurance and Yensen, 1991; CitationSisk and Margules, 1993). However, because edge effects may be caused by interactions among several variables, including biotic and abiotic parameters, edge depth is difficult to define and to measure (CitationMurcia, 1995; CitationCadenasso et al., 1997; CitationEsseen and Renhorn, 1998). In addition, because edge effects do not have clear boundaries, the influence of an edge follows a gradient (CitationFraver, 1994). Since species respond to edges in different ways (CitationPalik and Murphy, 1990; CitationMatlack, 1994), CitationMurcia (1995) suggested that any measurement of edge effects should consider which spatial and temporal scales are most relevant for each species. Some researchers (CitationMurcia, 1995; CitationCadenasso et al., 1997; CitationEsseen and Renhorn, 1998; CitationMcCollin, 1998) have pointed out that in a large proportion of existing studies, the definition of an edge is not based on functional properties.
A plant community consists of a mosaic of patches at different stages of development (CitationWatt, 1947). Mosaic structures, consisting of patches that vary in size, age structure, and developmental stage, have been identified in many forest types (e.g., CitationNakashizuka, 1983; CitationVeblen, 1986; CitationLiu and Hytteborn, 1991; CitationLertzman, 1992; CitationYamamoto, 1992a; CitationHiura et al., 1996). The development and regeneration of forest starts from a disturbance (e.g., CitationYamamoto, 1992b; CitationRunkle, 1998). To show how patches develop, various phases of forest development must be compared. Therefore, any comparison of stand structure along the edge and in the interior should consider the mosaic structure of the interior. Previous research on edge effects in forests has paid little attention to this point. To demonstrate the stand structure of an edge, it must be compared to the interior and to different phases of stand development within the interior. In addition, it is important to predict the dynamics, development, and restoration of the stand structure of an edge; to date, however, few studies have evaluated stand development along edges.
Mt. Fuji is the highest mountain in Japan (3776 m a.s.l.). Subalpine coniferous forests dominated by Tsuga diversifolia, Abies mariesii, and A. veitchii cover the north slope from 1500 to 2400 m (CitationFranklin et al., 1979; CitationOhsawa, 1984). Stand structure and regeneration in the forest are affected primarily by gap formation, which creates a mosaic of patches (CitationNakamura, 1985). CitationKanzaki (1984) showed that the canopy layer of a T. diversifolia forest in central Japan comprised a mosaic of patches arising from gaps that had formed at different times.
In 1964, a road for sightseeing was constructed through the primary coniferous forest in the subalpine zone of Mt. Fuji. The subsequent death of canopy trees in the edges alongside the road was attributed to road construction (CitationMaeda et al., 1998). Approximately 30 yr after the road was constructed, CitationMaeda et al. (1998) showed that conifers had successfully regenerated, especially along the edges. However, to better understand the process of forest development that has shaped the current stand structure, it is necessary to quantify the regeneration and recovery of the stand.
Using stand structure variables, I quantified the edge effects in this forest. Specifically, my objectives were to determine (1) the phase of forest development near the edge and (2) whether species differed in their regeneration responses following the death of canopy trees.
Study Site and Methods
The study plot was located 2100 m a.s.l. on the north slope of Mt. Fuji (35° N, 138°E). CitationNakamura and Obata (1982) found that the mean monthly temperature on Mt. Fuji (at 1900 m) ranged from 16.4°C in August to −7.6°C in January, the mean annual temperature was 4.3°C, and the mean annual precipitation measured 2300 mm. These values indicate that the study site is located in the subalpine zone. However, the maximum snow depth was approximately 1 m, suggesting that the study site is not in the region of Japan that experiences very heavy snowfall. Before the road was constructed in 1964, the forest was undisturbed. Unfortunately, stand structure and species composition along the road were not recorded prior to construction.
The road is 29.5 km long and climbs from 857 to 2305 m a.s.l.; about 15 km is in subalpine forest. Many canopy trees along the road have died, apparently as a result of the road construction (CitationMaeda et al., 1998). Although the reasons cannot be definitively proven, exposure to strong winds can cause die-backs following road construction. Because standing dead trees along the road were considered unsightly, they were cut down; thus, most of the forest along the road is no longer in a natural condition, with standing dead trees. Standing dead trees were left uncut in only a small part of the forest along the road, to serve as a control site.
