Abstract
Programmed-1 ribosomal frameshifting (-1 PRF) is a translational mechanism adopted by some viruses, including SARS-CoV-2. To find a compound that can inhibit -1 PRF in SARS-CoV-2, we set up a high-throughput screening system using a HeLa cell extract-derived cell-free protein synthesis (CFPS) system. A total of 32,000 compounds were individually incubated with the CFPS system programmed with a -1 PRF-EGFP template. Several compounds were observed to decrease the -1 PRF-driven fluorescence, and one of them had some suppressive effect on -1 PRF of a SARS-CoV-2 genome sequence in transfected cells. Thus the CFPS system can be used as a tool for a high-throughput screening of chemicals.
The genomic RNA of coronaviruses including SARS-CoV-2 is translated into viral proteins in the infected cells, and one of the viral proteins is the RNA-dependent RNA polymerase (RdRp). RdRp uses the original RNA as the template to make copies of the genomic and subgenomic RNAs, eventually leading to amplification of the virus [Citation1,Citation2]. Translation of the coronavirus RNA involves a special process called programmed-1 ribosomal frameshifting (-1 PRF), and RdRp is generated due to this process [Citation3,Citation4]. In other words, if this -1 PRF is inhibited, RdRp will not be produced and virus replication will be stopped. Importantly, -1 PRF is a special translational mechanism adopted by viruses such as the coronaviruses and the acquired immunodeficiency syndrome virus [Citation5], and mRNA derived from human cells is not translated in this way. Therefore, drugs that could suppress the viral frameshifting are unlikely to interfere with endogenous cell functions. Thus, while current therapeutic strategies to overcome COVID-19 have focused on the development of vaccines and drugs that inhibit the virus-specific 3CL protease [Citation6] or RNA polymerase [Citation7], -1 PRF is another promising target for the development of anti-SARS-CoV-2 agents [Citation8].
We have developed a HeLa cell extract-derived cell-free protein synthesis (CFPS) system [Citation9,Citation10]. This system enabled us to reconstitute eukaryotic translation initiation factor 3, a protein complex of 12 subunits, by coexpressing all the subunits [Citation11], and helped us to analyze the aggregation status of polyglutamine stretches in vitro [Citation12]. The RNA-based encephalomyocarditis virus has been successfully synthesized from a synthetic RNA [Citation13] and even from a DNA template in this CFPS system [Citation14].
Here we report the use of the in vitro protein expression system to screen 32,000 compounds in a search for an inhibitor of -1 PRF of SARS-CoV-2.
Materials & methods
Plasmids
The template plasmid for screening pUC-T7-HCV IRES-FLAG-CAT-Fs-EGFP-HA (A) was constructed as follows. The complementary DNA (5′-ATGTGGAAAGGTTATGGCTGTAGTTGTGATCAACTCCGCGAACCCATGCTTCAGTCAGCTGATGCACAATCGTTTTTAAACGGGTTTGCGGTGTAAGTGCAGCCCGTCTTACACCGTGCGGCACAGGCACTAGTACTGATGTCGTATACAGGGCTTTTGACATCTACAATGAT-3′) for an RNA sequence that spans the frameshift region (Fs) of SARS-CoV-2 (GenBank: NC_045512.2) was inserted between the chloramphenicol acetyltransferase (CAT) [Citation15] and EGFP-HA [Citation16] open reading frames (ORFs), and the resulting DNA (CAT-Fs-EGFP-HA) was incorporated into the plasmid pUC-T7-HCV IRES-FLAG [Citation9]. For transfection, the plasmid pCIneo-Fs-EGFP-HA was constructed by insertion of the Fs sequence 5′ upstream of the EGFP-HA ORF in the pCIneo-EGFP-HA plasmid [Citation16] such that when a -1 frameshift occurs, the EGFP protein should be translated.
