Abstract
Osmotic minipumps represent a convenient and established method for targeted delivery of agents into the brain of small rodents. Agents unable to cross the blood brain barrier can be directly infused into the brain parenchyma or lateral ventricle through implanted cannulas. The small volume of the minipump reservoir typically limits the infusion time to 4–6 weeks. Pump changes with reattachment of a new pump reservoir to the cannula might lead to brain tissue irritation or increased intracranial pressure associated with hydrocephalus. Here, we describe a pump reservoir exchange technique using a Y-shaped connection piece (Y-con) between the infusion cannula and the pump reservoir. This allows repeated replacement of a subcutaneously installed pump reservoir for brain delivery of agents in mice. Experimental evaluation of Y-con pump replacement revealed no signs of tissue irritation or hydrocephalus and allowed extended controlled delivery of infusion agents in the brain.
Osmotic minipumps represent an established technique for targeted delivery of agents into the brain of small animals. Thus, agents unable to cross the blood brain barrier can be directly infused in the brain parenchyma or into the ventricles through implanted cannulas. We describe a pump reservoir exchange technique allowing repeated replacements of a subcutaneously installed pump reservoir for brain delivery of agents in mice.
The invention of Alzet osmotic pumps in the 1970s allowed constant, reproducible, and long-term delivery of drugs, peptides, and antibodies to small laboratory rodents (Citation1, Citation2). Subcutaneously implanted osmotic pumps reduce animal stress and diminish the risk of infections, compared with repeated acute injections. Subsequent advances in the development of targeted delivery using osmotic minipumps provided broader treatment implementations (Citation3) and brain infusion devices that circumvent the blood brain barrier (Citation4–6). In so-called Brain Infusion Kits, a subcutaneously placed osmotic minipump is connected with a plastic tube to an implanted stainless-steel cannula that delivers the agent to the brain parenchyma or the ventricle.
While the surgical replacement of subcutaneously placed osmotic pumps has been described (Citation7), no established technique is available to exchange pump reservoirs connected to an intracerebral implanted cannula. This limitation shortens the duration of intracerebral infusions, especially in laboratory mice, which can only carry relatively small subcutaneous pump reservoirs due to their small body size. An extended infusion period would be of great advantage for various intracerebral applications in laboratory mice.
Direct replacement of the pump reservoir displaces the liquid volume in the tubing during reservoir reattachment and could result in increased agent release and tissue pressure at the injection site. Intracerebroventricular (i.c.v.) infusions have been reported to cause increased intracerebral pressure and associated brain deformation (Citation8). To circumvent these putative adverse effects, we have developed a novel Y-shaped connection piece (Y-con) between the minipump and the cannula. The Y-con features a pressure release exit channel () that allows excessive liquid to leave the device during a pump change, preventing increased tissue pressure.
The Y-connection piece (Y-con) allows exchange of the pump reservoir of Alzet osmotic pumps by providing a pressure release exit. (A) Plain view of the Y-con. The Y-con consists of a central round plate and contains 3 adjusted channels. The two 4 mm long channels allow attachment of the new pump reservoir (bottom channel) and re-connection to the tubing that reaches the CNS cannula (top channel). The third 1.4 mm short channel (pressure release exit) enables equilibration of liquid pressure during surgery. (B) Scheme of the Y-con design, including length and angle details.
