Abstract
Background
Cardiovascular events are, after cancer, the most common cause of death in COPD patients. Arterial stiffness is an independent predictor of all-cause mortality and cardiovascular events. Several cross-sectional studies have confirmed increased arterial stiffness in patients with COPD. Various mechanisms in the development of arterial stiffness in COPD such as reduced lung function or systemic inflammation have been proposed. However, clinical predictors of arterial stiffness that had been reported in cross-sectional studies have not yet been confirmed in a longitudinal setting. We have assessed the course of augmentation index (AIx) - a measure of systemic arterial stiffness - and possible predictors in a cohort of COPD patients over a period of up to 7 years.
Methods
COPD patients underwent annual AIx measurement by applanation tonometry for a maximum duration of 7 years. Additionally, we performed annual assessments of lung function, blood gases, systemic inflammation, serum lipids and blood pressure. Associations between the course of AIx and potential predictors were investigated through a mixed effect model.
Results
Seventy-six patients (mean (SD) age 62.4 (7.1), male 67%) were included. The AIx showed a significant annual increase of 0.91% (95% CI 0.21/1.60) adjusted for baseline. The change in diffusion capacity (DLco), low-density lipoprotein (LDL), and high-sensitivity c-reactive protein (hsCRP) was independently associated with the increasing evolution of AIx (Coef. - 0.10, p<0.001, Coef. 1.37, p=0.003, and Coef. 0.07, p=0.033, respectively).
Conclusion
This study demonstrated a meaningful increase in arterial stiffness in COPD over time. A greater annual increase in arterial stiffness was associated with the severity of emphysema (measured by DLco), systemic inflammation, and dyslipidaemia.
Clinical Trial Registration
www.ClinicalTrials.gov, NCT1527773.
Introduction
Patients with chronic obstructive pulmonary disease (COPD) are at an increased risk for cardiovascular morbidity and mortality. Cardiovascular disease is considered to be responsible for up to 30% of the deaths in patients with COPD and represents the second highest cause of death after cancer.Citation1,Citation2 Chen et alCitation3 meta-analysed an extensive collection of evidence (29 databases) from large international studies and confirmed that the risk for cardiovascular disease (peripheral artery disease, ischemic heart disease, heart failure, cardiac dysrhythmia, diseases of pulmonary circulation) is increased by up to five times in patients with COPD. The authors pointed out the crucial need for the development of strategies to screen for and reduce cardiovascular risk in COPD patients. Global cardiovascular risk scores have been established to improve risk stratification and to predict cardiovascular mortality in COPD patients.Citation4 Since these risk scores may underestimate the cardiovascular risk, particularly in early prevention, non-invasive assessment techniques such as measurement of arterial stiffness, endothelial function, and carotid intima-media thickness have been investigated to improve early detection of vascular dysfunction before it becomes clinically overt.Citation5 Arterial stiffness assessed by pulse wave velocity (PWV) and augmentation index (AIx) is a strong predictor of cardiovascular events and all-cause mortality in the general population.Citation6 Several cross-sectional studies have shown increased arterial stiffness in COPD patients when compared to healthy controls.Citation7,Citation8 Recently, a longitudinal study of cardiovascular risk in COPD patients (ARCADE-Study) confirmed increasing PWV in COPD patients over a period of 2 years.Citation9 Various mechanisms in the development of arterial stiffness in COPD have been proposed. They include some classical risk factors for atherosclerosis such as smoking, physical inactivity, and aging. In addition, more COPD specific conditions like airway obstruction, emphysema, oxidative stress, exacerbations, systemic inflammation, and increased sympathetic tonus have been incriminated in the process of increased arterial stiffness.Citation10 Even though findings on the influence of airflow limitation and systemic inflammation on arterial stiffness have been incongruent,Citation8,Citation11,Citation12 a recent review on arterial stiffness in COPD postulated that these processes may contribute to vascular remodelling and atherosclerosis progression, possibly leading to cardiovascular events.Citation5 However, most of the studies assessing arterial stiffness in COPD are limited by their cross-sectional study-designs. Longitudinal data on the evolution of arterial stiffness and its possible predictors in COPD patients are scant. In this study, our objective was to investigate the longitudinal course of arterial stiffness measured by AIx in a well-characterized cohort of COPD patients and to evaluate possible predictors that have been described in cross-sectional studies.