I established my study plot in this control site in 1999. The plot was 50 × 140 m (0.7 ha), with the long side of the plot perpendicular to the road. A zone about 10 m wide along the roadside was not included in the plot because the road construction had destroyed the slope. The plot was divided into a 10 × 10–m grid (70 quadrats in total). Since the mean canopy gap size in primary Abies-Tsuga forests located 150 km north of this study site was 38.3–84.3 m2 (CitationYamamoto, 1995; CitationNarukawa and Yamamoto, 2001), this grid size was considered suitable to analyze the developmental phases of stand structure. Both live trees and standing dead trees >2 m tall were tagged and identified within each quadrat. Fallen dead trees were not measured. Several standing dead trees could not be identified because of bark decomposition. I measured the diameter at breast height (DBH) using a steel tape measure, or diameter vernier calipers for smaller trees. Saplings were defined as trees with a DBH of <3 cm and “large trees” as trees with a DBH ≥30 cm. For Rhododendron brachycarpum, a shrub with many stems, only the largest stem of an individual was tagged and measured. Several studies have indicated that Rhododendron spp. impede tree regeneration (e.g., CitationBaker and Van Lear, 1998). However, I found no significant correlations between the individual density of R. brachycarpum and conifer sapling density (T. diversifolia, r = −0.044, P = 0.801; A. mariesii, r = −0.119, P = 0.548; and A. veitchii, r = −0.176, P = 0.344), so any effects of R. brachycarpum on regeneration were omitted from the analysis. A bryophyte, Hylocomium splendens, dominated the forest floor.
Statistical analyses (e.g., cluster analysis and simple correlations among stand attributes) were confined to the three dominant conifers (T. diversifolia, A. mariesii, and A. veitchii). Analyses were performed separately for each quadrat. Cluster analysis was used to examine the phase of forest development and regeneration near the edge, using a data set that included the basal areas of both live and standing dead trees and the densities of both large live trees (DBH ≥30 cm) and saplings of the three dominant species. The dendrogram using the average linkage between groups was separated into four groups at 15% of the squared Euclidean distance (similarity). The four groups were randomly assigned the letters A, B, C, and D. To show whether there were species differences in the regeneration response following the death of canopy trees, I used a simple correlation for each dominant species. Also, multiple regression analysis was performed with sapling density as the dependent variable and stand structure parameters (the basal area of both live trees and standing dead trees and the densities of large live trees) as the explanatory variables.
Results
The dominant species in the stand in terms of both basal area and density was T. diversifolia (). Three conifers (T. diversifolia, A. mariesii, and A. veitchii) contributed 99.4% of the basal area and 86.0% of the density. The skew of the DBH distribution of T. diversifolia and A. mariesii was similar, while that of A. veitchii was much higher. This difference indicates that the DBH distribution of A. veitchii was considerably more biased toward smaller size classes than the distributions of the other two species. The DBH distribution differed significantly among the three species (Kruskal-Wallis test: P < 0.01, Bonferroni's multiple comparison: P < 0.01 for each combination). Unlike the other two species, the basal area value for A. veitchii was considerably lower than that of density. In each of the three species, the DBH of live trees was significantly smaller than that of the standing dead trees (Mann-Whitney U-test: each P < 0.01).
Based on attributes of stand structure and regeneration, the edge effect varied with distance from the road. The basal area of standing dead trees was larger and the density of saplings was greater near the road (). Saplings of both T. diversifolia and A. veitchii were abundant near the edge, while those of A. mariesii were not (). Cluster analysis showed that quadrats in the interior of the stand were structurally similar to those near the edge (). These quadrats, which belonged to group A, characterized by a high basal area of standing dead trees, few large trees, and high sapling density, were in the gap phase of forest development ().
Sapling density was negatively correlated to both the basal area of live trees and the density of large trees, and positively correlated to the basal area of standing dead trees (). Since the simple correlation coefficients between the density of large conspecific trees and sapling density were not significant for each combination, sapling density was not dependent on the density of conspecific trees (). The results of multiple regression analysis with sapling density as the dependent variable showed that the factors affecting regeneration differed by species (). The sapling density of T. diversifolia and A. veitchii was strongly affected by the basal area of standing dead trees. Both the standardized partial correlation coefficient and the coefficient of determination (R2) of A. veitchii were higher than those of T. diversifolia. This model of multiple regression analysis was not significant for A. mariesii, indicating that the stand characteristics of saplings of this species differ from those of the other species.
