Abstract
We examined the impact of Salix lapponum canopies on plant community structure in five sites along a climatic gradient in a glacier foreland in alpine south Norway. Species richness is lower inside canopies compared to outside in climatically relatively benign communities, while species richness is not affected by canopies in the most severe communities closest to the glacier. Differences in species composition inside and outside canopies, as judged by detrended correspondence analysis, are greater in the benign communities compared to the severe communities. Variation in the differences in species richness or composition outside and inside canopies within a community is related to differences in the reduction of photosynthetically active radiation (PAR) by canopies in only one community. Canopy size does not explain differences in species richness or composition between outside and inside canopies in any except one benign community. Our results suggest that species responses to canopies are individualistic, and that at the whole-community level negative and positive impacts of canopies on species occurrences cancel each other out in severe communities, whereas in benign communities negative effects dominate slightly over positive ones in their effects on species persistence inside canopies.
Introduction
Several studies have found that shrubs or trees function as “nurse” plants for other plant species growing in their understorey, or as so-called “nuclei” for high community diversity (CitationNiering et al., 1963; CitationCallaway, 1995; CitationPugnaire and Haase, 1996). The primary causes for the positive impacts of woody species on the population dynamics of other species and on community richness are proposed to be reduced negative impacts from herbivores (e.g., CitationMcAuliffe, 1988; CitationLevine, 2000), increased nutrient availability (CitationGutiérrez et al., 1993; CitationAlstad and Vetaas, 1994), increased soil water availability (CitationJoffre and Rambal, 1993), and reduced exposure to wind (CitationValiente-Banuet and Ezcurra, 1991). Although interspecific competition has always been recognized as important for species composition, diversity, and richness of communities (e.g., CitationClements, 1916; CitationMacArthur, 1972; CitationKeddy, 1989) the importance of facilitation for community processes has been critically examined only during the last few decades (e.g., CitationWilson and Agnew, 1992; CitationBengtsson et al., 1994; CitationBertness and Hacker, 1994; CitationCallaway, 1995; CitationCallaway and Walker, 1997), although its potential importance was mentioned already by CitationClements (1916). Positive interactions among plants have been documented in a wide range of habitat types (see CitationCallaway, 1995, for a review), such as arid habitats and deserts (CitationMcAuliffe, 1988; CitationPugnaire and Haase, 1996), the arctic (CitationCarlsson and Callaghan, 1991; CitationChapin et al., 1994), and salt marshes (CitationBertness and Hacker, 1994). One conclusion emerging is that positive interactions appear to be more common in physically harsh environments than in more benign physical environments (CitationBertness and Callaway, 1994; CitationCallaway, 1995; CitationHolmgren et al., 1997). This conclusion is also consistent with CitationGrime's (1979) hypothesis that competition intensifies along gradients of increasing productivity.
Most previous studies on the interplay between competition and facilitation along environmental gradients have focused on a few species. Typically, one potential competitor/facilitator is selected and its impact on one or two other species examined. Although such experimental studies are useful in revealing causation of interactions, and have contributed greatly to our understanding of interactions between plant species (CitationCallaway, 1995), we still know relatively little about how interactions affect the patterns at the whole-community level because individualistic responses of species may make up-scaling from interaction patterns between few species to whole-community pattern uncertain (CitationConnell, 1983, CitationHacker and Gaines, 1997). Therefore, there is a need for studies that focus all vascular plant species at the community level in an integrated way.
In this study we examine species richness and composition outside and inside canopies of a shrub willow (Salix lapponum) in five alpine communities situated along a gradient of environmental severity. In addition, we examine how properties of individual canopies (reduction of photosynthetically active radiation [PAR] and canopy size) affect differences in species composition and richness outside and inside the canopies. In particular we ask: (1) Do willow canopies affect species richness and composition? (2) If so, is the impact different in communities situated along a gradient of environmental severity? (3) Are there relationships between the reduction in PAR or the size of individual canopies and differences in species composition and richness outside and inside canopies? (4) If so, do relationships between canopy properties and species composition and richness differ along the gradient of environmental severity?