Methods
Subjects
The Obstructive Pulmonary Disease Outcomes Cohort Study (TOPDOCS) was performed as a prospective, non-interventional cohort project including mild to very severe COPD patients from seven pulmonary outpatient clinics in Switzerland. Initially, this study was conducted as a cross-sectional study and was later transformed into a longitudinal study design. The patients were recruited during outpatient visits or during hospitalization for initially three annual study visits. Only patients in Zurich, Switzerland, underwent pulse-wave analysis. Patients aged between 40 and 75 years with confirmed COPD according to GOLD-guidelinesCitation13 were assessed for eligibility between October 2010 and April 2016. Patients undergoing exacerbation were included into the study with a delay of at least 6 weeks. Exclusion criteria were defined as such: suffering from physical or intellectual impairment prohibiting informed consent or compliance with the protocol.
The study was conducted in accordance with the declaration of Helsinki and all subjects gave written informed consent to participate. The Ethics Committee of the Canton of Zurich approved the study (EK-ZH-NR: 2011–0106) and the study is registered at www.ClinicalTrials.gov, NCT1527773.
Measurements
All measurements were conducted within 2 hrs on the day of the annual study visits. Patients were asked to follow their usual daily routine including their regular alcohol-, coffee- and medication intake.
Vital Signs
Blood pressure (BP) and heart rate were measured in triplicate separated by one-minute intervals. Measurements were conducted after resting in supine position for 5 mins in a quiet room of our hospital with a validated, semi-automated oscillometric device (Omron, Omron Healthcare Co, Ltd. Kyoto, Japan). The average of these three blood pressure measurements was used for further analysis.
Lung Function
All patients underwent standard pulmonary functional testing according to ATS/ERS guidelines.Citation14,Citation15 Forced vital capacity (FVC), forced expiratory volume in 1 s (FEV1), residual volume to total lung capacity (RV/TLC), and diffusing capacity of the lung for carbon monoxide (DLco) were measured. Only values measured after bronchodilation are reported.
Exacerbations
Acute exacerbations (AE) were defined as an increase in patient’s dyspnea, cough, and/or sputum that led to prescription of antibiotics and/or corticosteroids. Patients were annually asked about their number of AE and the number of AEs leading to hospitalization during the preceding year. Patient reports were compared to documents from the general practitioner, pulmonologist and hospital to receive most accurate information on AE. “Frequent exacerbators” were defined as patients with ≥2 AE per year.
Daily Physical Activity (PA) and Exercise Capacity
Daily physical activity (PA) was measured by a multisensory activity monitor (SenseWear Pro™; Bodymedia Inc., Pittsburgh, PA, USA), valid to assess PA in COPD patients.Citation16 Physical activity level (PAL) as an objective measure of PA is defined as the total energy expenditure divided by the resting metabolic rate.Citation17 The monitor was worn on the upper left arm over the triceps muscle for 7 consecutive days once a year. Data were considered valid if the armband was worn at least for 4 days with a minimum of 22.5 hrs/day. Seasonality was considered in the analysis. Exercise capacity was measured by 6-mins walk distance (6MWD).Citation18
Comorbidities and Survival
Comorbidities were evaluated every year through review of the medical history, interviews and physical examination. The International Classification of Diseases - Tenth Revision (ICD-10)Citation19 was used for classifying comorbidities. Completely resolved conditions were excluded from the report. For this sub-study, only diseases associated with increased cardiovascular risk were analyzed. Follow-up time reached either from the date of baseline visit to time of death, or from baseline visit to the final study end in summer 2016. Furthermore, to assess survival time we either called the patients, their next relatives, or the general practitioner to receive current information on survival and comorbidities in spring 2019.
Blood Analysis and Blood Gas Analysis
Measurement of high-sensitivity C-reactive protein (hsCRP), cholesterol, low-density lipoprotein (LDL), high-density lipoprotein (HDL), and triglyceride (TG) was performed from fresh blood samples.
Arterial blood gas analysis was used to measure the partial pressure of oxygen (PaO2) and carbon dioxide (PaCO2). Analysis was performed with ABL 700 series blood gas analyzer (Radiometer Copenhagen).
Pulse Wave Analysis
Subjects had to rest 10 mins in supine position before pulse wave analysis. Subsequently, radial artery pulse waveforms were recorded using the SphygmoCor System (AtCor Medical, Sydney, NSW, Australia). Approximately 10 radial pulse waves were measured to generate a corresponding central aortic pressure waveform using a validated mathematical transfer function.Citation20 As shown in , the inflection point of the aortic pressure waveform corresponds to the onset of the reflected wave returning from peripheral arteries and divides the aortic pressure wave into an early and late systolic peak. This inflection point can be determined with an algorithm. The augmentation index (AIx) quantifies the augmentation of central aortic pressure, hence representing a measure of peripheral arterial wave reflection. AIx can be calculated as the difference between the second (P2) and the first systolic peak pressure (P1) and is expressed as the percentage of central pulse pressure (PP): AIx (%) = [(P2−P1)/PP] ×100. Since AIx is influenced by heart rate,Citation21,Citation22 the index is adjusted to a heart rate of 75 bpm. SphygmoCor Px software adjusts the AIx at an inverse rate of 4.8% for each 10bpm increment. To ensure a high measurement quality, only measurements with an operator index of 70 and above were accepted.