Discussion
FOREST DEVELOPMENT NEAR EDGES
Canopy openings resulting from the death of canopy trees caused by natural (e.g., CitationTaylor and Qin, 1992; CitationKubota, 1995) and human (e.g., CitationKneeshaw et al., 1998; CitationNagaike et al., 1999; CitationVan Pelt and Franklin, 1999) disturbances change stand structure and improve the light environment in the understory. The positive correlation between sapling density and the basal area of the standing dead trees that resulted from road construction implies that the death of canopy trees improved the light environment under the canopy and created gaps (e.g., CitationNakashizuka, 1985; CitationCanham et al., 1990, and ). It is widely recognized that some shade-tolerant conifers undergo advanced regeneration (i.e., form seedling and sapling banks; e.g., CitationGreene et al., 1999; CitationMessier et al., 1999). I found no correlation between the densities of conspecific trees and saplings, suggesting that regeneration depends on improvement of the light environment following the formation of canopy gaps rather than on advanced regeneration ().
As shown in , it is difficult to quantify the extent of the edge effects from the road because they depend on attributes of stand structure. Cluster A in ) indicates the gap phase in the course of forest development; this is the initial phase of regeneration and is characterized by recruitment (CitationWatt, 1947; CitationNakashizuka, 1984). As CitationMurcia (1995), CitationCadenasso et al. (1997), and CitationEsseen and Renhorn (1998) have pointed out, edge effects may be caused by interactions among several variables. Therefore, it is necessary to evaluate edge effects using data sets that combine several variables, as CitationFraver (1994) and this study did.
DIFFERENCES IN SPECIES' RESPONSES FOLLOWING THE DEATH OF CANOPY TREES
The DBH distribution of A. veitchii was more skewed and had a smaller average than the distributions of T. diversifolia and A. mariesii (). Therefore, smaller size classes were more abundant in A. veitchii than in the other two species, showing that A. veitchii was regenerating successfully. Regeneration of A. veitchii continued between 1999 and 2001 (Nagaike, Abe, and Arai, unpublished data). The distribution of sapling density of each species differed, indicating that response to the improvement in light conditions varied by species (). The diameter and apical growth of A. veitchii exceed those of T. diversifolia in gaps (CitationNakamura and Obata, 1982; CitationKanzaki, 1984).
CitationKanzaki (1984) described the regeneration processes in a T. diversifolia–A. veitchii forest as follows: (1) after a disturbance, Abies regenerates from seedling and sapling pools and grows rapidly; subsequently, (2) pure stands of Tsuga remain after the death of Abies, which has a shorter life span. CitationYamamoto (1993, Citation1995) showed that A. mariesii and A. veitchii frequently recruited after gap formation, whereas T. diversifolia did so less frequently. CitationNakamura and Obata (1982, Citation1985) and CitationNakamura (1992) also pointed out that A. veitchii could form patches of saplings beneath canopy gaps in a T. diversifolia forest due to the difference in the survival ratio of seedlings and their positive response to increased light. In a T. diversifolia–A. veitchii forest near this study stand, regeneration was dominated by A. veitchii (CitationMaeda et al., 1998). In Abies-Tsuga-Chamaecyparis forests of British Columbia, A. amabilis gap-fillers (trees that replace dead individuals in gaps) were more abundant than T. heterophylla or T. mertensiara (CitationLertzman, 1995). Therefore, it seems that Abies spp. generally outperform Tsuga during the sapling phase, suggesting that their growth response to increased light is better ().
CitationKohyama (1984) and CitationKimura et al. (1986) reported that A. veitchii saplings grew taller more quickly than A. mariesii and that the rapidly growing A. veitchii dominated A. mariesii in simultaneously decayed stands. Abies mariesii is more shade tolerant than A. veitchii (CitationKimura, 1963), and the photosynthetic response to improvement of the light environment at the leaf level is better in A. veitchii than in A. mariesii (T. Tanaka, unpublished data). The sapling density of A. veitchii in the study stand exceeded that of A. mariesii () and was strongly affected by the basal area of standing dead trees (). Moreover, the model of multiple regression analysis in the case of A. veitchii was the most suitable, suggesting that saplings of this species were more responsive to the death of canopy trees following road construction.
It may be difficult to show an edge effect in the species composition of saplings. The death of canopy trees following road construction alters the light environment in the understory, and A. veitchii, which is more shade intolerant than the other two species and quickly responds to increased light, may become the dominant species. While the density of canopy A. mariesii was greater than that of A. veitchii, the sapling density was lower. These results, which are likely caused by road construction, differ from those of a study conducted by CitationKohyama (1984) in a primary forest. He demonstrated that A. mariesii dominates A. veitchii in the understory of any mature stand and that A. veitchii is the competitively superior species in seedling-bank regeneration, while A. mariesii is superior in sapling-bank regeneration. Although the study stand is currently a mixed T. diversifolia forest, successful regeneration of A. veitchii may eventually change the species composition until a successor forms a canopy over A. veitchii. These results suggest that road construction affects not only stand structure but also species composition.
