Abstract
Library preparation is a crucial step in next-generation sequencing workflows. Key determinants of successful library preparation are the available amount of input DNA and the efficiency of the conversion of this DNA into functional library molecules. While the standard blunt-end ligation protocol for Ion Torrent libraries has a theoretical maximum efficiency of 25%, Y-adapters enable highly efficient library preparation by (i) sticky-end ligation and (ii) rendering both DNA strands functional for sequencing, hence resulting in a theoretical efficiency of up to 100%. Moreover, the generation of adapter dimers is reduced. Therefore, we designed, optimized and validated Y-adapters compatible with Ion Torrent sequencing. These facilitate higher library yields combined with overall high sequencing performance regarding the key characteristics read-length, base quality, and library complexity.
Method Summary
We developed, optimized, and validated Y-adapters for Ion Torrent sequencing, which are neither commercially available nor have been described elsewhere before. These Y-adapters enable highly efficient library preparation by (i) sticky-end ligation and (ii) rendering both DNA strands, hence theoretically up to 100% of the input DNA strands are functional for sequencing. Moreover, with low DNA input, the portion of adapter dimers is substantially reduced when using the described Y-adapters.
Graphical abstract
High-throughput sequencing is widely used for a variety of sequence-based analyses in different fields [Citation1–3]. Library preparation represents a decisive step in a sequencing workflow, and problems or limitations at this point can jeopardize the whole sequencing project as the available input material may be limited. Therefore, efficient conversion of genetic material into a sequenceable library is urgently necessary. Today, Illumina and Ion Torrent instrument families are dominating the market of second-generation sequencing platforms. Both platforms differ in the two key features of clonal library amplification and sequence detection [Citation4,Citation5]. Nevertheless, they share some characteristics of the shotgun DNA library preparation process: the necessity of DNA fragmentation to a defined fragment size range followed by fragment end polishing and the addition of platform-specific adapters by ligation [Citation6]. In both cases, each library fragment needs to have specific sequence features at the 5′- and the 3′-ends, respectively, in order to be functional (). In a generic Ion Torrent library preparation workflow, two different linear adapters (A and P1) are ligated onto the DNA fragments in order to incorporate the necessary functionalities for sequencing. In these A-P1 libraries, where A and P1 represent the two different linear adapters, statistically only 50% of the ligated sequence fragments carry the combination of A and P1 adapter that is obligatory for sequencing (A-P1). As a result, the sequence information stored in the other half of ligated fragments carrying identical adapters on both ends (A-A or P1-P1) is lost. In addition, only 25% of the total input DNA strands are sequenceable overall, since only one strand of the functional fragments (A-P1) will be clonally amplified. By contrast, generic sequencing on Illumina platforms is based on so-called Y-adapters. The advantage of these Y-shaped adapters is that one adapter comprises the necessary functionalities for both the 5′- and the 3′-ends. After ligation of this adapter to both fragment ends, both DNA strands are available for sequencing, yielding a higher proportion of functional molecules (up to 100% of the DNA strands). In addition, the Y-adapters are ligated in a sticky-end fashion, with a nontemplated A-overhang at the 3′-ends of the DNA fragments in combination with a T-overhang of the adapters. The higher efficiency of A-T cloning compared with blunt-end ligation has been shown before [Citation7]. Hence, the conversion of DNA fragments into library molecules is enhanced due to the advantages of ligating only one adapter together with the benefit of sticky-end ligation. Additionally, adapter dimer formation is significantly reduced compared with blunt-end ligation. Adapter dimers are undesirable, since they are quantified as part of the functional library and equally sequenced, although no information of the sample is included, thereby wasting sequencing capacity [Citation8].
Y-adapters are ligated in a sticky-end fashion to the fragment, thereby yielding library molecules, of which both strands fulfill the prerequisites for sequencing. By contrast, two different linear adapters that are ligated in a blunt-end mechanism beside the functional library molecule (A-P1) also create nonsequenceable molecules as byproducts (A-A, P1-P1), resulting in lower conversion of sample DNA to functional library. Additionally, blunt-end ligation increases the chances for the formation of unwanted adapter dimers and input fragment concatemers.