(A) Schematic structure of the template for screening. A sequence around the -1 PRF site is presented. The Slippery motif and the in-frame termination codon are highlighted; -1 PRF occurs at the Slippery motif [Citation3]. (B) The template was incubated in the CFPS system with or without the antisense DNA oligonucleotide to the 3’ end of the frameshift region. EGFP fluorescence of the samples was measured by a microplate reader (left panel; n = 32) and then the protein bands were resolved by 12.5% SDS-PAGE followed by western blotting with anti-FLAG M2-peroxidase (right panel). (C) Screening steps. (D) Flow of screening.
CFPS: Cell-free protein synthesis; Fs: Frameshift; -1 PRF: Programmed-1 ribosomal frameshifting.
![Figure 1. Setting up the screening system. (A) Schematic structure of the template for screening. A sequence around the -1 PRF site is presented. The Slippery motif and the in-frame termination codon are highlighted; -1 PRF occurs at the Slippery motif [Citation3]. (B) The template was incubated in the CFPS system with or without the antisense DNA oligonucleotide to the 3’ end of the frameshift region. EGFP fluorescence of the samples was measured by a microplate reader (left panel; n = 32) and then the protein bands were resolved by 12.5% SDS-PAGE followed by western blotting with anti-FLAG M2-peroxidase (right panel). (C) Screening steps. (D) Flow of screening.CFPS: Cell-free protein synthesis; Fs: Frameshift; -1 PRF: Programmed-1 ribosomal frameshifting.](/cms/asset/9b3a9aca-aec2-4685-b768-fd81cc9dea90/ibtn_a_12367238_f0001.jpg)
Chemicals & proteins
The Core 9600 library and Advanced Core 22400 library were provided by the BINDS (Basis for Supporting Innovative Drug Discovery and Life Science Research) project (AMED, Japan); each compound (50 and 200 pmol, respectively) was dissolved in DMSO (25 and 100 nl, respectively) and applied to each well of 384-well plates. T7 RNA polymerase and GADD34 were bacterially produced and then purified [Citation9,Citation17].
Cell extracts
The HeLa cell extract for the CFPS system was prepared following the reported protocol [Citation18] with some modifications. HeLa S3 cells (1.0 l) were grown in a spinner flask regulated with a Cellmaster Model 1700 cell culture controller (Wakenyaku, Kobe, Japan) in Joklik Minimal Essential Medium Eagle (Sigma-Aldrich, MO, USA) supplemented with 4 g/l sodium hydrogen carbonate, 10% heat-inactivated calf serum, 1% penicillin–streptomycin mixed solution (Nacalai, Kyoto, Japan) and 1% l-alanyl-l-glutamine solution (Nacalai). The culture conditions were 5% CO2, 37°C, pH 7.0, 6.77 p.p.m. oxygen, with stirring at 50 r.p.m. When the cell density reached approximately 1.0 × 106 cells/ml, cells were harvested by centrifugation at 270 × g for 10 min at 4°C and then washed three-times with a buffer (25 ml; 35 mM Hepes-KOH pH 7.5, 140 mM NaCl, 11 mM glucose) and once with an extraction buffer (25 ml; 20 mM Hepes-KOH pH 7.5, 30 mM KCl, 1.65 mM magnesium acetate) by centrifugation at 130 × g for 10 min at 4°C. The cell pellet was suspended in an equal volume of the extraction buffer and was disrupted by nitrogen pressure (1.0 MPa, 30 min) in a mini-Bomb cell disruption chamber (Kontes Glass, NJ, USA). After addition of 1/30 volume of a buffer (20 mM Hepes-KOH pH 7.5, 900 mM potassium acetate, 30 mM KCl, 1.65 mM magnesium acetate), the cell homogenate was centrifuged twice at 1700 × g for 5 min at 4°C. The supernatant (the HeLa cell extract: 10–15 mg protein/ml) was frozen in liquid nitrogen and stored at -80°C.