Figure 1 Schematic presentation of the pulse wave curve measured at the A. radialis. After the foot of the pulse (T0), indicating the onset of ejection, the pressure wave rises to an initial peak where it forms a shoulder (P1). This is the peak of the primary left ventricular ejection pressure. The second shoulder (P2) represents the peak of the arterial reflection wave. The difference between P2 and P1 is called augmentation pressure (AP). The end of ejection (ED) is the point of the closure of the aortic valve and time of the end of systole. The augmentation index (AIx) is calculated as the difference between the second (P2) and first (P1) systolic peak pressure and is expressed as a percentage of the central PP: AIx (%) = [(P2−P1)/PP] ×100.
![Figure 1 Schematic presentation of the pulse wave curve measured at the A. radialis. After the foot of the pulse (T0), indicating the onset of ejection, the pressure wave rises to an initial peak where it forms a shoulder (P1). This is the peak of the primary left ventricular ejection pressure. The second shoulder (P2) represents the peak of the arterial reflection wave. The difference between P2 and P1 is called augmentation pressure (AP). The end of ejection (ED) is the point of the closure of the aortic valve and time of the end of systole. The augmentation index (AIx) is calculated as the difference between the second (P2) and first (P1) systolic peak pressure and is expressed as a percentage of the central PP: AIx (%) = [(P2−P1)/PP] ×100.](/cms/asset/0b21bc14-4831-40bc-9902-b6d0aa4eeae3/dcop_a_12198138_f0001_b.jpg)
Data Analysis and Statistics
All results are shown as mean values (standard deviation (SD)) or median (25%/75% quartiles) unless otherwise stated. Statistical analysis was performed with STATA 15.1 (StataCorp, Texas, USA).
To analyse the cross-sectional association between AIx at baseline and baseline characteristics, univariate linear regression models were used. To investigate the change of AIx over time and the associations between the change in AIx (dependent variable) and the yearly assessed possible predictors such as BP, lung function, PA, and comorbidities and their annual changes, univariate mixed effect models were used. Furthermore, the independent association between AIx and possible predictors was analysed using a multivariate mixed effect regression model involving variables that showed a univariate regression p-value of <0.05. Residual analysis of the model was performed to check the regression assumptions. A two-sided p-value of <0.05 was considered statistically significant.
Results
Study Participants
Of the 219 patients screened for eligibility in the University Hospital Zurich between 2010 and 2016, 158 patients attended the baseline visit. Data from 76 COPD patients with at least two visits and available AIx measurement were entered for this sub-study (). The mean (SD) age at baseline was 62.4 (7.1) years, 67% were male, and 21% of the COPD patients were current smokers. The detailed patient characteristics are shown in . Mean (min/max) follow-up time was 2.1 (0.53/6.84) years with annual measurements. During the follow-up period, 48 patients were analysed at the second follow-up, 27 at the third follow-up and 15 at the fourth follow-up. Through the observation period, 18 of the patients died, 20 patients dropped out due to worsening health status prohibiting physical presence, and 10 patients underwent lung transplantation. Around one-fifth of patients refused/withdrew their will to participate for the first or second follow-up visit. The baseline AIx did not differ between patients who underwent follow-up and patients who did not (p=0.395).
Table 1 Patient Characteristics
Arterial Stiffness and Possible Predictors at Baseline
At baseline, mean (SD) AIx was 27.1 (8.9) %. Male patients showed a significantly lower AIx than female patients (Coef. −5.23, p=0.015). A higher BP was associated with an elevated AIx (systolic BP: Coef. 0.14, p=0.019; diastolic BP: Coef. 0.27, p=0.09). COPD severity expressed by FEV1 % pred. and PaO2 was significantly associated with a higher AIx (Coef. −0.11, p=0.019 and −1.35, p=0.029, respectively). Although missing statistical significance, patients who showed a longer survival time tend to have a lower AIx compared to patients who died earlier (Coef. −0.09, p=0.081). Further analysed, possible predictors are shown in .
Table 2 Univariate Regression Analysis of Possible Baseline Characteristics as Predictors for Baseline Aortic Augmentation Index
Change in Arterial Stiffness Over Time
AIx showed a significant mean (95% CI) annual increase of 0.83 (0.28/1.38) %, p=0.003), even when adjusted for baseline AIx (Coef. (95% CI) 0.91 (0.21/1.60) %, p=0.011) (). The annual increase of AIx was independent of the aging of the cohort over time (Coef (95% CI) 0.74 (0.14/1.34), p=0.015).