Materials and Methods
STUDY AREA
The study was done in five plant communities dominated by Salix lapponum within the Finse valley in the northern part of Hardangervidda in alpine southwest Norway. The mean monthly temperatures at 1224 m elevation in the Finse area are 5.0, 7.0, 6.8, 3.0, and −0.5°C for June, July, August, September, and October, respectively (CitationAune, 1993). The mean monthly precipitation for the same months is 69, 88, 111, 128, and 125 mm (CitationFørland, 1993). Our study communities () are situated in two areas of contrasting climatic severity. The three communities (A–C) experiencing the most benign environmental conditions are situated on the south-facing slope of Kvannjolnuten at 1320 (A) and 1270 (B) m elevation and at the bottom of the Finse valley (C) at ca. 1220 m elevation. These communities are from 3.7–4.5 km from the Blåisen glacial outlet of the Hardangerjøkulen glacier. These three communities are situated in lee-sides in early melting snow beds with a humus rich podsol soil in the low alpine zone, and are characteristic for plant communities in alpine Norway in sites between windblown ridges and late melting snow beds. The two most climatically severe communities (D and E) are situated on north-facing slopes ca. 0.9 km from the Blåisen glacial outlet at ca. 1300 m (D) and 1340 m (E) elevation. These two communities occur in the middle-alpine zone where typical lee-zone plant communities are scarce. In these communities the soil and vegetation cover is substantially less developed than in the three other communities. Although we did not measure weather conditions directly, there are several features indicating that the two communities on the north-facing slopes are exposed to the most severe environmental conditions. First, solar insulation is lower on the north-facing compared to the south-facing slope. Second, cold glacial wind reaches the two communities close to the glacier but not the three other communities. Third, a preliminary analysis of trends in species composition reveals that the rank of the communities is in the expected order along the first DCA axis (, Appendix 1). This analysis also provides a crude description of the communities within the study area (see CitationElven (1978) for a further description of plant communities in the glacial forelands of Blåisen).
FIELD WORK
Within each of the five study communities, measuring ca. 150 × 150 m, we randomly selected 20 willow shrubs of at least ca 1 × 1 m in size. The selected shrubs were at least 5 m apart. Each shrub was divided into a north and south section. To examine if the presence of a canopy affects species richness and composition of vascular plant species, we randomly positioned two quadrats (25 × 25 cm) within each section: one inside and one ca. 50 cm outside the canopy. These quadrats were further divided into four 12.5 × 12.5 cm subquadrats. The abundance of vascular plant species was measured inside each of the subquadrats on a scale from 0 to 3, where 0 = absence, 1 = low abundance (the species cover < ca. 10 % of the subquadrat), 2 = medium abundance (the species cover ca. from 10–50 % of the subquadrat), 3 = high abundance (the species cover > 50% of the subquadrat). Adding the abundance values from each of the four sub-quadrats yield an abundance measure of 0 to 12 for each species (see Appendix 1). To examine if properties of individual canopies have an effect on the magnitude of difference in species composition and richness, we measured photosynthetic active radiation (PAR) with a LI190SA quantum sensor (Li-Cor) immediately above the ground-vegetation of each quadrate at the time of the species abundance measurements. On average, an individual canopy reduced PAR by 59.3% ± 18.1 (SD, n = 93) relative to immediately outside a canopy. We also estimated the size of each canopy by measuring its length in both east-west and north-south directions. On average, an individual canopy was 3.8 m2 ± 2.8 (SD, n = 100).
STATISTICAL ANALYSES
To examine if species richness differed between inside and outside canopies and between communities, we first included the inside/outside-canopy factor and community (A–E), and their interaction, in an initial two-factor ANOVA. This analysis showed a significant interaction between the canopy-factor and community on species richness (F 4,390 = 3.04, P = 0.02), indicating that differences in species richness inside and outside canopies differed between the five communities. Therefore, we continued analyzing the effects of canopies on species richness separately for each community. These models included canopy (inside, outside) as a fixed factor, block (the 20 individual canopies) as a random factor, and the north-south direction (north, south) as a random factor. Because these are mixed models, we used the mean square of the interaction between canopy and block in the denominator when calculating the F-ratio of the canopy effects (CitationZar, 1984).