Screening of compounds
The HeLa cell extract (3.8 ml; 20.7 mM Hepes-KOH pH 7.5, 31 mM KCl, 1.71 mM magnesium acetate, 30 mM potassium acetate) was mixed with mixture 1 (0.9 ml; 12.5 mM ATP, 8.3 mM CTP, 8.3 mM GTP, 8.3 mM UTP, 200 mM creatine phosphate, 0.6 mg/ml creatine kinase, 0.3 mM each of 20 amino acids, 2.5 mM spermidine), mixture 2 (3.3 ml; 138.5 mM Hepes-KOH pH 7.5, 290.8 mM potassium acetate, 10.5 mM magnesium acetate, 2.77 mM DTT), GADD34 (200 μl; 0.15 μg/μl), T7 RNA polymerase (300 μl; 0.6 μg/μl) and the template plasmid pUC-T7-HCV IRES-FLAG-CAT-Fs-EGFP-HA (500 μl; 0.5 μg/μl). The resulting mixture (9.0 ml for two 384-well plates) was dispensed into each well (5 μl/well) of the BINDS-provided 384-well plates using an electric pipette (VIAFLO II 16ch: Integra Biosciences, NH, USA) controlled by a dispensing machine (ASSIST: Integra Biosciences). The plates were then incubated at 32°C for 90 min in a shaker (800 r.p.m.; DWMaxM/BR-034P: Taitec, Koshigaya, Japan); the plates were sealed with a polyester film (Easy seal #676001: Greiner Bio-One, Frickenhausen, Germany) to prevent evaporation during incubation. Subsequently, fluorescence (excitation 485 nm, emission 535 nm) from each well was measured using a plate reader (GENios: Tecan, Männedorf, Switzerland). For the inhibition control, the antisense DNA (5 μM) against the 3′ part of the Fs sequence (5′-TTTGACATCTACAATGAT) was applied to some wells.
Analysis of the fluorescence data
EGFP fluorescence (excitation 485 nm, emission 535 nm) from each well was measured by a microplate reader (GENios), and the value of T/C: 100 × treated/control (%) was calculated, where ’treated’ represents the fluorescence value of each well to which a compound had been applied and ’control’ represents the average value of fluorescence of the wells to which only DMSO had been applied. For assessment of the assay, the untreated/treated ratio (signal/background [S/B] ratio) was calculated using the equation S/B = Av100%/Av0%, where Av100% and Av0% are the mean values of positive (DMSO) and negative (DMSO + antisense DNA) controls, respectively. Furthermore, the assay performance was assessed using the Z’-factor, defined by the equation Z’ = 1- [(3SD100%–3SD0%)/(Av100%–Av0%)], where SD100% and SD0% are the standard deviations of the positive and negative controls, respectively [Citation19].
Western blotting
After fluorescence measurement, reaction mixtures (5 μl each) were removed by pipetting from the wells that exhibited a decrease in fluorescence and were mixed with an equal volume of 2× SDS sample buffer (65.8 mM Tris-HCl pH 6.8, 26.3% [w/v] glycerol, 2.1% SDS, 0.01% bromophenol blue). After incubation at 95°C for 3 min, the samples were resolved by 12.5% SDS-PAGE at 150 V at room temperature. After electrophoresis, proteins in the gel were transferred to a polyvinylidene difluoride membrane (IPVH00010: Millipore, MA, USA) in transfer buffer (24 mM Tris, 190 mM glycine, 20% v/v methanol) at 70 V for 2 h at 4°C. The membrane was then blocked with 1% skim milk in TN buffer (10 mM Tris-HCl pH 7.5, 10 mM NaCl) for 30 min. After blocking, the membrane was incubated with anti-FLAG M2-peroxidase (A8592: Sigma-Aldrich), anti-HA.11 Epitope Tag Antibody (MMS-101R: BioLegend, CA, USA) or β-actin antibody (C4, sc-47778: Santa Cruz Biotechnology, TX, USA) at 1:1000 dilution in 1% skim milk containing 0.2% Tween® 20 overnight at 4°C. In the case of anti-FLAG M2-peroxidase, after the membrane was washed three times with TN buffer containing 0.2% Tween 20 for 5 min each, Immobilon western chemiluminescent horseradish peroxidase substrate (WBKLS0500: Millipore) was applied to the membrane. FLAG-tagged proteins on the membrane were visualized by LAS4000 mini (Fujifilm, Tokyo, Japan). When the anti-HA antibody or the anti-β-actin antibody was used, the membrane was washed three times with TN buffer containing 0.2% Tween 20 for 5 min each, and then incubated with a peroxidase-linked secondary antibody (NA931: Cytiva, Uppsala, Sweden) at 1:1000 dilution for 2 h at room temperature. The membrane was treated as described above and proteins were visualized by LAS4000 mini (Fujifilm).