In conclusion, even though the road was constructed 30 yr before the time of this study, the stand structure near the edge still resembles that of gaps, and the species composition in the stand may have been changed by the road construction. CitationKohyama and Fujita (1981) and CitationKohyama (1982) showed in an Abies forest in central Japan that patches with a high density of saplings, which seem to indicate a gap phase, advance to a self-thinning stage. After this stage, it is unknown whether the original species composition of the stand is restored. The community dynamics (changes in species composition and density) in the study stand should therefore be monitored.
FIGURE 1. Dispersion maps of attributes of stand structure and the results of cluster analysis in the study stand. The cluster analysis was performed using a data set that included the basal areas (BAs) of both live trees and standing dead trees, and the densities of both larger live trees (DBH > 31cm) and saplings of a total of 3 species. The dendrogram using the average linkage between groups was separated into 4 groups at 15% of the squared Euclidean distance (similarity). The 4 groups were randomly assigned the letters A, B, C, and D
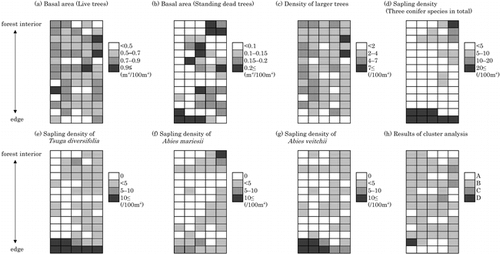
Table 1 Structure of live and standing dead trees of the stand
Table 2 Simple correlation coefficients among stand attributes
Table 3 Simple correlation coefficients between the densities of larger trees and saplings in the stand
Table 4 Results of multiple regression analysis with sapling density as the dependent variable
Acknowledgments
I would like to thank Midori Abe and Mio Hamanaka of Niigata University and Hisashi Sugita of the Forestry and Forest Products Research Institute for their help collecting field data. I would also like to thank the staff of the Yamanashi Forest Research Institute and of the Landscape Management Laboratory of the Kansai Branch of the Forestry and Forest Products Research Institute for their kind encouragement. This research was part of a research project, “Sustainability and Biodiversity Assessment of Forest Utilization Options,” by the Research Institute for Humanity and Nature, Japan.
References Cited
- Baker, T. T. and D. H. Van Lear . 1998. Relations between density of rhododendron thickets and diversity of riparian forests. Forest Ecology and Management 109:21–32.
- Brothers, T. S. and A. Spingarn . 1992. Forest fragmentation and alien plant invasion of central Indiana old-growth forests. Conservation Biology 6:91–100.
- Cadenasso, M. L. , M. M. Traynor , and S. T A. Pickett . 1997. Functional location of forest edges: gradients of multiple physical factors. Canadian Journal of Forest Research 27:774–782.
- Canham, C. D. , J. S. Denslow , N. J. Platt , J. R. Runkle , T. A. Spies , and P. S. White . 1990. Light regimes beneath closed canopies and tree-fall gaps in temperate and tropical forests. Canadian Journal of Forest Research 20:620–631.
- Chen, J. , J. F. Franklin , and T. A. Spies . 1992. Vegetation responses to edge environments in old-growth Douglas-fir forests. Ecological Applications 2:387–396.
- Esseen, P-A. and K-E. Renhorn . 1998. Edge effects on an epiphytic lichen in fragmented forests. Conservation Biology 12:1307–1317.
- Forman, R. T T. 1995. Land Mosaics: The Ecology of Landscapes and Regions. Cambridge: Cambridge University Press. 632 pp.
- Franklin, J. F. , T. Maeda , Y. Ohsumi , M. Matsui , H. Yagi , and G. M. Hawk . 1979. Subalpine coniferous forests of central Honshu, Japan. Ecological Monographs 49:311–334.
- Fraver, S. 1994. Vegetation responses along edge-to-interior gradients in the mixed hardwood forests of the Roanoke river basin, North Carolina. Conservation Biology 8:822–832.
- Greene, D. F. , J. C. Zasada , L. Sirois , D. Kneeshaw , H. Morn , I. Chamon , and M-J. Simard . 1999. A review of the regeneration dynamics of North American boreal forest tree species. Canadian Journal of Forest Research 29:824–839.