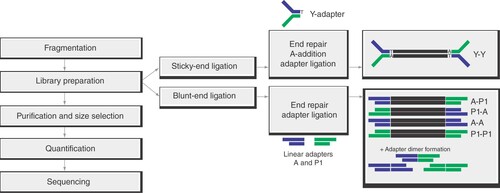
While for the Illumina platforms Y-adapters are the standard (as was the case for the discontinued 454 platform), there are no such adapters commercially available for the Ion Torrent platforms. Therefore, in order to take advantage of the enhanced library preparation enabled by the application of Y-adapters, here we set out to design and validate custom Y-adapters compatible with Ion Torrent platforms. Overall, the available information regarding the design of such adapters is scarce. To our knowledge, only one approach on the development and implementation of Y-adapters has been published, thereby focusing on adapters for the Roche 454 Titanium platform [Citation9]. While a step-by-step library preparation workflow was provided, no detailed comparative analyses regarding adapter design, ligation efficiency and sequencing parameters were included. Therefore, we investigated the influence of different parameters such as length and melting temperature of the double-stranded part as well as the number and distribution of phosphorothioate (PT) bonds in order to yield an optimal design. The functionality and performance of the adapters was assessed based on qPCR quantification of the libraries, sequence output, read length, and quality of the generated reads, as well as complexity of the obtained datasets, and compared with commercially available linear adapters.
Materials & methods
Preparation of Y-adapters
HPLC-purified or NGS-grade oligonucleotides (Supplementary Table S1) were ordered at metabion (Planegg/Steinkirchen, Germany) and dissolved in oligo buffer (1× TE, pH 8.0, 50 mM NaCl) at a concentration of 100 μM. For the assessment of different determinants of the Y-adapter performance, experiments were conducted with differing lengths of double-stranded regions, inosine nucleotides for shift in melting temperature and PT bonds at varying positions. For hybridization of the Y-adapter, 25 μl of the 100-μM stock solutions of the top and the matching bottom oligonucleotide were combined and filled with oligo buffer to a final volume of 100 μl to yield 25-μM adapters. The temperature profile for hybridization of the two oligonucleotides was as follows [Citation10]: 95°C for 1 min, followed by decreasing the temperature by 0.1°C per second to the 15°C final temperature, followed by an unlimited hold at 14°C. Finally, adapters were aliquoted and stored at −20°C for further use.
Library preparation & sequencing
The workflow from sample to sequencing was performed according to the previously published detailed protocol [Citation11], except for library preparation. In summary, DNA was extracted from overnight Escherichia coli DH10B cultures and mongoose tissue using a QIAamp DNA Mini Kit (Qiagen, Hilden, Germany) and from a tick cell line using the High Pure PCR Template Preparation Kit (Roche, Mannheim, Germany). Where not specified otherwise, 200–500 ng DNA was used as input material and fragmented with a Covaris M220 Focused-ultrasonicator™ (Covaris, Brighton, UK), aiming for a fragment size of 500–600 bp. For library preparation with Y-adapters, the GeneRead DNA Library I Core Kit (Qiagen) was deployed, in contrast to the established protocol. Following end repair and A-addition as per the manufacturer's instructions, 1 μl of 25 μM custom Y-adapter was used for adapter ligation. For comparison with commercial standard Ion Torrent adapters, library preparation was conducted in parallel, employing the GeneRead DNA Library L Core Kit (Qiagen) on the same batch of fragmented DNA according to the manufacturer's instructions, using each 1 μl barcoded adapter and P1 adapter (Ion Xpress™ Barcode Adapters, Thermo Fisher Scientific, Darmstadt, Germany). Subsequently, the libraries were purified using 1.8 volumes AMPure XP magnetic beads (Beckman Coulter, Krefeld, Germany) according to the manufacturer's instructions. Finally, the library was eluted twice with 50 μl DNase-free water for a total of 100 μl purified library. In the experiments with low DNA input, one fifth of the purified library was retained for subsequent library analysis. Size exclusion was performed with AMPure XP magnetic beads in two steps for a final fragment size distribution of 500–600 bp according to Wylezich and colleagues [Citation11]. After quality control (QC) with an Agilent High Sensitivity DNA Chip on a Bioanalyzer 2100 (both Agilent Technologies, Waldbronn, Germany), the libraries were quantified using KAPA Library Quantification Kit for Ion Torrent platforms (Roche) according to the manufacturer's instructions. After the PCR had finished, a melting curve analysis was performed to distinguish between library and adapter dimer molecules. Sequencing performance of Y-adapter libraries was tested and validated on an Ion Torrent PGM or an Ion S5 XL sequencer (Thermo Fisher Scientific). All steps for template preparation and sequencing were done as per the manufacturer's instructions, except for an additional denaturation of the library pool at 96°C for 2 min, followed by immediate cooling on ice right before the addition of the library pool to the emulsion PCR reaction. This denaturation of the library pool is necessary because both strands can serve as template molecules and will otherwise lead to polyclonal reads.