To assess the influence of individual canopies on species richness and composition, the properties of each shrub were defined as the size of the canopies and the reduction in PAR inside the canopy compared to outside. We made a PAR-reduction index that accounts for PAR-measurements made on different days and at different times during a day with considerable differences in incoming PAR (e.g., the relative reduction in PAR by a canopy is larger on a clear day than on a cloudy day, ). The index was established by regressing relative reduction of PAR ([PARoutside − PARinside]/PARoutside), against PARoutside. The regression line is then supposed to account for the difference in light conditions during sampling. The residuals from this regression are taken as an estimate of the reduction of PAR by the canopy and is hereafter called “delta PAR.” We used these values to examine how the canopies affected species richness and composition through variation in delta PAR. The species richness response variables were found by subtracting species richness outside from species richness inside, separately for the south and north sides of each canopy. A Detrended Correspondence Analysis (DCA, Citationter Braak and Smilauer, 1998) was performed within each separate community to estimate the difference in species composition between inside and outside canopies. A unimodal method was preferred as the gradient length was above two SD units within each community (Citationter Braak and Verdonschot, 1995). The ordinations were performed with default settings in CANOCO 4 for Windows (Citationter Braak and Smilauer, 1998). We assessed the difference in species composition between inside and outside a canopy (separately for the north and south directions) by the distance between the two samples scores along the first four axes (hereafter called delta sample scores). To secure robustness of interpretation of this particular analysis we ranked the delta sample scores prior to statistical testing. We used linear regression to examine the impacts of delta PAR and canopy size (predictors) on differences in species richness and composition between the outside and inside of the canopies.
We made a direct analysis on the difference in species composition between outside and inside the canopies by a Canonical Variate Analysis (CVA, Citationter Braak and Smilauer, 1998) to evaluate the significance of the trend in species composition inside and outside the shrub-canopies by a constrained Monte Carlo permutation test. The constrained permutation accounts for the split-plot design in the data, and each shrub consists of 4 samples (2 outside and 2 inside). The randomizations were allowed only within these four samples (Citationter Braak and Smilauer, 1998); hence the variation between shrubs did not therefore influence the statistical tests. We also performed a CVA using present/absent data to examine if the results obtained were influenced by our subjective abundance measurements. These analyses yielded similar interpretations as the analyses based on the abundance data, and therefore we only present the results based on the abundance data.
Results
DIFFERENCES IN SPECIES RICHNESS BETWEEN INSIDE AND OUTSIDE CANOPIES
Species richness is significantly different between inside and outside canopies in community B (). The effects of canopies are subtler in communities A and C, where there is a significant (community A), or marginal significant (community C), interaction between the canopy effect and the block effect (), showing that the effect of canopies on species richness differs between shrubs within these communities. Species richness does not differ significantly inside and outside canopies in communities D and E (). There is a weak trend where species richness is higher outside canopies in communities A and B (with the most benign environmental conditions), whereas differences in species richness between inside and outside canopies are very small in C, D, and E ().
DIFFERENCES IN SPECIES COMPOSITION BETWEEN INSIDE AND OUTSIDE CANOPIES
Species composition differs significantly inside and outside canopies in all communities (). Inspection of the P-values suggests that differences in species composition inside and outside the canopies are greater in the benign communities (A–C) than in the severe communities (D–E). Appendix 1 shows the mean abundance of species inside and outside the canopies in each of the five communities.
IMPACT OF CANOPY PAR REDUCTION AND CANOPY SIZE ON SPECIES RICHNESS
Simple linear regression shows that there is a significant positive relationship between differences in species richness outside and inside canopies and delta PAR of canopies in community A (, ), showing that in this community, canopies that reduce PAR to a large extent reduce species richness to a greater extent than more open canopies. PAR reduction of canopies has no impact on species richness in any of the other communities ().
The size of canopies has no effect on the difference in species richness inside and outside canopies in any community ().