Transfection
First, 48-well plates were seeded with HEK293T cells (5.0 × 104/well). After 16–24 h, the cells were transfected with pCIneo-EGFP-HA or pCIneo-Fs-EGFP-HA using FuGENE 4K (Promega, WI, USA). After 24–28 h, cells were observed under a fluorescence microscope and then lysed with passive lysis buffer (20 μl/well, Promega). The supernatant after centrifugation (15,000 × g for 20 min) was used for western blotting with the anti-HA antibody and the anti-β-actin antibody.
Results & discussion
High-throughput screening
A high-throughput screening system for a compound that could inhibit -1 PRF in SARS-CoV-2 was constructed using a HeLa cell extract-derived CFPS system. The template DNA is depicted in A. The transcription/translation unit comprises the T7 RNA promoter (T7p); hepatitis C virus internal ribosome entry site; FLAG-CAT, a DNA sequence spanning the -1 PRF region of SARS-CoV-2 (Fs); EGFP-HA; and a T7 terminator (T7t). The Fs sequence harbors a translation termination codon in-frame with the FLAG-CAT ORF such that when -1 PRF occurs on the Slippery motif, the FLAG-CAT-Fs-EGFP-HA ORF is translated and the fluorescence of the EGFP fusion protein is detected (B). If a compound inhibits -1 PRF, the fluorescence is expected to decrease. As an inhibition control, an antisense DNA targeting the 3′ region of the Fs sequence suppressed the synthesis of the Fs product with a concomitant decrease in the detected fluorescence (B).
The HeLa cell extract supplemented with T7 RNA polymerase and the template DNA were dispensed into each well of the 384-well plates (Core 9600 library and Advanced Core 22400 library, provided by the BINDS project) so that the concentration of each compound reached 10 μM for the Core 9600 library or 40 μM for the Advanced Core 22400 library. The plates were incubated at 32°C for 1.5 h, and fluorescence from each well was measured (C). The results are shown in . The S/B ratios of Core 9600 and Advanced Core 22400 were 3.9 and 6.9, respectively, and the Z’-factor of Core 9600 and Advanced Core 22400 were 0.68 and 0.79, respectively, indicating the robustness of this screening system. Among 32,000 wells, fluorescence in 66 decreased to less than -3σ of the T/C (%) average value. Samples from these wells were analyzed by western blotting with the anti-FLAG antibody, and 16 samples showed a decrease in the yield of the FLAG-CAT-Fs-EGFP-HA protein. These 16 samples were rescreened in the same way but with different concentrations (1–40 μM), and two compounds (compound A and compound B) were confirmed to diminish the synthesis of FLAG-CAT-Fs-EGFP-HA as well as the fluorescence (D & ).
(A) T/C % values of Core Library 9600 compounds. The S/B and Z’-factors are calculated. (B) T/C % values of Advanced Core Library 22400 compounds. The S/B and Z’-factors are calculated.
B: Background; C: Control; S: Signal; T; Treated.