Table 3 Univariate Mixed Effect Model of Annual Change in AIx
Potential Predictors of Arterial Stiffness Increase Over Time
In the univariate mixed effect model, the amount of annual increase of AIx was positively associated with the change of RV/TLC (Coef. 0.05, p=0.034) and the change of DLco (Coef −0.06, p=0.036) (). On the other hand, changes in FEV1% pred. and blood gases had no effect on the course of AIx. High LDL (Coef. 0.61, p=0.038) and the intake of statins (Coef. 1.49, p=0.039) were positively associated with an increasing AIx over time. In addition, high hsCRP levels, but not the annual change in hsCRP, were positively associated with the AIx course (Coef. 0.07, p=0.017) (). A history of coronary artery disease revealed a significant positive association with the annual increase of AIx (Coef. 2.04, p=0.041). In contrast, the course of exacerbations, smoking status, inhalation therapy, and blood pressure did not show any association with the annual increment of AIx. All results are presented in and . The multivariate effect model confirmed an independent, positive association of LDL (Coef. 1.37, p=0.003) and hsCRP (Coef. 0.07, p=0.033) with an increased evolution of AIx (). In addition, the AIx course was independently and negatively associated with the annual change in DLco (Coef −0.10, p<0.001). A shorter survival time was not associated with a greater increase in AIx over time. Due to the high correlation between LDL and statin intake, as well as between DLco and RV/TLC, statin intake and RV/TCL were removed from the final multivariate model.
Table 4 Univariate Mixed Effect Model of Possible Predictors for Annual Change in AIx, Adjusted for AIx at Baseline
Table 5 Univariate Mixed Effect Model of Annual Change in Possible Predictors for Annual Change in AIx, Adjusted for AIx at Baseline
Table 6 Multivariate Mixed Effect Model of Annual Change in AIx, Adjusted for AIx at Baseline
Figure 3 The figure shows the estimation of the course of AIx over time according to the influence of change in DLco % pred based on the unadjusted regression model. The mean (CI) AIx at baseline (0) and follow-up visits (1–4) is estimated for a yearly change in DLco % pred. of −40, −20, 0, and 10.
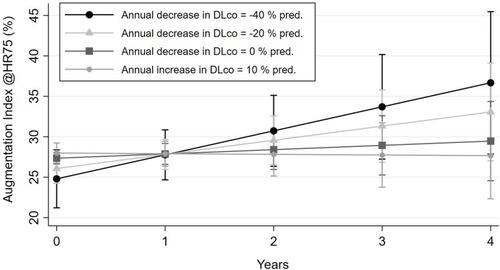
Figure 4 The figure demonstrates the estimated course of AIx over time dependent on hsCRP values based on the unadjusted regression model. The mean (CI) AIx at baseline (0) and yearly follow-up (1–4) is estimated for hsCRP values of 5, 20, 50 and 70 mg/l.
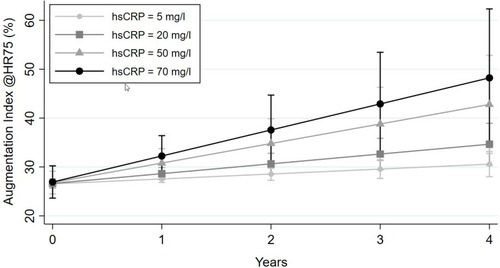
Discussion
This study investigated the longitudinal course of arterial stiffness and its possible predictors in a well-characterized cohort of patients with mild to very severe COPD. We found an annual increase of AIx indicating progressive rigidity of the arterial wall in COPD patients over time. Decreasing DLco, a surrogate marker of emphysema, as well as increasing systemic inflammation and dyslipidaemia enhanced the increasing evolution of arterial stiffness over time. Our results add novel information to the field of cardiovascular risk in patients with COPD. By assessing potential predictors on the course of arterial stiffness, these data provide additional information useful for predicting the cardiovascular disease course of COPD patients in clinical practice.
The mean (SD) AIx (27.1 ± 8.9%) in our cohort was comparable to former studies assessing arterial stiffness in COPD patients with similar age, gender ratio and BMI.Citation23 To our knowledge, there are no longitudinal data available describing the course of arterial stiffness measured by AIx, neither in COPD patients nor in healthy individuals. Nevertheless, compared to the suspected annual AIx course in the general population obtained from cross-sectional, unselected population-based studies, our study revealed an elevated annual AIx increase in COPD patients (0.26–0.38%/year vs 0.91%/year).Citation24 Furthermore, the increase in AIx was independent of aging in our cohort over time. The recently published ARCADE study assessed arterial stiffness measured by PWV in COPD patients and comparators (current or past smokers, FEV/FVC>70%) over 2 years. They found similar increase in the rates of PWV in both groups. However, this study was limited by differences in smoking status, overt cardiovascular disease, and dropout rates between the two groups. In addition, arterial stiffness measured by AIx and PWV are not directly comparable or interchangeable.