IMPACT OF PAR REDUCTION AND CANOPY SIZE ON SPECIES COMPOSITION
The difference in species composition outside and inside individual canopies is significantly related to delta PAR of canopies in community C (, ). Thus, in this community canopies that reduce PAR to a great extent have a larger difference in species composition inside and outside canopies, compared to canopies that reduce PAR to a smaller extent.
The size of individual canopies is significantly related to the difference in species composition inside and outside canopies in community C (, ), and marginally significant in community A (). Large canopies in these communities have greater differences in species composition outside and inside canopies than smaller canopies. In community C, canopy size and PAR reduction are significantly positively correlated (r pearson = 0.36, P = 0.02) and although the correlation is not particularly high, it is difficult to tease apart the separate effect of these two variables on the difference in species composition outside and inside canopies in this community. Canopy size and PAR reduction are not significantly correlated in any other community.
Discussion
Species richness was significantly lower inside canopies in one of the most environmentally benign communities (B), whereas willow canopies had a variable effect on species richness in the two other benign communities (A and C). The fact that there were no canopy effect on species richness in the two communities (D and E) situated in more severe environmental conditions suggest that our result only partly agrees with previous experimental results where canopies have facilitated establishment, growth, and reproduction of individual plants in relative harsh environments (e.g., CitationBertness and Callaway, 1994; CitationCallaway, 1994; CitationHacker and Gaines, 1997; CitationHolmgren et al., 1997). Moreover, the actual reduction in species richness inside canopies in the two most benign communities is rather small, indicating that competitive exclusion caused by canopies is modest in these communities.
While species richness appears to be relatively little affected by canopies, species composition is highly significantly different inside and outside canopies in the two most benign communities, showing that the presence of a canopy actually does have an effect on which species are growing below it. Thus, a willow canopy produces a significant (and large) species turnover over a distance of only ca. 1 to 2 m, suggesting that willow canopies have impacts on the population dynamics of individual species that translates into a small-scale spatial pattern of species composition across the mosaic of willow canopies within a community. The lower species richness inside canopies in A and B indicate that the competitive impacts of canopies may be most important in producing differences in species composition inside and outside canopies in these communities. Based on the large difference in species composition, which takes into account abundances of individual species, between inside and outside canopies, and the relatively small differences in species richness, our results suggest that the effects of canopies are highly species-specific and that generalizations about canopy effects at the community level, based on a few species, would be risky. Moreover, from Appendix 1 it is evident that some species have a higher abundance inside while other species have a higher abundance outside canopies. Nevertheless, it appears that more species are negatively than positively affected in the two most benign communities, whereas in the three other communities, negative and positive effects appear to cancel each other out at the community level.
Willow canopies in our study area greatly reduce the availability of light for plants (CitationTotland and Esaete, 2002), which may be a considerable stress factor for alpine species (CitationKörner, 1999; CitationGrytnes, 2000). However, despite this, in the most environmentally severe communities, this light reduction does not negatively affect species richness. Thus, other environmental factors that are changed by canopies likely positively affect establishment, growth and survival of most species. In particular, we believe that increased soil organic content, through higher litter accumulation below canopies, may have an important positive effect (see CitationTotland and Esaete, 2002) that counteracts negative effects, in particularly in the most severe communities close to the glacier. There, the vegetation cover, and thus also soil organic content, is considerably lower than farther away from the glacier. Consequently, the relative difference in soil organic content, nutrient availability, and water content may be greater between inside and outside canopies in communities D and E, compared to the others. In addition, a shelter effect by canopies may reduce wind (e.g., CitationValiente-Banuet and Ezcurra, 1991) and herbivory-related (e.g., CitationMcAuliffe, 1988; CitationLevine, 2000) stress and thereby contribute to reduce any negative effects of reduced PAR on plant persistence below canopies.