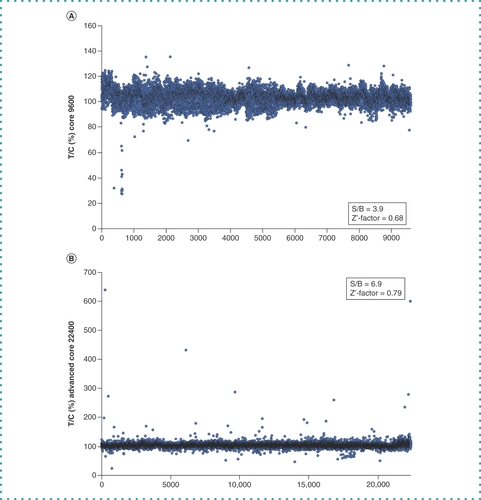
The template plasmid pUC-T7-HCV IRES-FLAG-CAT-Fs-EGFP-HA was incubated in the CFPS system with different concentrations (0–40 μM) of (A) compound A or (B) compound B on 384-well plates. After fluorescence was measured (upper panels), samples were resolved by 12.5% SDS-PAGE and then analyzed by western blotting with the anti-FLAG antibody (middle panels). Relative frameshifting (Fs) efficiency (FLAG-CAT-Fs-EGFP-HA / FLAG-CAT + FLAG-CAT-Fs-EGFP-HA) was calculated from the intensity of each protein band in the middle panels with the dimethyl sulfoxide control set to 100% (lower panels). Each column and error bar represent the mean and standard deviation of three wells, respectively.
CFPS: Cell-free protein synthesis; Fs: Frameshift.
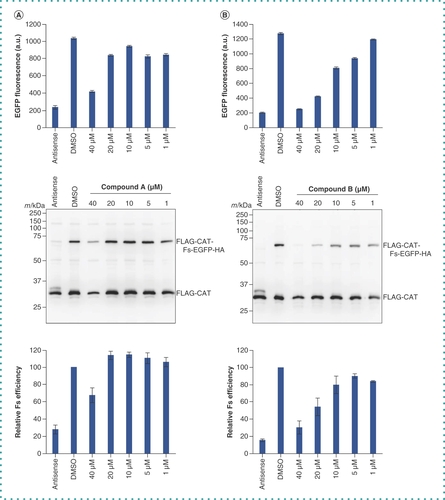
Cell-based assay
The two compounds (compound A and compound B; ) that survived the high-throughput screening were next tested to determine whether they could inhibit -1 PRF in cells. HEK293T cells were transfected with pCIneo-EGFP-HA or pCIneo-Fs-EGFP-HA, and the extracts from transfected cells were assayed by western blotting with anti-HA antibody. Compound A significantly inhibited translation of Fs-EGFP-HA compared with EGFP-HA, whereas compound B inhibited both similarly; compound B might have some cytotoxicity with an off-target effect that exists in the cellular assay system but not in the cell-free assay system ().
HEK293T cells were transfected with pCIneo-EGFP-HA or pCIneo-Fs-EGFP-HA and were treated with the different concentrations (0–10 μM) of (A) compound A and (B) compound B. The cells were harvested and treated as described in the Methods section, and the samples were resolved by SDS-PAGE followed by western blotting with the anti-HA antibody and the anti-β-actin antibody (left panels). The synthesis rate of EGFP-HA and Fs-EGFP-HA were calculated from each band intensity relative to that of β-actin with the dimethyl sulfoxide control (0 μM) set to 100%. Each column and error bar represent the mean and standard deviation of the three independent experiments, respectively. *p < 0.05, Student’s t-test.
Fs: Frameshift.
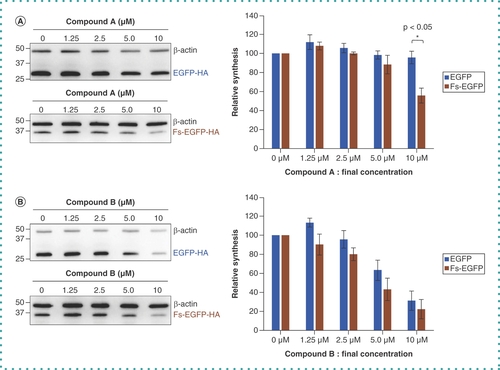
The chemical structure of compound A () is very different from that of 2-{[4-(2-methylthiazol-4-ylmethyl)-[1,4]diazepane-1-carbonyl]amino}benzoic acid ethyl ester (MTBD), which was identified as a SARS -1 PRF inhibitor through virtual screening of a chemical database followed by in vitro translation and cell-based assays [Citation20–22]. Thus, compound A could be another lead chemical for the development of an inhibitor against -1 PRF of SARS-CoV-2.