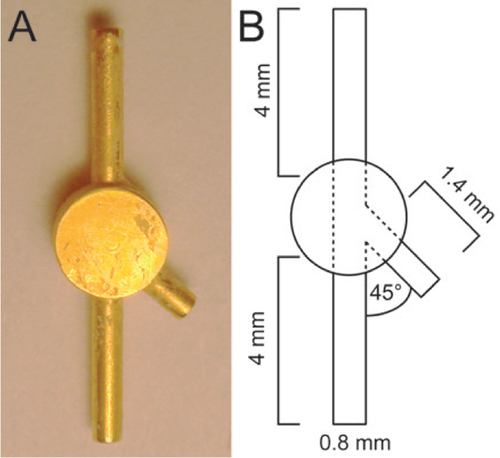
To make the Y-con, a circular brass bar with a diameter of 2.5 mm was used (Hardware store HORNBACH-Baumarkt-AG, Tübingen, Germany). A longitudinal hole and a second connecting hole at a 45° angle from the first were drilled with a diameter of 0.78 mm at a distance of 0.2 mm from the end of the brass bar (Drill beads: Zecha GmbH, Konigsbach-Stein, Germany; Drill: Bergeon Swiss Tools, Le Locle, Switzerland). Inching of a 1.2 mm thick cross-sectional slice of the brass bar (0.2 mm distance from both sides of the holes) was performed with a turning machine at a precision of 3 µm (Bergeon Swiss Tools, Le Locle, Switzerland). An outer channel diameter of 0.8 mm was chosen to fit the tubing provided in the Alzet Brain Infusion Kits (Durect Corperation, Cupertino, CA). The channel, which is slightly larger in diameter than the hole, is applied to the hole with a certain amount of force to fix it to the relatively soft brass. The metal channels from the Brain infusion Kits were attached to the brass slice (about 0.5 mm deep), then fixed mechanically and with glue (UHU GmbH & Co. KG, Buehl/ Baden, Germany). This allows a constant flow rate and facilitates firm attachment to the tubing (). The 2 opposing 4 mm long channels connect to the tubing that reaches the pump reservoir and the brain infusion cannula. The shorter 1.4 mm long channel provides pressure release by enabling liquid displacement during pump reservoir replacement. The reduced length in combination with the 45° angle of this channel allows stable closure of the pressure release exit using dental cement (). This closure is achieved by subsequent sealing of the pressure release exit and filling of the space between the two channels by applying additional dental cement, ensuring free animal movement after the first pump reservoir replacement. Potential tissue irritation is minimized by the lack of sharp edges or notches and by installing the Y-con channels parallel to the skin during surgery. To avoid metal dissociation, oxidation, and copper-dependent Fenton reactions, the Y-con received a >5 µm thick gold coating (Puramet 202 finegold bath, Doduco GmbH, Pforzheim, Germany) (Citation9, Citation10).
To evaluate the Y-con based osmotic pump change technique for brain delivery, we used male hemizygous CD11b- HSVTK (TK) mice (Citation11) expressing a herpes simplex virus thymidine kinase under the monocytic CD11b promoter. Mice were kept under pathogen-free conditions and experiments were approved by the local authorities. Intracerebroventricular application of the thymidine-analog Ganciclovir (GCV) via Alzet osmotic minipumps to these mice (see also Supplementary Material), resulted in an almost complete microglia ablation in the neocortex within a two week timeframe, while PBS control application did not affect TK mice (Citation12). Using this treatment paradigm, we showed that application of PBS into TK mice via minipumps for two weeks and subsequent Y-con dependent reservoir change to GCV resulted in microglia depletion highly comparable to the direct GCV application for two weeks via minipumps, without any deleterious side effects.
In the first group of mice, PBS was infused i.c.v. via minipumps for two weeks and the animals were subsequently sacrificed (PBS group). In a second group, GCV was infused i.c.v. via minipumps for two weeks and sacrificed (GCV group). A third group of mice received an i.c.v. PBS infusion for two weeks, followed by pump reservoir replacement and GCV infusion for another two weeks (PBS + GCV group) (). Fourteen days after implantation of the osmotic minipump, a small incision was made in the skin to access the subcutaneous pump reservoir. The tubing was cut close to the pump reservoir, which was subsequently removed, and the new reservoir with an attached Y-con was placed in the subcutaneous pocket. The opposing channel of the Y-con was inserted into the tube connected to the CNS. After equilibration of liquid pressure, the pressure release exit was sealed with dental cement. (Further pump reservoir replacements can be achieved by removing the dental cement at the pressure release exit and resealing after pump exchange.) Finally, the cut was sutured, positioning the pressure release exit parallel to the skin to avoid tissue irritation.
Y-con based pump reservoir exchange (first PBS, then GCV) and direct application of GCV lead to similar Iba1+ microglia ablation in the neocortex of TK mice. Three month old male TK mice received two weeks i.c.v. treatment of PBS, GCV, or PBS followed by two weeks GCV application (PBS + GCV) via pump exchange. (A) Direct i.c.v. infusion (left and middle panel) and pump reservoir replacement (right panel) using the Y-con, did not result in alterations of the lateral ventricle volume and revealed no tissue irritation. Calibration bar 2000 µm. (B) Iba1 immunohistochemistry in the neocortex of PBS-treated TK mice indicates a normal number of microglia cells (left panel), while direct application of GCV and treatment with PBS prior to GCV (PBS + GCV) via pump reservoir replacement resulted in almost complete microglia ablation (middle and right panel). Calibration bar: 100 µm. (C) Quantitative stereological analysis of Iba1+ cell number in the neocortex reveals a >90% ablation of microglia in TK mice after 2 weeks GCV treatment in comparison to the PBS treated control group (bars indicate the mean ± SEM; n = 3 mice/group). ANOVA indicates a significant difference between the three treatment groups (F(2,8) = 406.48; ***P < 0.001). Tukey HSD post-hoc analysis displays significant differences between the PBS and GCV treated TK mice.