Surprisingly, we found few indications of differential impacts of canopies on species richness and composition, based on variation in canopy PAR reduction or size within the communities. PAR reduction of individual canopies is related to species richness only in community A, whereas differences in species composition were related to PAR reduction or canopy size only in community C. An explanation for the impact of canopy PAR reduction on species richness or composition is straightforward; the greater the PAR reduction, the fewer species would be able to germinate, establish, grow, and survive because most alpine species require a high availability of PAR (CitationKörner, 1999). The lack of a relationship between PAR reduction and canopy size and species richness and composition in the other communities may be due to a small variation in the two environmental variables within the communities. In addition, when the canopies have reached a certain size, the differences in environmental factors imposed by a canopy, such as PAR, soil moisture, and nutrient availability, may be so small that differences in the canopy effect experienced by plants may be minor.
Abiotic environmental factors may be far more important for species richness and composition of plant community than interspecific interactions (e.g., CitationGough et al., 1994; CitationJumpponen et al., 1999). Our results certainly agree; the differences in species richness and composition are substantially greater among communities situated in contrasting abiotic environments than between plots situated inside and outside canopies within the same community. In our system, differences in current abiotic conditions are probably not the only reason for the great difference in community parameters. The communities are situated along a chronosequence of glacier retreat both since the last glaciation, ca. 9000 yr ago, and also since the Little Ice Age, ca. 300 yr ago (CitationNesje and Dahl, 1991). Several authors have found positive associations between plants in primary succession systems, such as glacier forelands (e.g., CitationDale et al., 1991; CitationMatthews, 1992; CitationBlundon et al., 1993; CitationChapin et al., 1994; CitationVetaas, 1994; CitationFastie, 1995). Our results of no net positive or negative effect of willow shrubs on community richness is consistent with the results of CitationJumpponen et al. (1998). They found that canopies of Salix commutata and S. phylicifolia on a glacier foreland in Washington, U.S.A. had no effect on species richness. Thus, their results and ours suggest that nucleation in successional systems may not always occur, and may vary among nucleation species.
Our results suggest that up-scaling from results based on few species to a whole-community scale may not be straightforward for two main reasons. First, responses of a few studied species may not be representative of patterns occurring at the whole-community level, due to the highly species-specific responses to interactions. Second, impacts of a canopy species on the occurrence and richness of others may vary both in strengths and directions (negative, positive, neutral) along environmental gradients.
FIGURE 1. Map showing the location of the five studied plant communities (A–E) within the Finse valley. “Blåisen” indicated the situation of the Blåisen glacier outlet. Solid thick line is the Bergen-Oslo railroad. Contour interval is 20 m. Scale is indicated in the lower right corner. Small map indicate location of Finse in Norway
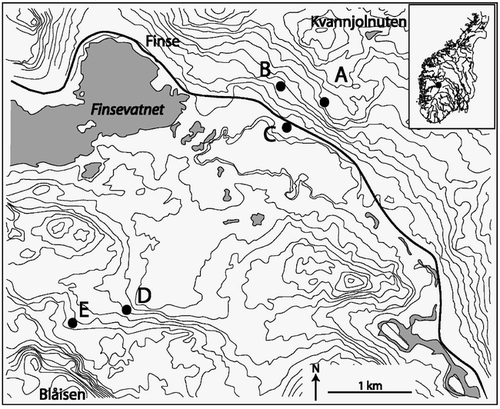
FIGURE 2. Plot of species scores (most common species shown) along the first and second ordination axes from a detrended correspondence analysis (DCA) including all samples (default settings, Canoco 4.0). The centroids of the site scores within each community show that the communities appear in order along the first axis from the most benign community (A) with the lowest score along the first axis to the most severe community (E) with the highest score along the first DCA-axis (inserted figure). Eigenvalues of the two first axes are 0.560 and 0.321, respectively