Conclusion
A high-throughput screening system for a SARS-CoV-2 frameshift inhibitor was constructed on the basis of the HeLa cell extract-derived CFPS system. Screening of 32,000 compounds resulted in two candidates, and one of them had some suppressive effect on -1 PRF of a SARS-CoV-2 genome sequence in transfected cells. The study demonstrates that the CFPS system can be used as a tool for high-throughput screening of chemicals.
Future perspective
This study verifies the usefulness of the CFPS system for high-throughput screening of chemicals. Other possible applications of the CFPS-based screening system include searching for inhibitors of translation specific to other viruses or cellular genes of interest. One could consider using the CFPS system for drug discovery related to post-translational events such as protein modifications. The protocol described here should help these future applications.
Background
A translational mechanism, programmed-1 ribosomal frameshifting (-1 PRF) is a promising target for the development of anti-SARS-CoV-2 drugs.
A HeLa cell extract-derived cell-free protein synthesis (CFPS) system has been developed and used to study the biogenesis of protein complexes and an RNA virus.
Experimental
A high-throughput screening system to search for a -1 PRF inhibitor was established using the CFPS system.
Results & discussion
A total of 32,000 compounds were screened for -1 PRF inhibition.
Two compounds passed the screening.
One of them had some suppressive effect on -1 PRF of a SARS-CoV-2 genome sequence in transfected cells.
Conclusion
This compound could be a lead chemical toward the development of an inhibitor against -1 PRF of SARS-CoV-2.
The CFPS system is verified to be useful as a tool for high-throughput screening of chemicals.
Author contributions
K Machida and H Imataka conceived the study and designed the experiments. R Tanaka, S Miki, S Noseda and M Yuasa-Sunagawa performed the experiments. R Tanaka and K Machida analyzed the data. K Machida and H Imataka wrote the paper.
Financial disclosure
This work was financially supported by JSPS KAKENHI grants (21H02468 to K Machida and 22H02612 to H Imataka), a Grant-in-Aid for Transformative Research Areas (21H05727 to K Machida and 21H05277 to H Imataka), AMED-CREST (Advanced Research and Development Programs for Medical Innovation: 21gm1410008s0101), Kinoshita Foundation to K Machida, and Takeda Science Foundation to H Imataka. The authors have no other relevant affiliations or financial involvement with any organization or entity with a financial interest in or financial conflict with the subject matter or materials discussed in the manuscript apart from those disclosed.
Competing interests disclosure
The authors have no competing interests or relevant affiliations with any organization or entity with the subject matter or materials discussed in the manuscript. This includes employment, consultancies, honoraria, stock ownership or options, expert testimony, grants or patents received or pending, or royalties.
Writing disclosure
No writing assistance was utilized in the production of this manuscript.
Acknowledgments
The authors gratefully acknowledge Basis for Supporting Innovative Drug Discovery and Life Science Research from AMED under grant number JP22ama121053 (support no. 2487) for providing us with compounds for screening and valuable advice.
References
- Hartenian E , NandakumarD , LariA , LyM , TuckerJM , GlaunsingerBA. The molecular virology of coronaviruses. J. Biol. Chem.295(37), 12910–12934 (2020).
- V’kovski P , KratzelA , SteinerS , StalderH , ThielV. Coronavirus biology and replication: implications for SARS-CoV-2. Nat. Rev. Microbiol.19(3), 155–170 (2021).
- Bhatt PR , ScaiolaA , LoughranGet al. Structural basis of ribosomal frameshifting during translation of the SARS-CoV-2 RNA genome. Science372(6548), 1306–1313 (2021).
- Kelly JA , OlsonAN , NeupaneKet al. Structural and functional conservation of the programmed-1 ribosomal frameshift signal of SARS coronavirus 2 (SARS-CoV-2). J. Biol. Chem.295(31), 10741–10748 (2020).
- Brierley I , DosRamos FJ. Programmed ribosomal frameshifting in HIV-1 and the SARS-CoV. Virus Res.119(1), 29–42 (2006).