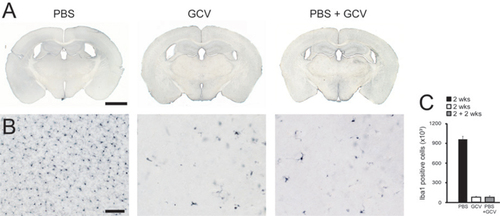
Analysis of the total number of neocortical microglia of PBS treated TK mice revealed the characteristic number of Iba1+ cells (Citation13), while both GCV alone and PBS + GCV treated TK mice displayed >90% microglia depletion. Therefore, pump reservoir exchange did not influence GCV treatment efficacy and resulted in similar levels of microglia cell ablation compared with the direct GCV application ().
To ensure the safety of pump reservoir exchanges utilizing the Y-con, ventricle volumes were compared in GCV and PBS + GCV mice. No increase in lateral ventricle volume was observed in mice in which the pump reservoir change has been performed () or in mice receiving single pump implantations and agent application for up to four weeks (Citation12, Citation14). In contrast, pump replacement without the Y-con can cause hydrocephalus and tissue damage including local gliosis ().
(A) PBS application (2 weeks) into the ventricle of 3 month old TK mice does not alter the size of the lateral ventricle system (n = 3). (B) Pump reservoir replacement applying direct reconnection of the tubing to the brain cannula (after initial 2 weeks PBS application) results in hydrocephalus in 3 month old TK mice 2 weeks after pump reservoir exchange (n = 3). Calibration bar: 1000 µm. (C) Quantitative stereological analysis of the ratio of lateral ventricle volume to total brain volume reveals a 2-fold increase (mean ± SEM; n = 3 mice/group); t-test indicates a significant difference between the groups (not replaced pump (nrp) and directly replaced pump (drp); t = 13.28, df = 4, ***P < 0.001).
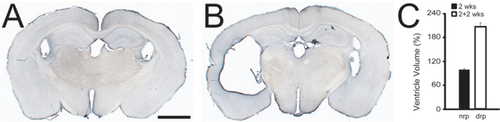
Application of agents directly to the CNS via Alzet osmotic pumps is limited by volume, flow rate, and size of the implanted pump reservoir. Thus, prolonged application requires exchange of the osmotic pump reservoir since it cannot be refilled or reused. An alternative approach to pump reservoir exchange is use of a refillable motorized pump (Citation15). However, the size and weight of these electronically controlled devices preclude their use in small animal models. Furthermore, motorized pumps are less cost-efficient in comparison with osmotic pumps and also require intensive cleaning and sterilization before they can be reused.
Alzet osmotic pumps of fer a continuous application of agents for up to six weeks. Reservoir exchange extends the delivery duration but bears the risk of liquid displacement within the tubing. Since mice have small brain structures and low ventricle volumes (approximately 10 mm3), the resulting increased parenchymal or ventricular pressure might not be compensated (Citation16) and could induce tissue extrusion or hydrocephalus. We have shown in the present study that such increased pressure can be avoided by replacing the pump with an attached Y-con. The key to the function of the Y-con is the third channel, which provides a pressure release exit and drainage for excessive liquid arising during tubing reconnection. In addition, the construction of the Y-con ensures free animal movement and minimal tissue irritation. Coating the Y-con with gold protects the surrounding tissue from brass or metal derived oxidative stress (Citation9, Citation10). Furthermore, brass and gold are not ferromagnetic and do not interfere with surgical materials or surgical instruments. The Y-con can be manufactured easily and inexpensively. Because it is composed of metal, the Y-con can be autoclaved, treated with organic solvents for sterilization, and reused repeatedly.
Our novel Y-con approach offers a safe option for replaceing Alzet osmotic pump reservoirs and extending treatment duration in small rodents while avoiding increased intracranial pressure and subsequent tissue irritation.
Author contributions
S.A.G. developed the Y-con, designed the experiments, and performed the analysis. Manuscript preparation was carried out by S.A.G. and M.J.
Competing interests
The authors declare no competing interests.
Supplementary Material For: Replacement of osmotic minipumps to extend the intracerebral infusion time of compounds into the mouse brain
Download PDF (68.4 KB)Acknowledgments
We thank W. Dennig for his support in building the Y-con piece and A. Aguzzi for scientific advice. The CD11b- HSVTK mice were provided by A. Aguzzi and F. Heppner (Zürich and Berlin, respectively). Ganciclovir was generously provided by Roche Bioscience (Palo Alto, California, USA). This work was supported by grants from the German Competence Network on Degenerative Dementias (BMBF-01GI0705) and the German National Genome Network (BMBF-NGFNPlus).