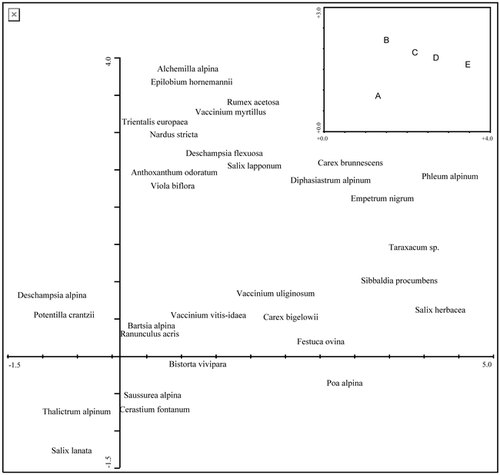
FIGURE 3. Relationship between photosynthetically active radiation (PAR) outside Salix lapponum canopies and the percentage reduction in PAR below the canopy (PARoutside − PARinside)/PARoutside) at Finse in 1998. The residuals of this regression are used as a standardized measure of PAR reduction. These standardized PAR reduction values (delta PAR) take into account that PAR measurements for different inside/outside pairs were done under different solar radiation regimes
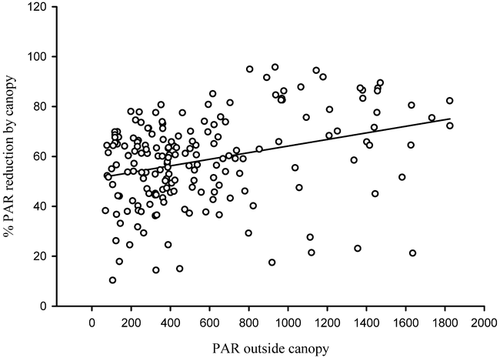
FIGURE 4. Bar graphs showing the mean and standard error (vertical lines) of species richness per plot (25 cm × 25 cm outside and inside Salix lapponum canopies in five communities (A–E) situated along a climate severity gradient at Finse in 1998
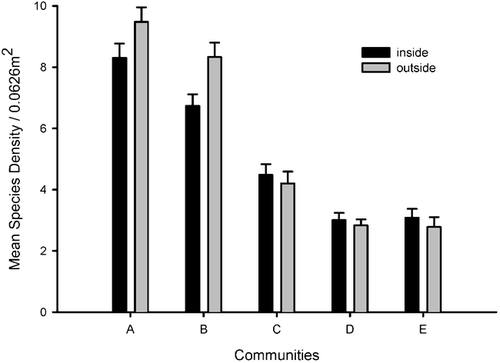
FIGURE 5. Relationship between (A) delta PAR (photosynthetically active radiation) of canopies and the difference in species richness from outside to inside Salix lapponum canopies at Finse in 1998 in community A, (B) between delta PAR and the ranked difference in sample scores from a Detrended Correspondence Analysis (DCA) between outside and inside canopies in community C, and (C) between canopy size and the ranked difference in sample scores in community C
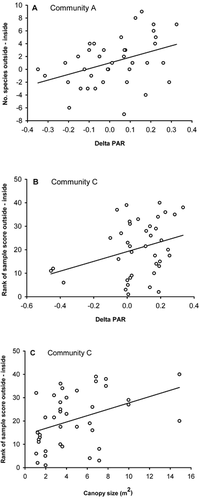
TABLE 1 F-ratios and P-values from two-factor ANOVA on the effect of canopy (inside vs. outside, fixed factor) and block (individual canopies, random factor), and their interaction, and a factor separating the north- and south-facing parts of a Salix lapponum canopy (north-south, random factor) on speicies richness within five communities at Finse in 1998. F = F-ratio, P = significance level. Degrees of freedom for the canopy effect are 1, 19 (denominator, numerator), and 19, 39 for the blocvk effect and for gthe interaction between canopy and block, and 1, 39 for the north-south effect
TABLE 2 Summary of species composition differences between inside and outside Salix lapponum canopies (Canonical Variate Analysis with inside/outside as environmental variable), as tested by a split-plot constrained Monte Carlo permutation test
TABLE 3 Standardized regression coefficients and significance values (in parentheses, bold values are significant at the 0.05 level) of simple linear regressions between difference in species richness inside and outside canopies (special richness), and difference in species composition inside and outside canopies (species composition) and canopy size and delta photosynthetically active radiation (PAR) (predictors) in each of five communities (A–E) at Finse in 1998. Delta PAR measures the standardized amount of reduction in PAR by Salix lapponum canopies. The value is the residuals of a regression between PAR outside (predictor) and percentage difference in PAR between inside and outside canopies. This standardizes for measurements of PAR made at different dates and at different times during the day. Species composition is represented as the ranked differences in site scores (distance along the first four axes of a detrended correspondence analysis (DCA) between inside and outside canopies. The direction (positive/negative) is not relevant for the actual difference in species composition between inside and outside canopies. Sample sizes are 26 in the regression between delta PAR and responses in community B, and 40 in all the other regressions
Acknowledgments
We thank Line Rosef for fieldwork assistance, the Research Council of Norway for financial support, the Alpine Research Centre at Finse for living facilities, and Beate H. Ingvartsen for drawing the map. John Birks, Mikael Ohlson, Ole Reidar Vetaas, and Rune H. Økland gave very useful comments on the manuscript.