Data analysis
For a rough estimation of the melting point of the Y-adapters' double-stranded region, the software MELTING v5.2.0 [Citation12] was used. This software was chosen as it considers the influence of suboptimal base pairing through inosine nucleotides. Specified parameters comprised the salt and oligonucleotide concentrations (50 mM Na+, 10 mM Tris+, 25 μM each oligonucleotide) as present in the buffer used for hybridization of the two oligonucleotides. For reliable comparison of sequencing data, an additional trimming was conducted after the trimming already performed by the Ion Torrent Software Suite, since the 3′ adapter extensions of the sequences occurring with custom sequences were not trimmed by the Ion Torrent Software Suite. To this end, Trim Galore v. 0.4.3 at Galaxy Europe [Citation13] was used for 3′ Y-adapter and quality trimming (additional command line parameters: -e 0.1 -q 20 -O 3, with sequences shorter than 50 nt removed). For the quality assessment of the generated sequence data, FastQC [Citation14] and qrqc [Citation15] with R-Studio (Version 1.1.456; RStudio, Inc. [Citation16]) and R (Version 3.5.1; R Core Team [Citation17]) were used. Consistent analysis of the sequence quality was ensured by bringing the datasets to a uniform sequencing read length and amount. To this end, the 15 nt always exhibiting a deviating per base sequence content at the 5′-end in both the standard and Y-adapter libraries were removed. Moreover, to ensure comparability, long reads were shortened to a length of 310 nt, and reads shorter than 310 nt were removed, resulting in 342,715 reads corresponding to 1,370,860 bases in the analyzed datasets.
Results & discussion
In general, Y-adapters are composed of two oligonucleotides (hereinafter named top and bottom oligo) that include a mutual complementary region forming a double-stranded region in the final adapters. Additionally, each oligonucleotide comprises a noncomplementary part representing unique 3′ and 5′ features. This design results in the Y-shaped structure of a ready-to-use adapter ().
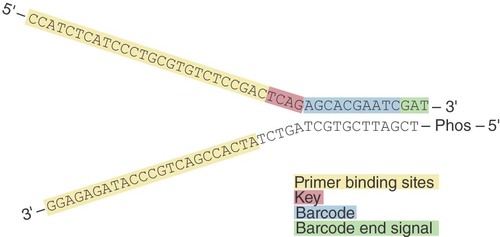
We used the information available for established Y-adapters for Roche/454 sequencing [Citation10] as a starting point for the initial design of our Y-adapters for Ion Torrent platforms. Importantly, the sequence of the barcoded oligonucleotide of the commercial Ion Xpress™ Barcode is identical with the top oligo in order to conserve all necessary functionalities and to avoid sequencing interference ().
The functionalities encompass the priming sites for clonal amplification and sequencing, as well as the sequence key used for the recognition of library molecules and signal normalization, and the barcode with the barcode end signal (the bases GAT). The T nucleotide forms the overhang required for sticky-end ligation. The bottom oligo comprises information of the P1 adapter, which is the primer binding site for the clonal amplification, and is 5′-phosphorylated to promote ligation to the library fragment. The original P1 adapter also contains an extension for probe binding for optional library quantification by probe-based qPCR that is not included in our design. Since the described regions must be unaltered to ensure full functionality of the adapter for amplification and sequencing, the necessary double-stranded region, also called ‘stem’, can only be formed by the barcode and the immediately adjacent regions.