Possible mechanisms leading to increased arterial stiffness like aging, elevated BP, dyslipidaemia, and chronic inflammation have been extensively reviewed in general cardiovascular literature.Citation25–Citation27 In COPD, arterial stiffness has been independently associated with impairment of lung function, hypoxia, systemic inflammation, and increased sympathetic tone; factors that could contribute to the increased cardiovascular risk in COPD patients.Citation8,Citation11 Further, the severity of emphysema measured by computed tomography scans has been described to be independently associated with arterial stiffness in COPD patients in cross-sectional studies.Citation11,Citation28,Citation29 Our data support the idea of a pathomechanical link between the severity of emphysema and increased arterial stiffness by showing an independently positive association between change in DLco and the course of AIx in COPD patients. Our study does not provide annual CT-scans to describe the course of emphysema. The main reason was the protection of the study participants from unnecessary radiation. Nevertheless, DLco is a surrogate marker of emphysema in COPD is shown to represent the severity of tissue destruction in the process of emphysema development.Citation30,Citation31 Although the exact pathophysiological mechanisms are not yet completely understood, recent findings suggest that increased systemic elastin degradation by matrix metalloproteinases like MMP-9 may lead to both formation of emphysema and arterial stiffness, depending on the grade of genetic predisposition.Citation11,Citation32 Maclay et alCitation32 found increased skin elastin degradation in COPD patients when compared to age and smoking matched healthy controls. Noteworthy, the upregulated skin elastolysis was associated with the grade of emphysema and arterial stiffness. In addition, the inhibition of matrix metalloproteinases in animal models reduced vascular calcium deposition; a process that reflects the vascular elastin degradation.Citation33 Smoke exposure plays a key role in the proteolytic attack against lung tissue, inter alia, through upregulating MMP in the setting of smoke-induced inflammation.Citation34 Regarding the increase of arterial stiffness over time, it seems to be crucial to prevent the progression of emphysema in COPD patients in clinical practice.
Several cross-sectional studies reported an independent relationship between the severity of airflow limitation measured as FEV1 and arterial stiffness in COPD.Citation11,Citation23,Citation35 Sabit et alCitation8 found an inversed relationship between FEV1 and arterial stiffness in 75 clinically stable COPD patients. Even though we could reproduce this observation at baseline, the course of arterial stiffness was not predicted by FEV1 or the annual change in FEV1 in the longitudinal setting. The same observation was made for PaO2. Cinarka et alCitation36 conducted a prospective, observational study reporting increased arterial stiffness in patients with severe COPD and hypoxic states. In our study, even if low PaO2 was negatively associated with AIx at baseline, the significance of this observation got lost looking at the course of AIx over time. Interestingly, these two conditions could have played a central role in the pathomechanical connection between emphysema and arterial stiffness. A possible explanation could be found in the different study designs. The cross-sectional nature of the studies mentioned above could not deliver determinants, indicating the direction and time-dependent relationships of obstructive lung function assessments and arterial stiffness. In addition, cross-sectional associations are at higher risk to be influenced by unknown confounders.