- Harris, L. D. 1984. The Fragmented Forest: Island Biogeography Theory and the Preservation of Biotic Diversity. Chicago: University of Chicago Press. 211 pp.
- Harris, L. D. 1988. Edge effects and conservation of biotic diversity. Conservation Biology 2:330–332.
- Hiura, T. , J. Sano , and Y. Konno . 1996. Age structure and response to finer-scale disturbances of Abies sachalinensis , Picea jezoensis , Picea glehnii , and Betula ermanii growing under the influence of a dwarf bamboo understory in northern Japan. Canadian Journal of Forest Research 26:289–297.
- Kanzaki, M. 1984. Regeneration in subalpine coniferous forests. I. Mosaic structure and regeneration process in a Tsuga diversifolia forest. Botanical Magazine Tokyo 97:297–311.
- Kimura, M. 1963. Dynamics of vegetation in relation to soil development in the northern Yatsugatake Mountains. Japanese Journal of Botany 18:255–287.
- Kimura, M. , W. Kimura , S. Honma , T. Hasuno , and T. Sasaki . 1986. Analysis of development of a subalpine Abies stand based on the growth processes of individual trees. Ecological Research 1:229–248.
- Kneeshaw, D. , Y. Bergeron , and L. De Grandpré . 1998. Early response of Abies balsamea seedlings to artificially created openings. Journal of Vegetation Science 9:543–550.
- Kohyama, T. 1982. Studies on the Abies population of Mt. Shimagare. II. Reproduction and life history traits. Botanical Magazine Tokyo 95:167–181.
- Kohyama, T. 1984. Regeneration and coexistence of two Abies species dominating subalpine forests in Japan. Oecologia 62:156–161.
- Kohyama, T. and N. Fujita . 1981. Studies on the Abies population of Mt. Shimagare. I. Survivorship curve. Botanical Magazine Tokyo 94:55–68.
- Kubota, Y. 1995. Effects of disturbance and size structure on the regeneration process in a sub-boreal coniferous forest, northern Japan. Ecological Research 10:135–142.
- Laurance, W. F. and E. Yensen . 1991. Predicting the impacts of edge effects in fragmented habitats. Biological Conservation 55:77–92.
- Lertzman, K. P. 1992. Patterns of gap-phase replacement in a subalpine, old-growth forest. Ecology 73:657–669.
- Lertzman, K. P. 1995. Forest dynamics, differential mortality and variable recruitment probabilities. Journal of Vegetation Science 6:191–204.
- Liu, Q. and H. Hytteborn . 1991. Gap structure, disturbance and regeneration in a primeval Picea abies forest. Journal of Vegetation Science 2:391–402.
- Maeda, T. , T. Osada , M. Kikuchi , Y. Kanbe , S. Asanuma , T. Kato , T. Shinozaki , and T. Satomichi . 1998. Revegetation on the destroyed stands caused by road construction of “Subaru line” at Mt. Fuji—a report of survey at 30 years after opening the road. Japanese Journal of Forest Environment 40:43–47. (in Japanese).
- Matlack, G. R. 1993. Microenvironment variation within and among forest edge sites in the eastern United States. Biological Conservation 66:185–194.
- Matlack, G. R. 1994. Vegetation dynamics of the forest edge—trends in space and successional time. Journal of Ecology 82:113–123.
- McCollin, D. 1998. Forest edges and habitat selection in birds: a functional approach. Ecography 21:247–260.
- Messier, C. , R. Doucet , J-C. Ruel , Y. Clareau , C. Kelly , and M. J. Lechowicz . 1999. Functional ecology of advance regeneration in relation to light in boreal forests. Canadian Journal of Forest Research 29:812–823.
- Murcia, C. 1995. Edge effects in fragmented forests: implications for conservation. Trends in Ecology and Evolution 10:58–62.
- Nagaike, T. , Y. Kubota , and N. Watanabe . 1999. The effects of selective logging on stand structure and the regeneration of subboreal forests in Hokkaido, northern Japan. Journal of Forest Research 4:41–45.
- Nakamura, T. 1985. Forest succession in the subalpine region of Mt. Fuji, Japan. Vegetatio 64:15–27.
- Nakamura, T. 1992. Effect of bryophytes on survival of conifer seedlings in subalpine forests of central Japan. Ecological Research 7:155–162.