In the initial experiments, two adapter variants analogous to the 454 Y-adapter design were tested, mainly differing in the stem length (20–22 bp and 16–18 bp) and used barcode (A). In the long variant, barcode (including end signal), key and additional four base pairs upstream of the barcode formed the stem, while in the short variant only the barcode (including end signal) and key were complementary. The simple fusion of the 454 structural elements and Ion Torrent sequences to meet requirements for sequencing delivered varying results in library yield as quantified by KAPA-PCR (A). By contrast, the traces of the high-sensitivity DNA electrophoresis showed a uniform size distribution for all six libraries with a peak at 600 bp (B). After equimolar pooling of the libraries containing the short/long adapter versions in one run each, the sequencing results of single libraries did not fit the expectation of the read-length distribution (compare B) and comparable read amounts, indicating suboptimal adapter design (C). This knowledge gained from the initial experiments served as a starting point for testing of varied adapters for identification of critical characteristics. For a comparable performance regarding the read length and quality, the melting temperature and the inclusion of PT bonds protecting the adapters from degradation were necessary.
(A) Initial design of the stem regions with three different barcodes (BC-042, 043, 044) and two variants each of the stem length. The bar plot shows the library yield (upper x-axis), with 500 ng Escherichia coli DNA input for each of the designs; mean and standard deviation were calculated from three dilutions used in qPCR quantification. ×: Melting temperature (lower x-axis) of the respective stem. (B) Size distribution of the generated libraries as determined by Bioanalyzer high-sensitivity DNA analysis. (C) Read length histogram of sequencing results of equimolar pools of the long and short adapters in one run each, respectively. For comparison, please see B showing the expected size distributions.
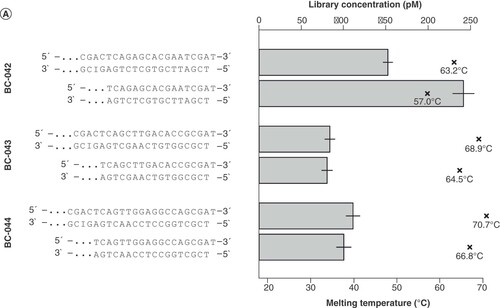
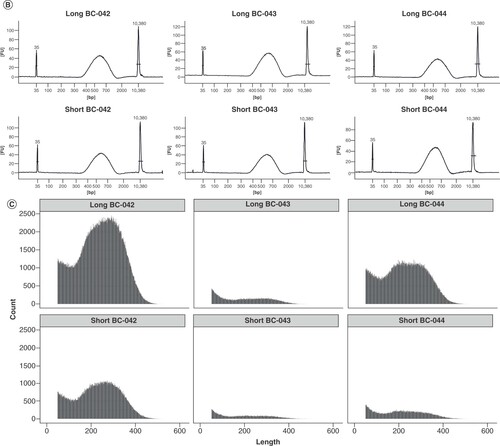
Narrow optimum range of Tm
The initial experiments showed that the library yield was highest using the adapter with the shortest barcode of 10 bp in combination with an overall short complementary region (see ). This implied that the Tm of the stem region was an important determinant of the functionality of the adapter. The melting point can be adjusted by (i) the length of the complementary double-stranded region and (ii) the inclusion of inosine nucleotides. Hypoxanthin, the nucleobase of inosine, can pair with any natural DNA base; however, the pairing is favored with cytosine, corresponding to the highest stability [Citation18]. To test for the impact of the Tm, we analyzed the consequences of the substitution of one or more bases by inosines. As shown in , the reduction of the Tm of the Y-adapter with barcode 44 (BC-044) approximately doubled the performance when reducing Tm to 64°C but still did not reach the performance of adapters with lower Tm. On the other hand, the inclusion of one or two inosine bases into BC-042 reduced both the Tm (from 49°C to 34°C) and the performance by approximately 70%. Likewise, in case of BC-009, the reduction of Tm by inclusion of inosines reduced the performance of library preparation. The available data suggest the existence of a temperature optimum between approximately 45°C and 55°C ().
Design of the stem regions with three different barcodes and varying numbers of inosine nucleotides (bold). Input: 250 ng of cell culture DNA. The barplot shows the library yield (upper x-axis) for each of the designs, mean and standard deviation were calculated from three dilutions used in qPCR quantification.
×: Melting temperature (lower x-axis) of the respective stem.