References Cited
- Alstad, G. and O. R. Vetass . 1994. The influence of Acacia tortilis stands on soil properties in arid north-eastern Sudan. Acta Oecologica 15:449–460.
- Aune, B. 1993. Air temperature normals, normal period 1961–1990. The Norwegian Meteorological Institute, Oslo, Norway.
- Bengtsson, J. , T. Fagerström , and H. Rydin . 1994. Competition and coexistence in plant communities. Trends in Ecology and Evolution 9:246–250.
- Bertness, M. D. and R. M. Callaway . 1994. Positive interactions in communities. Trends in Ecology and Evolution 9:191–193.
- Bertness, M. D. and S. D. Hacker . 1994. Physical stress and positive associations among marsh plants. American Naturalist 144:363–372.
- Blundon, D. J. , D. A. MacIssac , and M. R T. Dale . 1993. Nucleation during primary succession in the Canadian Rockies. Canadian Journal of Botany 71:1093–1096.
- Callaway, R. M. 1994. Facilitation and interfering effects of Arthrocnemum subterminale on winter annuals in a California salt marsh. Ecology 75:681–686.
- Callaway, R. M. 1995. Positive interactions among plants. Botanical Review 61:306–349.
- Callaway, R. M. and L. R. Walker . 1997. Competition and facilitation: a synthetic approach to interactions in plant communities. Ecology 78:1958–1965.
- Carlsson, B. A. and T. V. Callaghan . 1991. Positive plant interactions in tundra vegetation and the importance of shelter. Journal of Ecology 79:973–983.
- Chapin, F. S. , L. R. Walker , C. I. Fastie , and L. C. Sharman . 1994. Mechanisms of primary succession following deglaciation at Glacier Bay, Alaska. Ecological Monographs 64:149–175.
- Clements, F. E. 1916. Plant succession: an analysis of the development of vegetation. Carnegie Institution of Washington, Washington, D.C 242:1–512.
- Connell, J. H. 1983. Interpreting the result of field experiments: effects of indirect interactions. Oikos 41:290–291.
- Dale, M. R T. , D. J. Blundon , D. A. MacIsaac , and A. G. Thomas . 1991. Multiple species effects and spatial autocorrelation in detecting species associations. Journal of Vegetation Science 2:635–642.
- Elven, R. 1978. Association analysis of moraine vegetation at the glacier Hardangerjøkulen, Finse, South Norway. Norwegian Journal of Botany 25:171–191.
- Fastie, C. L. 1995. Causes and ecosystem consequences of multiple pathways of primary succession at Glacier Bay, Alaska. Ecology 76:1899–1916.
- Førland, E. J. 1993. Precipitation normals, normal period 1961–1990. The Norwegian Meteorological Institute, Oslo, Norway.
- Gough, L. , J. B. Grace , and K. L. Taylor . 1994. The relationship between species richness and community biomass: the importance of environmental variables. Oikos 70:271–279.
- Grime, J. P. 1979. Plant Strategies and Vegetation Processes. New York: John Wiley. 222 pp.
- Grytnes, J. A. 2000. Fine-scale vascular plant species richness in different alpine vegetation types: relationship with biomass and cover. Journal of Vegetation Science 11:87–92.
- Gutiérrez, J. R. , P. L. Meserve , L. Contreras , C. H. Vásquez , and F. M. Jaksic . 1993. Spatial distribution of soil nutrients and ephemeral plants underneath and outside the canopy of Porlieria chilensis shrubs (Zigophyllaceae) in arid costal Chile. Oecologia 95:347–352.