- Zhu W , XuM , ChenCZet al. Identification of SARS-CoV-2 3CL protease inhibitors by a quantitative high-throughput screening. ACS Pharmacol. Transl. Sci.3(5), 1008–1016 (2020).
- Chen J , FarrajRA , LimontaDet al. Reversible and irreversible inhibitors of coronavirus Nsp15 endoribonuclease. J. Biol. Chem.299(11), 105341 (2023).
- Ahidjo BA , LoeMWC , NgYL , MokCK , ChuJJH. Current perspective of antiviral strategies against COVID-19. ACS Infect. Dis.6(7), 1624–1634 (2020).
- Mikami S , KobayashiT , MasutaniM , YokoyamaS , ImatakaH. A human cell-derived in vitro coupled transcription/translation system optimized for production of recombinant proteins. Protein Expr. Purif.62(2), 190–198 (2008).
- Mikami S , MasutaniM , SonenbergN , YokoyamaS , ImatakaH. An efficient mammalian cell-free translation system supplemented with translation factors. Protein Expr. Purif.46(2), 348–357 (2006).
- Masutani M , MachidaK , KobayashiT , YokoyamaS , ImatakaH. Reconstitution of eukaryotic translation initiation factor 3 by co-expression of the subunits in a human cell-derived in vitro protein synthesis system. Protein Expr. Purif.87(1), 5–10 (2013).
- Machida K , ShigetaT , KobayashiA , MasumotoA , HidakaY , ImatakaH. Cell-free analysis of polyQ-dependent protein aggregation and its inhibition by chaperone proteins. J. Biotechnol.239, 1–8 (2016).
- Kobayashi T , MikamiS , YokoyamaS , ImatakaH. An improved cell-free system for picornavirus synthesis. J. Virol. Methods142(1–2), 182–188 (2007).
- Kobayashi T , NakamuraY , MikamiS , MasutaniM , MachidaK , ImatakaH. Synthesis of encephalomyocarditis virus in a cell-free system: from DNA to RNA virus in one tube. Biotechnol. Lett.34(1), 67–73 (2012).
- Imataka H , SogawaK , YasumotoKet al. Two regulatory proteins that bind to the basic transcription element (BTE), a GC box sequence in the promoter region of the rat P-4501A1 gene. EMBO J.11(10), 3663–3671 (1992).
- Machida K , KanzawaK , ShigetaT , YamamotoY , TsumotoK , ImatakaH. Huntingtin polyglutamine-dependent protein aggregation in reconstituted cells. ACS Synth. Biol.7(2), 377–383 (2018).
- Mikami S , KobayashiT , MachidaK , MasutaniM , YokoyamaS , ImatakaH. N-terminally truncated GADD34 proteins are convenient translation enhancers in a human cell-derived in vitro protein synthesis system. Biotechnol. Lett.32(7), 897–902 (2010).
- Mikami S , KobayashiT , ImatakaH. Cell-free protein synthesis systems with extracts from cultured human cells. Methods Mol. Biol.607, 43–52 (2010).
- Zhang JH , ChungTD , OldenburgKR. A simple statistical parameter for use in evaluation and validation of high throughput screening assays. J. Biomol. Screen.4(2), 67–73 (1999).
- Neupane K , MunshiS , ZhaoM , RitchieDB , IleperumaSM , WoodsideMT. Anti-frameshifting ligand active against SARS coronavirus-2 is resistant to natural mutations of the frameshift-stimulatory pseudoknot. J. Mol. Biol.432(21), 5843–5847 (2020).
- Park SJ , KimYG , ParkHJ. Identification of RNA pseudoknot-binding ligand that inhibits the -1 ribosomal frameshifting of SARS-coronavirus by structure-based virtual screening. J. Am. Chem. Soc.133(26), 10094–10100 (2011).
- Ritchie DB , SoongJ , SikkemaWK , WoodsideMT. Anti-frameshifting ligand reduces the conformational plasticity of the SARS virus pseudoknot. J. Am. Chem. Soc.136(6), 2196–2199 (2014).