Arterial stiffness has been suggested as one pathophysiologic element linking chronic systemic inflammation and increased cardiovascular risk.Citation37 Several human and animal-based studies have shed light on this complex process on a molecular pathophysiological level. CRP, interleukine-6 (IL-6) and endothelin-1 production can alter NO synthesis, which, in turn, could lead to endothelial dysfunction. In addition, increased IL-6 and TNF-α could increase the expression of adhesion molecules on activated endothelial cells and facilitate the formation of atheromatous plaques.Citation38,Citation39 In this study, hsCRP was independently associated with the evolution of arterial stiffness over time supporting the hypothesis that systemic inflammation could be an important determinant in the development of increased arterial stiffness and further cardiovascular risk in COPD patients. Although dyslipidaemia is a well-known, independent risk factor for cardiovascular disease, the relationship between serum lipids and arterial stiffness is still under debate. Wang et alCitation40 conducted a population-based, cross-sectional study with 2375 participants investigating the relationship between serum lipids and PWV. They found LDL to be independently positive and HDL independently inversely associated with arterial stiffness. Our data confirmed these findings in a longitudinal setting, even though the association between HDL and AIx course did not quite reach statistical significance. The intake of statins showed a high correlation to elevated LDL values and was therefore not analysed in our final statistical model. Nevertheless, these findings underline the crucial need to check for dyslipidaemia to initiate aggressive lipid-lowering therapy in COPD patients. Several previous studies determined arterial stiffness not only as an independent predictor of cardiovascular events but also of all-cause mortality.Citation6 In this study, shorter survival time was not associated with a steeper rise of AIx over time. Nevertheless, the negative association between survival time and AIx at baseline with barely missed statistical significance (p=0.081) could suggest that the period of longitudinal observation might be too short to show an association between survival time and course of AIx. Some classical risk factors like BP, smoking status, and PA showed no association to the course of arterial stiffness, even though these conditions had been described as significantly influencing arterial stiffness. However, the postulated link between these factors and arterial stiffness in COPD is driven by cross-sectional data. Mills et alCitation41 assessed arterial stiffness in 102 COPD patients and 103 healthy controls matched for age and smoking status. The cross-sectional analysis revealed increased arterial stiffness in COPD patients which was positively associated with diastolic and systolic BP. We could confirm this association through analysing arterial stiffness and BP cross-sectional at baseline. Nevertheless, no statistically significant association was detectable between BP, its changes, and the course of AIx in the longitudinal analysis, thus leading to the conclusion that elevated BP might only play a minor role in the development of arterial stiffness in COPD. In contrast to former cross-sectional studies,Citation7 increased PA or exercise capacity had no impact on the increase of AIx over time.
Limitations
There are some limitations to our study. A recent review about aging and arterial stiffness pointed out, that changes of arterial stiffness measured by AIx are more prominent in younger patients, whereas the changes in aortic PWV are more marked in patients older than 50 years.Citation25 As a result, the increasing arterial stiffness over time might still be underestimated in our cohort. Furthermore, since several patients with very severe COPD were unable to follow our invitations due to worsening health status, the influence of airflow limitation on the course of arterial stiffness could be underestimated; despite the AIx at baseline being no different. In addition, the protocol did not provide the formation and investigation of a control group. Therefore, we could not directly compare the natural increase in AIx with the course of AIx in COPD patients. Nonetheless, little information is available on patterns and determinants of longitudinal change in arterial stiffness in COPD.
Conclusion
To our knowledge, this is the first longitudinal study illustrating the course of arterial stiffness in COPD measured by AIx over time. Our data clearly demonstrated an annual increase of arterial stiffness in patients with COPD, which was independently associated with the course of CO diffusion capacity, serum lipids, and inflammation. Further studies are needed to elucidate the complex interactions between these factors in order to understand their clinical implication.
Abbreviations
AE, acute exacerbation; Aix, Augmentation index; BMI, body mass index; CI, confidence interval; Coef., coefficient; COPD, chronic obstructive pulmonary disease; hsCRP, high-sensitivity c-reactive protein; DLco, diffusing capacity of the lung for carbon monoxide; GOLD, global initiative for chronic obstructive lung disease; FEV1, forced expiratory volume in 1 s; FVC, forced vital capacity; HDL, high-density lipoprotein; HR, hazard ratio; IQR, interquartile range; LDL, low-density lipoprotein; MMP, matrix metalloproteinases; mMRC, modified British Medical Research Council; PA, physical activity; PAL, physical activity level; PWV, pulse-wave velocity; RV/TLC, residual volume to total lung capacity; SD, standard deviation; TG, triglyceride; TOPDOCS, The Obstructive Pulmonary Disease Outcomes Cohort Study; 6MWD, 6-mins walk distance.
Data Sharing Statement
The datasets used and/or analysed during the current study are available from the corresponding author on reasonable request.
Author Contributions
All authors made substantial contributions to conception and design, acquisition of data, or analysis and interpretation of data; took part in drafting the article or revising it critically for important intellectual content; gave final approval of the version to be published; and agree to be accountable for all aspects of the work.
Disclosure
Prof. Dr. Malcolm Kohler reports grants and personal fees from Bayer, personal fees from Novartis, personal fees from Boehringer, personal fees from GSK, personal fees from Astra Zeneca, grants from Roche, personal fees from CSL Behring, and personal fees from Mundipharma, during the conduct of the study. The authors report no other conflicts of interest in this work.
Acknowledgment
This study was supported by Lunge Zurich.