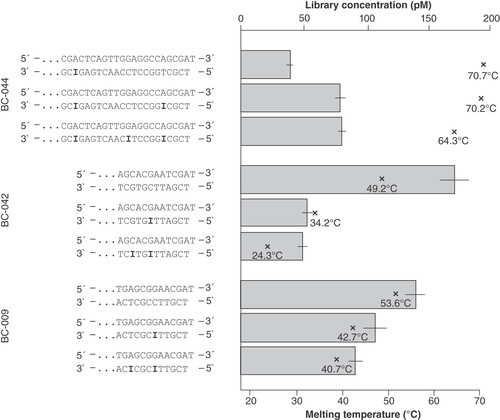
Phoshorothioate bonds are necessary at specific sites
Phoshorothioate (PT) bonds prevent degradation by endo- and exonucleases through the substitution of a nonbridging oxygen atom for a sulphur atom in the internucleotide linkage [Citation19,Citation20]. PT bonds are usually present in sequencing library adapters to prevent adapter and library decomposition. Interestingly, the position of the PT bonds varies: while in Roche 454 adapter oligonucleotides both ends each contained four PT bonds, Ion Torrent adapters have two PT bonds in the middle of the adapter at the end position of the shorter strand. Therefore, we determined if, how many, and at which sites PT bonds are necessary for optimal library yield and sequencing performance. To this end, we used optimized adapters with a short double-stranded region from previous experiments and included PTs at the end and parting positions of the two adapter strands (). The results of library quantification ( & Supplementary Figure S1) clearly underline the necessity of at least one PT bond at the ends of the adapter oligonucleotides. However, the library yield regarding the presence of one, two, three and four PT bonds was comparable. The PT bonds are likely preventing primer site degradation and enzymatic cleavage of the adapter's T overhang, which in consequence would obstruct sticky end ligation to the A-tailed library fragments. While the PT bonds in the termini of the adapter oligonucleotides had a significant impact, the internal PT bonds resulted in no beneficial effect. An additional protective effect could not be observed in combination with the terminal PT bonds, nor could any protection at all be seen in the case of internal PT bonds alone ().
Design of the top oligonucleotides with barcode BC-042 and varying numbers of phosphorothioate bonds and different positions. Libraries were prepared from 500 ng fragmented Escherichia coli DNA in two independent experiments. The bar plot shows the library yield for each of the designs; mean and standard deviation were calculated from three dilutions used in qPCR quantification. Colored adapter sequence: Black: primer binding sites; red: key; blue: barcode; green: barcode end signal. The adapter part forming the double-stranded stem is shown with grey background.
*Phosphorothioate bonds.
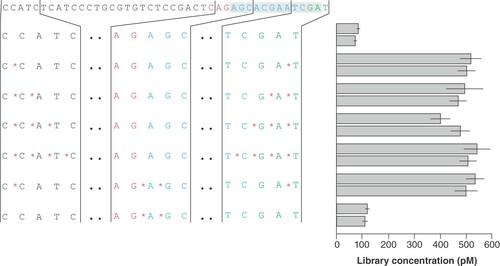
Y-adapters result in reduced adapter-dimer formation
In cases of limited sample material, efficient conversion of sample to library is crucial. Therefore, we compared the library yield at low input amounts of 2, 10 and 50 ng (A). Additionally, we quantified the formation of adapter dimers without any input DNA in the library preparation. The Y-adapter dimer formation is substantially reduced in comparison to standard adapters. At 2 ng input into library preparation, the quantification implies comparable library yield with Y- and standard adapters. However, the library yield in the standard adapter library is overestimated due to adapter-dimer formation, which is noticeable in the melting curve of the quantification PCR (B). The adapter dimers, perceived as library molecules in the quantification, occupy sequencing capacities during a run without providing template information and are therefore undesirable. At 10 ng input DNA, Y-adapter libraries already have higher concentrations, although the presence of adapter dimers can still be identified in the melting curve of standard adapter libraries (B). At 50 ng input DNA, the enhanced library yield caused by sticky-end ligation of Y-adapters is clearly visible (A) and potential adapter dimers are no longer observable in the melting curves (Supplementary Figure S2).
(A) Library concentration after library preparation without and with 2, 10 and 50 ng fragmented Escherichia coli DNA input. For optimal comparability, experiments were performed in duplicates with standard adapter BC-042 and Y-adapter BC-042, respectively. Mean and standard deviation were calculated from three dilutions used in qPCR quantification. (B) Melting curves generated after qPCR quantification for 2- and 10-ng DNA input, visualizing the presence of adapter dimers. Reference was assembled of single peaks for nontemplate control (primer dimer; yellow), library without input (adapter dimer; red) and library quantification standard (153 bp; black).