Supplementary data
To view the supplementary data that accompany this paper please visit the journal website at: www.tandfonline.com/doi/suppl/10.2144/000114064
Additional information
Funding
References
- Yates, F.E., H.Benson, R.Buckles, J.Urquhart, and A.Zaffaroni. 1975. Engineering development of therapeutic systems: a new class of dosage forms for the controlled delivery of drugs.Adv Biomed Eng.5:1–34.
- Theeuwes, F. and S.I.Yum. 1976. Principles of the design and operation of generic osmotic pumps for the delivery of semisolid or liquid drug formulations.Ann. Biomed. Eng.4:343–353.
- de la Torre, J.C. and M.Gonzalez-Carvajal. 1981. Steady state drug or fluid delivery to injured or transected spinal cord of rats.Lab. Anim. Sci.31:701–703.
- Pettigrew, J.D. and T.Kasamatsu. 1978. Local perfusion of noradrenaline maintains visual cortical plasticity.Nature271:761–763.
- Williams, L.R., H.L.Vahlsing, T.Lindamood, S.Varon, F.H.Gage, and M.Manthorpe. 1987. A small-gauge cannula device for continuous infusion of exogenous agents into the brain.Exp. Neurol.95:743–754.
- Rothman, R.B., L.S.Brady, H.Xu, and J.B.Long. 1993. Chronic intracerebroventricular infusion of the antiopioid peptide, Phe-Leu-Phe-Gln-Pro-Gln-Arg-Phe-NH2 (NPFF), downregulates mu opioid binding sites in rat brain.Peptides14:1271–1277.
- Banach-Petrosky, W., W.J.Jessen, X.Ouyang, H.Gao, J.Rao, J.Quinn, B.J.Aronow, and C.Abate-Shen. 2007. Prolonged exposure to reduced levels of androgen accelerates prostate cancer progression in Nkx3.1; Pten mutant mice.Cancer Res.67:9089–9096.
- Tamagawa, M., H.Keino, M.Taniguchi, J.Morita, and I.Naruse. 1994. Induction of the hydrocephalus by the intraventricular injection of aurintricarboxylic acid in mice exo utero.Cong. Anom.34:345–352.
- Stohs, S.J. and D.Bagchi. 1995. Oxidative mechanisms in the toxicity of metal ions.Free Radic. Biol. Med.18:321–336.
- Valko, M., H.Morris, and M.T.Cronin. 2005. Metals, toxicity and oxidative stress.Curr. Med. Chem.12:1161–1208.
- Heppner, F.L., M.Greter, D.Marino, J.Falsig, G.Raivich, N.Hovelmeyer, A.Waisman, T.Rulicke, et al.. 2005. Experimental autoimmune encephalomyelitis repressed by microglial paralysis.Nat. Med.11:146–152.
- Grathwohl, S.A., R.E.Kalin, T.Bolmont, S.Prokop, G.Winkelmann, S.A.Kaeser, J.Odenthal, R.Radde, et al.. 2009. Formation and maintenance of Alzheimer's disease beta-amyloid plaques in the absence of microglia.Nat. Neurosci.12:1361–1363.
- Long, J.M., P.R.Mouton, M.Jucker, and D.K.Ingram. 1999. What counts in brain aging? Design-based stereological analysis of cell number.J. Gerontol. A Biol. Sci. Med. Sci.54:B407–B417.
- Varvel, N.H., S.A.Grathwohl, F.Baumann, C.Liebig, A.Bosch, B.Brawek, D.R.Thal, I.F.Charo, et al.. 2012. Microglial repopulation model reveals a robust homeostatic process for replacing CNS myeloid cells.Proc. Natl. Acad. Sci. USA109:18150–18155.
- Abe, C., T.Tashiro, K.Tanaka, R.Ogihara, and H.Morita. 2009. A novel type of implantable and programmable infusion pump for small laboratory animals.J. Pharmacol. Toxicol. Methods59:7–12.
- Jacobs, S., E.Ruusuvuori, S.T.Sipila, A.Haapanen, H.H.Damkier, I.Kurth, M.Hentschke, M.Schweizer, et al.. 2008. Mice with targeted Slc4a10 gene disruption have small brain ventricles and show reduced neuronal excitability.Proc. Natl. Acad. Sci. USA105:311–316.