- Nakamura, T. and T. Obata . 1982. Differences in ecological character between Abies veitchii and Tsuga diversifolia . I. Growth of saplings in gaps of subalpine forest on Mt. Fuji. Bulletin of Tokyo University Forest 72:131–138. (In Japanese with English summary.).
- Nakamura, T. and T. Obata . 1985. Differences in ecological character between Abies veitchii and Tsuga diversifolia . II. Distribution of seedlings on the moss-covered floor of Tsuga forest on Mt. Fuji. Bulletin of Tokyo University Forest 74:67–79. (In Japanese with English summary.).
- Nakashizuka, T. 1983. Regeneration process of climax beech ( Fagus crenata Blume) forests. III. Structure and development processed of sapling populations in different aged gaps. Japanese Journal of Ecology 33:409–418.
- Nakashizuka, T. 1984. Regeneration process of climax beech ( Fagus crenata Blume) forests. V. Population dynamics of beech in a regeneration process. Japanese Journal of Ecology 34:411–419.
- Nakashizuka, T. 1985. Diffused light conditions in canopy gaps in a beech ( Fagus crenata Blume) forest. Oecologia 66:472–474.
- Narukawa, Y. and S. Yamamoto . 2001. Gap formation, microsite variation and the conifer seedling occurrence in a subalpine old-growth forest, central Japan. Ecological Research 16:617–625.
- Noss, R. F. 1983. A regional landscape approach to maintain diversity. Bioscience 33:700–706.
- Noss, R. F. and B. Csuti . 1994. Habitat fragmentation. In Meffe, G. K., and Carroll, C. R. (eds.), Principles of Conservation Biology. Sunderland, MA: Sinauer Associates, Inc., 237–264.
- Ohsawa, M. 1984. Differentiation of vegetation zones and species strategies in the subalpine region of Mt. Fuji. Vegetatio 57:15–52.
- Palik, B. J. and P. G. Murphy . 1990. Disturbance versus edge effects in sugar-maple/beech forest fragments. Forest Ecology and Management 32:187–202.
- Runkle, J. R. 1998. Changes in southern Appalachian canopy tree gaps sampled thrice. Ecology 79:1768–1780.
- Schonewald-Cox, C. and M. Buechner . 1992. Park protection and public roads. In P. Fiedler P., and Jain S. (eds.), Conservation Biology. New York: Chapman and Hall, 373–395.
- Sisk, T. D. and C. R. Margules . 1993. Habitat edges and restoration: methods for quantifying edge effects and predicting the results of restoration efforts. In Saunders, D. A., Hobbs, R. J., and Ehrlich, P. R. (eds.), Nature Conservation 3: Reconstruction of Fragmented Ecosystems. Chipping Norton, Australia: Surrey Beatty and Sons, 57–69.
- Taylor, A. H. and Z. Qin . 1992. Tree regeneration after bamboo die-back in Chinese Abies - Betula forests. Journal of Vegetation Science 3:253–260.
- Van Pelt, R. and J. F. Franklin . 1999. Response of understory trees to experimental gaps in old-growth Douglas-fir forests. Ecological Applications 9:504–512.
- Veblen, T. T. 1986. Treefalls and coexistence of conifers in subalpine forests of the central Rockies. Ecology 67:644–649.
- Watt, A. S. 1947. Patterns and process in the plant community. Journal of Ecology 35:1–22.
- Williams-Linere, G. 1990. Vegetation structure and environmental conditions of forest edges in Panama. Journal of Ecology 78:356–373.
- Yahner, R. H. 1988. Changes in wildlife communities near edges. Conservation Biology 2:333–339.
- Yamamoto, S. 1992a. Gap characteristics and regeneration in primary evergreen broad-leaved forests of western Japan. Botanical Magazine Tokyo 105:29–45.
- Yamamoto, S. 1992b. The gap theory in forest dynamics. Botanical Magazine Tokyo 105:375–383.
- Yamamoto, S. 1993. Gap characteristics and regeneration in a subalpine coniferous forest on Mt. Ontake, central Japan. Ecological Research 8:277–285.
- Yamamoto, S. 1995. Gap characteristics and gap regeneration in subalpine old-growth coniferous forests, central Japan. Ecological Research 10:31–39.
- Young, A. and N. Mitchell . 1994. Microclimate and vegetation edge effects in a fragmented Podocarp-broadleaf forest in New Zealand. Biological Conservation 67:63–74.
- Young, K. R. 1994. Roads and the environmental degradation of tropical montane forests. Conservation Biology 8:972–976.