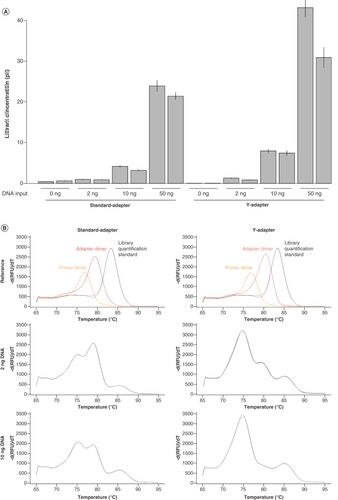
Y-adapters enable uniform ligation efficiency
Since the library preparation represents a decisive step in a sequencing workflow, adapters are required to function reliably and comparably in independent preparations. Pooling (multiplexing) of different samples in one sequencing run calls for a batch of adapters performing comparably well. For verification of uniform performance of the developed Y-adapters, eight adapters with different barcodes were manufactured based on obtained knowledge of melting temperature and PT bond requirements obtained from the previous experiments. The performances were compared regarding efficiency in library preparation and functionality in sequencing. The same fragmented E. coli DNA served as input material for all library preparations. Overall, we observed high library yields for seven out of eight adapters used (A). However, sticky-end ligation to adapter carrying barcode 70 (BC-070) resulted in significantly less library, demonstrating the need for evaluation of Y-adapter performance before their routine use in a production environment. Despite the lower efficiency of the BC-070 adapter in library preparation, all libraries performed equally well when sequenced multiplexed in one full Ion S5 sequencing run. Sequencing of this equimolar library pool produced equal read amounts for all libraries, including BC-070. The key signal was strong and the read length distribution as expected on basis of the library size.
(A) Eight optimized Y-adapters for Ion Torrent sequencing were compared on their ligation efficiency when ligated to the same fragmented Escherichia coli DNA, 500 ng, in two or three (BC-009, BC-042) independent experiments. The plot shows the library yield for each of the adapters; mean and standard deviation were calculated from three dilutions used in qPCR quantification. The horizontal line indicates the mean concentration of all libraries shown in the plot, the dashed lines indicate the mean ± 1 standard deviation. (B) Key metrics of sequence data generated on an Ion S5 XL using standard Ion Torrent adapters or custom Y-adapters. Read length histograms for E. coli libraries prepared using Y-adapters (top) or standard adapters (bottom). (C) Quality plot of the same libraries after trimming to a uniform sequence length and number of bases.
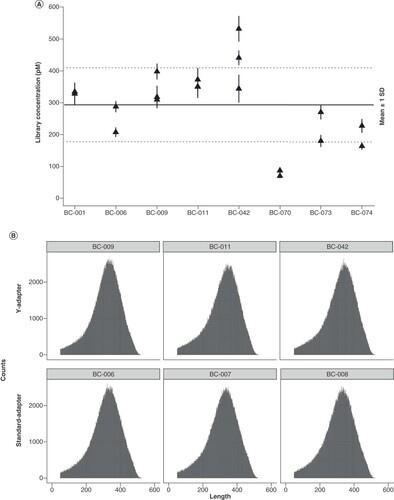
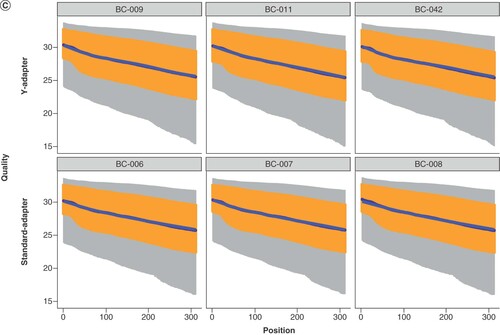
Y-adapters equal sequencing performance of commercial linear adapters
The highest library yields are meaningless if the ligated adapters do not perform well in sequencing. For comparison, the same fragmented E. coli DNA was ligated to three commercial adapters and three custom Y-adapters. The achieved library yields ranged between 211 and 288 pM for ligation with linear adapters and 399 and 442 pM for ligation with Y-adapters, respectively. Sequencing was performed in one Ion S5 run and resulted in overall tantamount read lengths for all libraries (B). For direct comparison of sequencing quality, the reads were trimmed to the same length to subtract template-independent decay of the read quality towards the ends. This trimming provided an equal amount of bases, which showed a comparable quality distribution for standard and Y-adapters (C). The percentage of sequences remaining after deduplication varied between 90.5 and 93.0% for standard adapters, and between 91.2 and 91.9% for Y-adapters, as determined by FastQC. In consequence, Y-adapters truly enable higher library yields at constant complexity levels, an advantage in comparison with library amplification that generates higher quantity at the cost of reduced library complexity and increased bias [Citation21,Citation22].