References
- BerryCE, WiseRA. Mortality in COPD: causes, risk factors, and prevention. COPD. 2010;7:375–382. doi:10.3109/15412555.2010.51016020854053
- StoneIS, BarnesNC, PetersenSE. Chronic obstructive pulmonary disease: a modifiable risk factor for cardiovascular disease? Heart. 2012;98:1055–1062.22739636
- ChenW, ThomasJ, SadatsafaviM, et al. Risk of cardiovascular comorbidity in patients with chronic obstructive pulmonary disease: a systematic review and meta-analysis. Lancet Respir Med. 2015;3:631–639. doi:10.1016/S2213-2600(15)00241-626208998
- LeeHM, LeeJ, LeeK, et al. Relation between COPD severity and global cardiovascular risk in US adults. Chest. 2012;142:1118–1125. doi:10.1378/chest.11-242122518027
- VivodtzevI, TamisierR, BaguetJP, et al. Arterial stiffness in COPD. Chest. 2014;145:861–875. doi:10.1378/chest.13-180924687708
- VlachopoulosC, AznaouridisK, StefanadisC. Prediction of cardiovascular events and all-cause mortality with arterial stiffness: a systematic review and meta-analysis. J Am Coll Cardiol. 2010;55:1318–1327. doi:10.1016/j.jacc.2009.10.06120338492
- SieviNA, FranzenD, KohlerM, et al. Physical inactivity and arterial stiffness in COPD. Int J Chron Obstruct Pulmon Dis. 2015;10:1891–1897. doi:10.2147/COPD.S9094326392763
- SabitR, BoltonCE, EdwardsPH, et al. Arterial stiffness and osteoporosis in chronic obstructive pulmonary disease. Am J Respir Crit Care Med. 2007;175:1259–1265. doi:10.1164/rccm.200701-067OC17363772
- GaleNS, AlbarratiAM, MunneryMM, et al. Aortic pulse wave velocity as a measure of cardiovascular risk in chronic obstructive pulmonary disease: two-year follow-up data from the ARCADE study. Medicina (Kaunas). 2019;55:89. doi:10.3390/medicina55040089
- SarkarMJER. Chronic obstructive pulmonary disease and arterial stiffness. EMJ Respir. 2016;4:114–121.
- McAllisterDA, MaclayJD, MillsNL, et al. Arterial stiffness is independently associated with emphysema severity in patients with chronic obstructive pulmonary disease. Am J Respir Crit Care Med. 2007;176:1208–1214. doi:10.1164/rccm.200707-1080OC17885263
- VanfleterenLE, SpruitMA, GroenenMT, et al. Arterial stiffness in patients with COPD: the role of systemic inflammation and the effects of pulmonary rehabilitation. Eur Respir J. 2014;43:1306–1315. doi:10.1183/09031936.0016931324311762
- RabeKF, HurdS, AnzuetoA, et al. Global strategy for the diagnosis, management, and prevention of chronic obstructive pulmonary disease - GOLD executive summary. Am J Respir Crit Care Med. 2007;176:532–555. doi:10.1164/rccm.200703-456SO17507545
- MillerMR, HankinsonJ, BrusascoV, et al. Standardisation of spirometry. Eur Respir J. 2005;26:319–338. doi:10.1183/09031936.05.0003480516055882
- MacintyreN, CrapoRO, ViegiG, et al. Standardisation of the single-breath determination of carbon monoxide uptake in the lung. Eur Respir J. 2005;26:720–735. doi:10.1183/09031936.05.0003490516204605
- Van RemoortelH, RasteY, LouvarisZ, et al. Validity of six activity monitors in chronic obstructive pulmonary disease: a comparison with indirect calorimetry. PLoS One. 2012;7:e39198. doi:10.1371/journal.pone.003919822745715
- WatzH, WaschkiB, MeyerT, et al. Physical activity in patients with COPD. Eur Respir J. 2009;33:262. doi:10.1183/09031936.0002460819010994
- HollandAE, SpruitMA, TroostersT, et al. An official European Respiratory Society/American Thoracic Society technical standard: field walking tests in chronic respiratory disease. Eur Respir J. 2014;44:1428–1446. doi:10.1183/09031936.0015031425359355
- WHO. International Classification of Diseases (ICD). 2013 Available from: http://www.who.int/classifications/icd/en/. Accessed 921, 2013.