- Hacker, S. D. and S. D. Gaines . 1997. Some implications of direct positive interactions for community species diversity. Ecology 78:1990–2003.
- Holmgren, M. , M. Scheffer , and M. A. Huston . 1997. The interplay of facilitation and competition in plant communities. Ecology 78:1966–1975.
- Joffre, R. and S. Rambal . 1993. How tree cover influences the water balance of Mediterranean rangelands. Ecology 74:570–598.
- Jumpponen, A. , K. Mattson , J. M. Trappe , and R. Ohtonen . 1998. Effects of established willows on primary succession on Lyman Glacier forefront, North Cascade Range, Washington, U.S.A.: evidence for simultaneous canopy inhibition and facilitation. Arctic and Alpine Research 30:31–39.
- Jumpponen, A. , H. Vare , K. G. Mattson , R. Ohtonen , and J. M. Trappe . 1999. Characterization of ‘safe sites’ for pioneers in primary succession on recently deglaciated terrain. Journal of Ecology 87:98–105.
- Keddy, P. A. 1989. Competition . New York: Chapman and Hall. 202 pp.
- Körner, C. 1999. Alpine Plant Life. Berlin: Springer-Verlag. 338 pp.
- Levine, J. M. 2000. Complex interactions in a streamside plant community. Ecology 81:3431–3444.
- MacArthur, R. H. 1972. Geographical Ecology, Patterns in the Distribution of Species. New York: Harper and Row. 269 pp.
- Matthews, J. A. 1992. The Ecology of Recently Deglaciated Terrain: A Geoecological Approach to Glacier Forelands and Primary Succession. Cambridge: Cambridge University Press. 386 pp.
- McAuliffe, J. R. 1988. Marcovian dynamics of simple and complex desert plant-communities. American Naturalist 131:459–490.
- Nesje, A. and S. O. Dahl . 1991. Holocene glacier variations of Blåisen, Hardangerjøkulen, Central Southern Norway. Quarternary Research 35:25–40.
- Niering, W. A. , R. H. Whittaker , and C. H. Lowe . 1963. The saguaro: a population in relation to environment. Science 142:15–23.
- Pugnaire, F. I. and P. Haase . 1996. Facilitation between higher plant species in a semiarid environment. Ecology 77:1420–1426.
- ter Braak, C. J F. and P. Smilauer . 1998. CANOCO reference manual and user's guide to Canoco for Windows: Software for canonical community ordination (version 4). Microcomputer Power, Ithaca, New York, USA.
- ter Braak, C. J F. and P. F M. Verdonschot . 1995. Canonical correspondence analysis and related multivariate methods in aquatic ecology. Aquatic Sciences 57:255–289.
- Totland, Ø and J. Esaete . 2002. Effects of willow canopies on species performance in a low-alpine community. Plant Ecology 161:157–166.
- Valiente-Banuet, A. and E. Ezcurra . 1991. Shade as a cause of the association between the cactus Neobuxbaumia tetetzo and the nurse plant Mimosa luisana in the Tehuacán valley, Mexico. Journal of Ecology 79:961–971.
- Vetaas, O. R. 1994. Primary succession of plant assemblages on a glacier foreland—Bødalsbreen, Southern Norway. Journal of Biogeography 21:297–308.
- Wilson, J. W. and O. D Q. Agnew . 1992. Positive-feedback switches in plant communities. Advances in Ecological Research 20:265–336.
- Zar, J. H. 1984. Biostatistical Analysis. 2nd. ed. Englewood Cliffs, NJ.: Prentice Hall. 718 pp.
APPENDIX 1
The mean abundance (mean of two 0.25 × 0.25 quadrats averaged over 20 canopies) of species inside and outside Salix lapponum canopies in five communities at Finse in 1998. Abundance is expressed on a scale from 0 to 12 based on visual estimates. Open cells for a species denotes that the species did not occur in that community. 0 for either inside or outside indicates that the species was present in the community but did not occur either inside or outside canopies
APPENDIX 1 (Cont)