Evaluation on field samples
The Y-adapter ligation efficiency was compared on samples for diagnostic metagenomic analyses, with different samples combined with varying amounts of DNA input (). Thereof, libraries for sequencing on Ion Torrent platforms were prepared using the blunt end ligation with linear adapters in comparison to sticky end ligation with custom Y-adapters. The results clearly show that the Y-adapters resulted in a more than twofold higher library concentration at the same input. This observation is independent of the amount of starting material. Additionally, the custom Y-adapters were successfully applied in the discovery of a novel picornavirus in an open diagnostic metagenomics approach on a case series of diseased lambs [Citation23]. In this example of one possible application, extracted RNA was reverse transcribed into cDNA, which after fragmentation served as input for library preparation.
Shown are the mean yield, including standard deviation calculated from three dilutions used in qPCR quantification.
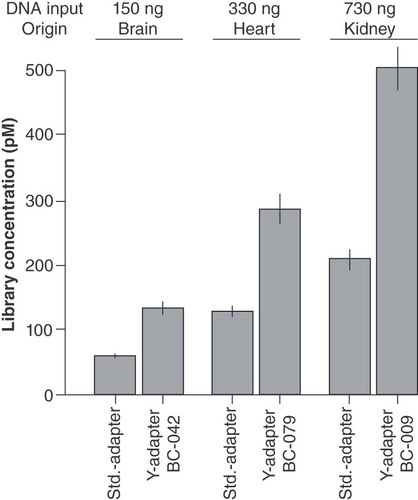
In summary, we developed and validated Y-adapters for Ion Torrent sequencing, which are neither commercially available nor have been described elsewhere before. Y-adapters enable more efficient library preparation by (i) sticky-end ligation and (ii) rendering both DNA strands functional for sequencing. The described Y-adapters enable higher library yields in comparison to standard Ion Torrent adapters. Moreover, the generation of adapter dimers that reduce sequencing output is minimized. With regard to the key performance characteristics (read-length, base quality, library complexity), libraries generated using our presented Y-adapters equal those of Ion Torrent standard libraries. Due to the higher possible library yield, the Y-adapters truly enable an efficient conversion of sample to functional library molecules and therefore enable omitting library amplification. Moreover, the herein described information builds a basis for other researchers in the broad applications of high-throughput sequencing to enhance their library yield by designing their own custom Y-adapters compatible with Ion Torrent platforms. Manufacturing custom adapters drastically reduces the adapter cost per library. In addition, the presented Y-adapters may be of interest to research groups performing sequencing on both Ion Torrent and Illumina platforms, since it facilitates a unified, amplification-free, automatable protocol for Illumina and Ion Torrent library preparation only differing in the sequences of the added adapters.
Future perspective
With the costs for sequencing and analysis further decreasing, NGS use will further increase for various types of scientific analyses and will become a standard technique in routine diagnostics. From the viewpoint of diagnostics, the restrictions imposed by the manufacturers – that is, the use of only certified library preparation and sequencing reagents from the original supplier – can be beneficial because they ensure reproducibility and standardization. From the scientific viewpoint, however, this is an unwanted restriction because it removes an element of choice and innovation. The presented Ion Torrent custom Y-adapters provide a more efficient and cheaper conversion of DNA into library and additionally provide a starting point for innovation and hence introduction of new flexibility, ultimately leading to further increase in the application of NGS in all fields.
Data availability
Escherichia coli sequencing data of the comparison of custom Y-adapters with standard adapters have been deposited within the European Nucleotide Archive under the project accession number PRJEB30332.
Author contributions
Study design and conceptualization, LF and DH; experimental studies, LF; data interpretation and discussion, LF and DH; manuscript preparation and review, LF and DH.
Authors’ original file for figure 1
Download MS Word (44.7 KB)Authors’ original file for figure 2
Download PDF (940.8 KB)Acknowledgments
The authors gratefully acknowledge Jenny Lorke and Patrick Zitzow for excellent technical assistance and support.