- Pauca AlfredoL, O’Rourke MichaelF, Kon NealD. Prospective evaluation of a method for estimating ascending aortic pressure from the radial artery pressure waveform. Hypertension. 2001;38:932–937. doi:10.1161/hy1001.09610611641312
- WilkinsonIB, MacCallumH, FlintL, et al. The influence of heart rate on augmentation index and central arterial pressure in humans. J Physiol. 2000;525(Pt 1):263–270. doi:10.1111/j.1469-7793.2000.t01-1-00263.x10811742
- WilkinsonIB, MohammadNH, TyrrellS, et al. Heart rate dependency of pulse pressure amplification and arterial stiffness. Am J Hypertens. 2002;15:24–30. doi:10.1016/S0895-7061(01)02252-X11824855
- AlbuA, FodorD, BondorC, et al. Carotid arterial stiffness in patients with chronic obstructive pulmonary disease. Acta Physiol Hung. 2011;98:117–127. doi:10.1556/APhysiol.98.2011.2.321616770
- JannerJH, GodtfredsenNS, LadelundS, et al. The association between aortic augmentation index and cardiovascular risk factors in a large unselected population. J Hum Hypertens. 2012;26:476–484. doi:10.1038/jhh.2011.5921654851
- LeeH-Y, OhB-H. Aging and arterial stiffness. Circ J. 2010;74:2257–2262. doi:10.1253/circj.CJ-10-091020962429
- WuC-F, LiuP-Y, WuT-J, et al. Therapeutic modification of arterial stiffness: an update and comprehensive review. World J Cardiol. 2015;7:742–753. doi:10.4330/wjc.v7.i11.74226635922
- ZiemanSJ, MelenovskyV, KassDA. Mechanisms, pathophysiology, and therapy of arterial stiffness. Arterioscler Thromb Vasc Biol. 2005;25:932–943. doi:10.1161/01.ATV.0000160548.78317.2915731494
- BarrRG, AhmedFS, CarrJJ, et al. Subclinical atherosclerosis, airflow obstruction and emphysema: the MESA lung study. Eur Respir J. 2012;39:846–854. doi:10.1183/09031936.0016541022034646
- DuckersJM, ShaleDJ, StockleyRA, et al. Cardiovascular and musculoskeletal co-morbidities in patients with alpha 1 antitrypsin deficiency. Respir Res. 2010;11:173. doi:10.1186/1465-9921-11-17321138571
- GouldGA, RedpathAT, RyanM, et al. Lung CT density correlates with measurements of airflow limitation and the diffusing capacity. Eur Respir J. 1991;4:141–146.2044729
- ScharfSM, IqbalM, KellerC, et al. Hemodynamic characterization of patients with severe emphysema. Am J Respir Crit Care Med. 2002;166:314–322. doi:10.1164/rccm.210702712153963
- MaclayJD, McAllisterDA, RabinovichR, et al. Systemic elastin degradation in chronic obstructive pulmonary disease. Thorax. 2012;67:606–612. doi:10.1136/thoraxjnl-2011-20094922374923
- QinX, CorriereMA, MatrisianLM, et al. Matrix metalloproteinase inhibition attenuates aortic calcification. Arterioscler Thromb Vasc Biol. 2006;26:1510–1516. doi:10.1161/01.ATV.0000225807.76419.a716690876
- ChurgA, ZhouS, WrightJL. Matrix metalloproteinases in COPD. Eur Res J . 2012;39:197–209. doi:10.1183/09031936.00121611
- ZureikM, BenetosA, NeukirchC, et al. Reduced pulmonary function is associated with central arterial stiffness in men. Am J Respir Crit Care Med. 2001;164:2181–2185. doi:10.1164/ajrccm.164.12.210713711751184
- CinarkaH, KayhanS, GumusA, et al. Arterial stiffness measured via carotid femoral pulse wave velocity is associated with disease severity in COPD. Respir Care. 2014;59:274–280. doi:10.4187/respcare.0262123821765
- DransfieldMT, CockcroftJR, TownsendRR, et al. Effect of fluticasone propionate/salmeterol on arterial stiffness in patients with COPD. Respir Med. 2011;105:1322–1330. doi:10.1016/j.rmed.2011.05.01621696934
- Cortez-CooperM, MeadersE, StallingsJ, et al. Soluble TNF and IL-6 receptors: indicators of vascular health in women without cardiovascular disease. Vasc Med. 2013;18:282–289. doi:10.1177/1358863X1350833624165468
- HungMJ, CherngWJ, HungMY, et al. Interleukin-6 inhibits endothelial nitric oxide synthase activation and increases endothelial nitric oxide synthase binding to stabilized caveolin-1 in human vascular endothelial cells. J Hypertens. 2010;28:940–951. doi:10.1097/HJH.0b013e32833992ef20375905
- WangF, YeP, LuoL, et al. Association of serum lipids with arterial stiffness in a population-based study in Beijing. Eur J Clin Invest. 2011;41:929–936. doi:10.1111/eci.2011.41.issue-921314825
- MillsNL, MillerJJ, AnandA, et al. Increased arterial stiffness in patients with chronic obstructive pulmonary disease: a mechanism for increased cardiovascular risk. Thorax. 2008;63:306. doi:10.1136/thx.2007.08349318024535