Financial & competing interests disclosure
This research was funded by the German Federal Ministry of Education and Research within project “DetektiVir” (grant number 13N13783). The authors have no other relevant affiliations or financial involvement with any organization or entity with a financial interest in or financial conflict with the subject matter or materials discussed in the manuscript apart from those disclosed.
No writing assistance was utilized in the production of this manuscript.
Additional information
Funding
References
- Levy SE , MyersRM. Advancements in next-generation sequencing. Annu. Rev. Genomics Hum. Genet.17, 95–115 (2016).
- Buermans HP , den DunnenJT. Next generation sequencing technology: advances and applications. Biochim. Biophys. Acta.1842(10), 1932–1941 (2014).
- Reuter JA , SpacekDV, SnyderMP. High-throughput sequencing technologies. Mol. Cell58(4), 586–597 (2015).
- Bentley DR , BalasubramanianS, SwerdlowHPet al. Accurate whole human genome sequencing using reversible terminator chemistry. Nature456(7218), 53–59 (2008).
- Rothberg JM , HinzW, RearickTMet al. An integrated semiconductor device enabling non-optical genome sequencing. Nature475(7356), 348–352 (2011).
- Head SR , KomoriHK, LaMereSAet al. Library construction for next-generation sequencing: overviews and challenges. BioTechniques56(2), 61–77 (2014).
- Zhao G , LiJ, HuT, WeiH, GuanY. Realizing directional cloning using sticky ends produced by 3′-5′ exonuclease of Klenow fragment. J. Biosci.38(5), 857–866 (2013).
- Quail MA , KozarewaI, SmithFet al. A large genome center's improvements to the Illumina sequencing system. Nat. Methods5(12), 1005–1010 (2008).
- Zheng Z , AdvaniA, MeleforsÖet al. Titration-free 454 sequencing using Y adapters. Nat. Protoc.6(9), 1367–1376 (2011).
- Roche . Multiplex Identifier (MID) Adaptors for Rapid Library Preparations. In: Technical Bulletin GS FLX System & GS Junior System.8–9 (2010).
- Wylezich C , PapaA, BeerM, HöperD. A versatile sample processing workflow for metagenomic pathogen detection. Sci. Rep.8(1), 13108 (2018).
- Dumousseau M , RodriguezN, JutyN, LeNovère N. MELTING, a flexible platform to predict the melting temperatures of nucleic acids. BMC Bioinformatics13(1), 101 (2012).
- Afgan E , BakerD, BatutBet al. The Galaxy platform for accessible, reproducible and collaborative biomedical analyses: 2018 update. Nucleic Acids Res.46(W1), W537–W544 (2018).
- Babraham Bioinformatics. FastQC. http://www.bioinformatics.babraham.ac.uk/projects/fastqc
- GitHub . vsbuffalo/qrqc. http://github.com/vsbuffalo/qrqc
- RStudio . www.rstudio.com
- The R Project for Statistical Computing . www.R-project.org
- Alseth I , DalhusB, BjøråsM. Inosine in DNA and RNA. Curr. Opin. Genet. Dev.26, 116–123 (2014).
- Spitzer S , EcksteinF. Inhibition of deoxyribonucleases by phosphorothioate groups in oligodeoxyribonucleotides. Nucleic Acids Res.16(24), 11691–11704 (1988).
- Vosberg HP , EcksteinF. Effect of deoxynucleoside phosphorothioates incorporated in DNA on cleavage by restriction enzymes. J. Biol. Chem.257(11), 6595–6599 (1982).
- Dabney J , MeyerM. Length and GC-biases during sequencing library amplification: a comparison of various polymerase-buffer systems with ancient and modern DNA sequencing libraries. BioTechniques52(2), 87–94 (2012).
- Kozarewa I , NingZ, QuailMA, SandersMJ, BerrimanM, TurnerDJ. Amplification-free Illumina sequencing-library preparation facilitates improved mapping and assembly of (G+C)-biased genomes. Nat. Methods6(4), 291–295 (2009).
- Forth LF , ScholesSFE, PesaventoPAet al. Novel picornavirus in lambs with severe encephalomyelitis. Emerg. Infect. Dis.25(5), 963–967 (2019).